Solvent Compatibility: The Ultimate Guide You Need Now!
Solvent compatibility, a crucial factor in fields ranging from pharmaceutical development to coating applications, profoundly impacts product performance. Hildebrand Solubility Parameters, a widely-used metric, estimate the miscibility of different solvents, aiding formulators in predicting blend behavior. The Environmental Protection Agency (EPA) emphasizes understanding solvent compatibility to minimize environmental impact from industrial processes. Furthermore, optimal solvent blends, guided by principles of solvent compatibility, can improve the efficacy of chromatography techniques in analytical laboratories. Mastering solvent compatibility principles allows you to ensure quality and effectiveness in every step of your process.
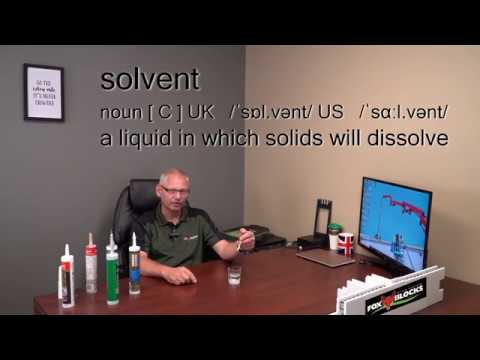
Image taken from the YouTube channel Fox Blocks ICF , from the video titled Fox Blocks Compatibility with solvent based products .
Solvent compatibility is the cornerstone of successful formulations and processes across a multitude of industries. It refers to the ability of two or more solvents to mix together and form a stable, homogeneous solution.
In simpler terms, it's about ensuring that the solvents get along well and don't separate or cause undesirable effects.
Why Solvent Compatibility Matters
Understanding solvent compatibility is paramount because it directly impacts product performance, stability, and even safety.
A well-chosen solvent blend can optimize the properties of a coating, ensure the strength of an adhesive, or improve the efficacy of a pharmaceutical product. Conversely, incompatible solvents can lead to a host of problems.
Industries Reliant on Solvent Compatibility
Solvent compatibility isn't just a theoretical concept; it's a practical necessity in numerous fields.
-
Coatings: Achieving the desired gloss, smoothness, and drying time depends heavily on selecting compatible solvent blends.
-
Adhesives: Solvent compatibility ensures proper wetting of the surfaces being bonded, leading to strong and durable joints.
-
Pharmaceuticals: Solvents play a crucial role in drug formulation and delivery, and their compatibility affects drug solubility, stability, and bioavailability.
-
Inks: Proper solvent mixtures ensure the ink flows correctly, dries at the right rate, and adheres well to the substrate.
-
Cleaning Products: Compatibility helps create effective cleaning solutions that dissolve dirt and grime without damaging the surfaces being cleaned.
Hansen Solubility Parameters (HSP): A Predictive Tool
One of the most valuable tools for predicting solvent compatibility is the concept of Hansen Solubility Parameters (HSP).
HSP provides a numerical way to characterize solvents based on their dispersion forces, polar forces, and hydrogen bonding capabilities. By comparing the HSP values of different solvents, it's possible to estimate whether they will be compatible. We will delve deeper into HSP later on.
The Consequences of Solvent Incompatibility
Failing to consider solvent compatibility can have significant consequences, leading to:
-
Phase Separation: The mixture separates into distinct layers, resulting in a non-uniform product.
-
Cloudiness or Haziness: The solution becomes translucent or opaque, affecting its appearance and potentially its performance.
-
Material Degradation: Incompatible solvents can cause swelling, cracking, or dissolution of materials, compromising their structural integrity.
-
Changes in Viscosity: The viscosity of the mixture can increase or decrease unexpectedly, affecting its flow properties and application.
-
Reduced Product Performance: Coatings may exhibit poor adhesion, adhesives may lose strength, and pharmaceutical formulations may become unstable.
Hansen Solubility Parameters offer a robust, quantifiable way to navigate the complexities of solvent interactions. However, to truly leverage this predictive power, a firm grasp of solvent classifications is essential. Understanding the fundamental differences between solvent types allows for informed decisions when selecting and blending solvents for specific applications.
Key Solvents: Polar vs. Non-Polar, Protic vs. Aprotic
Solvents are categorized based on several key characteristics, most notably their polarity and proticity. These properties dictate how a solvent interacts with other substances, influencing its ability to dissolve them and its overall compatibility with other solvents.
Polar vs. Non-Polar Solvents
The distinction between polar and non-polar solvents hinges on the distribution of electrical charge within the solvent molecule. This charge distribution dictates the types of intermolecular forces a solvent can exert, and consequently, what types of substances it can dissolve.
Molecular Structure and Intermolecular Forces
Polar solvents possess molecules with an uneven distribution of electron density, creating partial positive and negative charges. This asymmetry arises from differences in electronegativity between the atoms within the molecule.
The resulting dipole moment allows polar solvents to engage in dipole-dipole interactions and, in some cases, hydrogen bonding. These stronger intermolecular forces enable polar solvents to effectively dissolve other polar substances, as well as ionic compounds.
Non-polar solvents, on the other hand, have a relatively even distribution of electron density. Their molecules lack a significant dipole moment, and their intermolecular forces are primarily London dispersion forces.
These forces are weaker than dipole-dipole interactions or hydrogen bonds, limiting non-polar solvents to dissolving other non-polar substances through induced dipole interactions.
Examples of Polar and Non-Polar Solvents
Common examples of polar solvents include:
- Water (H₂O).
- Alcohols (e.g., methanol, ethanol, isopropanol).
- Acetone.
- Acetonitrile.
- Dimethylformamide (DMF).
- Dimethyl Sulfoxide (DMSO).
These solvents are frequently used in applications where dissolving polar solutes is necessary, such as in pharmaceuticals, cosmetics, and certain types of coatings.
Examples of non-polar solvents include:
- Hexane.
- Toluene.
- Xylene.
- Diethyl ether.
- Petroleum ether.
These solvents are commonly used in applications such as degreasing, dissolving oils and fats, and in certain types of paints and varnishes.
Protic vs. Aprotic Solvents
Beyond polarity, solvents are also classified as either protic or aprotic, based on their ability to donate protons (H⁺). This ability has significant implications for chemical reactions and solvent compatibility.
Proton Donation and its Consequences
Protic solvents contain hydrogen atoms bonded to highly electronegative atoms, such as oxygen or nitrogen. This arrangement makes these hydrogen atoms acidic and capable of being donated as protons.
This ability to donate protons influences the solvent's behavior in chemical reactions. For instance, protic solvents can stabilize anions through hydrogen bonding, affecting reaction rates and mechanisms.
Aprotic solvents, conversely, lack acidic protons. While they may contain hydrogen atoms, these atoms are not readily donated as protons.
This inability to donate protons alters their interaction with solutes and their influence on chemical reactions. Aprotic solvents are often preferred in reactions where proton donation is undesirable, such as in certain types of nucleophilic substitutions.
Examples of Protic and Aprotic Solvents
Common examples of protic solvents include:
- Water (H₂O).
- Alcohols (e.g., methanol, ethanol, isopropanol).
- Acetic acid.
- Formic acid.
These solvents are widely used in acid-base chemistry and in reactions where proton transfer is involved.
Examples of aprotic solvents include:
- Acetone.
- Acetonitrile.
- Dimethylformamide (DMF).
- Dimethyl Sulfoxide (DMSO).
- Dichloromethane (DCM).
These solvents are commonly used in reactions requiring a non-acidic environment and in applications where stabilizing cations is important.
Influence of Polarity and Proticity on Solvent Compatibility
Polarity and proticity play crucial roles in determining solvent compatibility. The "like dissolves like" principle dictates that polar solvents tend to be miscible with other polar solvents, while non-polar solvents tend to be miscible with other non-polar solvents.
Similarly, proticity can influence miscibility, with protic solvents often exhibiting better compatibility with other protic solvents. However, exceptions exist, and understanding the interplay between polarity, proticity, and the specific chemical structures of the solvents is crucial for accurate predictions. Hansen Solubility Parameters provide a more nuanced and quantitative approach to predicting these complex interactions.
These intermolecular forces are weaker than dipole-dipole interactions or hydrogen bonds, limiting their ability to dissolve polar substances. The preference of non-polar solvents is primarily for dissolving other non-polar compounds.
The Science of Solubility: How Solvents Interact
The dance between solvents and solutes, the very act of dissolution, is governed by a set of fundamental principles. Understanding these principles is not just academic; it’s the key to predicting solvent behavior and designing effective solutions for a myriad of applications.
The Guiding Principle: "Like Dissolves Like"
The cornerstone of solvent compatibility is the adage "like dissolves like." This seemingly simple statement encapsulates a complex interplay of intermolecular forces. Essentially, it means that solvents tend to dissolve solutes that share similar chemical characteristics and intermolecular forces.
Polar solvents are adept at dissolving polar solutes and ionic compounds, while non-polar solvents favor non-polar substances. This affinity arises from the energetic favorability of interactions between similar molecules.
When the intermolecular forces between solvent and solute are comparable to those within each substance individually, dissolution is thermodynamically favored. If, however, there is a significant mismatch, the solute will likely remain undissolved, or even worse, phase separation may occur.
Decoding Solubility and Miscibility
While often used interchangeably, solubility and miscibility have distinct meanings. Solubility refers to the ability of a solid, liquid, or gas to dissolve in a liquid solvent, forming a homogeneous solution. It is typically expressed as the maximum amount of solute that can dissolve in a given amount of solvent at a specific temperature.
Miscibility, on the other hand, specifically describes the ability of two liquids to mix and form a homogeneous solution. If two liquids are miscible, they can be mixed in any proportion without phase separation.
Immiscible liquids, such as oil and water, will separate into distinct layers due to their differing polarities and intermolecular forces.
Factors Influencing Solubility
Several factors can influence the extent to which a solute dissolves in a solvent. The most important of these include temperature, pressure, and molecular structure.
Temperature
Temperature plays a crucial role in solubility. For most solid solutes in liquid solvents, solubility increases with increasing temperature. This is because higher temperatures provide more energy to break the intermolecular forces holding the solute together, facilitating its interaction with the solvent.
However, there are exceptions to this rule. The solubility of some salts can decrease with increasing temperature. For gases, the opposite trend is observed: solubility generally decreases as temperature increases.
Pressure
Pressure has a significant effect on the solubility of gases in liquids. Henry's Law states that the solubility of a gas in a liquid is directly proportional to the partial pressure of the gas above the liquid.
In simpler terms, increasing the pressure of a gas above a liquid forces more gas molecules to dissolve in the liquid. This principle is utilized in carbonated beverages, where carbon dioxide is dissolved under pressure.
Molecular Structure
The molecular structure of both the solvent and the solute profoundly impacts solubility. As discussed earlier, the principle of "like dissolves like" highlights the importance of matching polarity and intermolecular forces.
Solutes with structures that allow them to form strong intermolecular interactions with the solvent will exhibit higher solubility. For example, alcohols, with their ability to form hydrogen bonds, are generally more soluble in water than hydrocarbons of similar molecular weight.
The Role of Intermolecular Forces
Intermolecular forces are the attractive or repulsive forces that exist between molecules. These forces dictate the physical properties of substances, including their melting point, boiling point, and, crucially, their solubility.
Hydrogen Bonding
Hydrogen bonding is a particularly strong type of intermolecular force that occurs between molecules containing hydrogen atoms bonded to highly electronegative atoms such as oxygen, nitrogen, or fluorine. Solvents capable of hydrogen bonding, like water and alcohols, are excellent solvents for other hydrogen-bonding compounds.
Van der Waals Forces
Van der Waals forces encompass a range of weaker intermolecular forces, including dipole-dipole interactions, dipole-induced dipole interactions, and London dispersion forces. London dispersion forces are present in all molecules, regardless of polarity, and arise from temporary fluctuations in electron distribution.
The strength of London dispersion forces increases with the size and surface area of the molecule. These forces are particularly important in non-polar solvents, where they are the primary means of intermolecular interaction.
The intricate interplay of these factors dictates whether a substance will gracefully dissolve or stubbornly resist. But can we move beyond simple observations and actually predict solubility? The answer lies in a powerful tool called Hansen Solubility Parameters.
Hansen Solubility Parameters (HSP): A Powerful Prediction Tool
Hansen Solubility Parameters (HSP) represent a significant advancement in predicting solvent compatibility. Instead of relying solely on the "like dissolves like" rule, HSP provides a quantitative framework for assessing the interactions between solvents and solutes. This allows for a more precise and informed selection of solvents for various applications.
Understanding the Significance of HSP
HSP, developed by Charles M. Hansen, offers a three-dimensional approach to characterizing solvents and polymers based on their intermolecular forces. It moves beyond a simple binary classification of polar and non-polar.
The power of HSP lies in its ability to quantify the relative contributions of different types of intermolecular forces to the overall solubility behavior. This allows for a more nuanced understanding of solvent-solute interactions and more accurate predictions of compatibility.
The Three HSP Parameters
At the heart of the HSP system are three parameters, each representing a different type of intermolecular force: dispersion forces (δD), polar forces (δP), and hydrogen bonding forces (δH).
Dispersion (δD)
The dispersion parameter (δD) accounts for the London dispersion forces, also known as van der Waals forces. These forces arise from temporary fluctuations in electron distribution, creating temporary dipoles.
Dispersion forces are present in all molecules, regardless of polarity, and are generally the dominant force in non-polar substances. Higher δD values indicate stronger dispersion interactions.
Polar (δP)
The polar parameter (δP) quantifies the contribution of dipole-dipole interactions between molecules. These interactions occur in polar molecules due to uneven electron distribution and permanent dipoles.
Substances with high δP values exhibit strong dipole-dipole interactions and tend to be more soluble in other polar solvents.
Hydrogen Bonding (δH)
The hydrogen bonding parameter (δH) measures the strength of hydrogen bonds formed between molecules. Hydrogen bonds are a particularly strong type of dipole-dipole interaction that occurs when hydrogen is bonded to highly electronegative atoms like oxygen, nitrogen, or fluorine.
Solvents with high δH values are capable of forming strong hydrogen bonds and are often good solvents for other hydrogen bonding compounds. Water is a classic example of a solvent with a high δH value.
Predicting Solvent Compatibility with HSP
HSP values can be used to predict solvent compatibility by calculating the distance (Ra) between the HSP values of a solvent and a solute (or another solvent). This distance represents the degree of dissimilarity between the intermolecular forces of the two substances.
The closer the HSP values are, the smaller the distance (Ra), and the more likely the two substances are to be compatible (i.e., soluble or miscible). A smaller Ra suggests a higher degree of "like dissolves like."
Calculating the Distance (Ra)
The distance (Ra) between two sets of HSP values is calculated using the following equation:
Ra = √[4(δD1 - δD2)² + (δP1 - δP2)² + (δH1 - δH2)²]
Where:
- δD1, δP1, δH1 are the dispersion, polar, and hydrogen bonding parameters for substance 1.
- δD2, δP2, δH2 are the dispersion, polar, and hydrogen bonding parameters for substance 2.
The factor of 4 applied to the dispersion parameter is an empirical correction factor that has been found to improve the accuracy of predictions.
The Solubility Sphere
A "solubility sphere" can be visualized in HSP space, with the center representing the HSP values of the solute and the radius (Ro) representing the maximum distance (Ra) at which solvents will still dissolve the solute. Solvents falling within the sphere are considered good solvents, while those outside are considered poor solvents.
Examples of HSP Calculations and Interpretation
Let's consider a simplified example: predicting the solubility of a polymer in different solvents. Suppose a polymer has the following HSP values: δD = 18 MPa0.5, δP = 8 MPa0.5, δH = 10 MPa0.5.
We want to determine whether acetone (δD = 15.5 MPa0.5, δP = 10.4 MPa0.5, δH = 7 MPa0.5) and hexane (δD = 14.9 MPa0.5, δP = 0 MPa0.5, δH = 0 MPa0.5) are good solvents for this polymer.
-
Calculate Ra for Acetone:
Ra = √[4(18 - 15.5)² + (8 - 10.4)² + (10 - 7)²] = √(25 + 5.76 + 9) = √39.76 ≈ 6.31 MPa0.5
-
Calculate Ra for Hexane:
Ra = √[4(18 - 14.9)² + (8 - 0)² + (10 - 0)²] = √(38.44 + 64 + 100) = √202.44 ≈ 14.23 MPa0.5
Assuming the radius of the solubility sphere (Ro) for the polymer is 7 MPa0.5, acetone (Ra = 6.31 MPa0.5) falls within the sphere and is predicted to be a good solvent.
Hexane (Ra = 14.23 MPa0.5) falls outside the sphere and is predicted to be a poor solvent. This analysis aligns with the general understanding that acetone, a polar solvent, is more likely to dissolve a polymer with moderate polarity and hydrogen bonding capability than hexane, a non-polar solvent.
By utilizing Hansen Solubility Parameters and performing these calculations, scientists and engineers can make informed decisions about solvent selection, leading to more effective formulations, improved product performance, and reduced trial-and-error experimentation.
The power of HSP lies in its ability to quantify the relative contributions of different types of intermolecular forces to the overall solubility behavior. This allows for a more nuanced understanding of solvent-solute interactions and more accurate predictions of compatibility. Now, let's shift our focus to how these theoretical concepts translate into real-world applications. Understanding solvent compatibility isn't just an academic exercise; it's a critical factor in ensuring the success and longevity of products across various industries.
Compatibility in Action: Coatings, Adhesives, and Plastics
Solvent compatibility plays a pivotal role in determining the performance and durability of various products. The selection of appropriate solvents is particularly crucial in industries such as coatings, adhesives, and plastics, where the wrong choice can lead to a multitude of problems, from aesthetic flaws to structural failures. Let's examine how solvent compatibility manifests in these key areas.
Solvent Compatibility in Coatings
Coatings rely heavily on solvents to dissolve or disperse the binder (resin) and other additives, ensuring a smooth and uniform application. The solvent's compatibility with the binder is paramount.
Solvent Selection for Different Coating Types
The choice of solvent varies depending on the type of coating.
-
Acrylic coatings often utilize solvents like toluene, xylene, or esters due to their good solvency and evaporation rates.
-
Epoxy coatings, known for their durability and chemical resistance, may require stronger solvents such as ketones or glycol ethers to effectively dissolve the epoxy resin.
-
Water-based coatings present a unique scenario, where co-solvents (small amounts of organic solvents) might be added to aid film formation and improve freeze-thaw stability.
Consequences of Incompatible Solvents in Coatings
Using incompatible solvents in coatings can lead to a range of defects, impacting the final product's appearance and performance.
-
Blushing, a milky or hazy appearance, occurs when moisture condenses in the coating film during evaporation due to rapid cooling caused by the solvent's evaporation. This is often due to the solvent's inability to adequately dissolve the resin.
-
Orange peel describes a textured surface resembling the skin of an orange, which arises from poor leveling and flow of the coating. It is generally caused by a solvent system that evaporates too quickly or has poor solvency for the binder.
-
Cratering, the formation of small, crater-like depressions in the film, results from surface tension differences and the inability of the coating to flow properly, which are often exacerbated by incompatible solvents.
Solvent Compatibility in Adhesives
Adhesives rely on solvents to carry the adhesive polymer and facilitate wetting of the substrates to be bonded. Solvent selection directly impacts the adhesive's ability to form a strong and durable bond.
Solvent Selection for Adhesive Formulations
Different adhesive types require different solvent systems:
-
Contact adhesives frequently utilize solvents like acetone or methyl ethyl ketone (MEK) for rapid evaporation and tack development.
-
Epoxy adhesives may use solvents such as xylene or toluene to adjust viscosity and improve wetting properties.
-
Cyanoacrylate adhesives (super glues) typically do not contain solvents, but surface preparation with a compatible solvent cleaner is often essential for optimal bonding.
The Impact of Incompatibility on Adhesion
Solvent incompatibility can compromise the adhesive's ability to create a lasting bond.
-
Reduced adhesion strength can occur if the solvent doesn't properly dissolve or disperse the adhesive polymer, leading to poor wetting of the substrate.
-
Decreased durability may result from a solvent that weakens the adhesive bond over time, making it susceptible to environmental factors or mechanical stress.
-
Bond failure can happen if the solvent causes phase separation or other forms of instability in the adhesive formulation.
Solvent Compatibility in Plastics
Plastics exhibit varying degrees of resistance to different solvents. Understanding solvent compatibility is crucial for preventing damage, degradation, and failure in plastic components.
Solvent Resistance of Different Plastics
The chemical structure of a plastic determines its solvent resistance.
-
Polyethylene (PE) and polypropylene (PP), being non-polar polymers, generally exhibit good resistance to polar solvents but can swell or dissolve in non-polar solvents like hexane or toluene.
-
Polyvinyl chloride (PVC) is more resistant to aliphatic hydrocarbons but can be attacked by ketones, esters, and aromatic solvents.
-
Acrylics are generally dissolved by ketones, esters, and chlorinated solvents.
Solvent-Induced Damage to Plastics
Solvents can interact with plastics in several ways.
-
Swelling occurs when solvent molecules penetrate the plastic matrix, causing it to expand and soften. This can alter the plastic's dimensions and mechanical properties.
-
Dissolution is the complete breaking down of the plastic structure as solvent molecules disrupt the intermolecular forces holding the polymer chains together.
-
Environmental Stress Cracking (ESC) involves the combined action of stress and a solvent, leading to crack formation and premature failure.
By carefully considering solvent compatibility in coatings, adhesives, and plastics, manufacturers can optimize product performance, enhance durability, and avoid costly failures. A proactive approach to solvent selection based on understanding of HSP is essential for success in these diverse applications.
Solvent compatibility profoundly influences material selection and performance, but responsible solvent handling requires an unwavering focus on safety. Understanding the potential hazards and implementing appropriate safety measures is paramount to protecting yourself and your environment. Let's delve into the crucial aspects of using Safety Data Sheets (SDS) and Personal Protective Equipment (PPE), cornerstones of safe solvent handling.
Ensuring Safety: SDS and PPE
Safety isn't just a box to check; it's a deeply ingrained practice when working with solvents. Every solvent possesses unique properties that dictate its potential hazards. Ignoring these hazards can lead to serious health consequences, environmental damage, and even catastrophic accidents. Understanding how to access and interpret crucial safety information is your first line of defense.
The Importance of Safety Data Sheets (SDS)
Safety Data Sheets (SDS) are comprehensive documents that provide detailed information about a chemical substance. Think of them as essential guides containing everything you need to know to handle a specific solvent safely.
Navigating Solvent Hazards, Flammability, and Toxicity
The SDS is your primary source for identifying the specific hazards associated with a particular solvent.
- Solvent Hazards: Look for hazard statements that describe the potential health effects of exposure (e.g., skin irritation, respiratory problems, organ damage).
- Flammability: Check the flash point, which indicates the lowest temperature at which a solvent's vapors can ignite. Also, note the flammability classification (e.g., flammable, highly flammable).
- Toxicity: Review the toxicity data (e.g., LD50, LC50) to understand the potential for acute and chronic health effects from exposure.
Understanding the SDS Sections
An SDS is organized into a standardized format with 16 sections, making it easier to find the information you need. Some key sections include:
- Identification: Identifies the substance and supplier.
- Hazard(s) Identification: Lists all hazards associated with the substance.
- Composition/Information on Ingredients: Details the chemical composition.
- First-Aid Measures: Provides instructions for immediate medical attention.
- Fire-Fighting Measures: Outlines appropriate fire-fighting techniques and equipment.
- Accidental Release Measures: Describes procedures for containing and cleaning up spills.
- Handling and Storage: Offers guidance on safe handling and storage practices.
- Exposure Controls/Personal Protection: Specifies exposure limits and recommended PPE.
- Physical and Chemical Properties: Lists physical and chemical characteristics.
- Stability and Reactivity: Describes the substance's stability and potential reactivity.
- Toxicological Information: Provides detailed toxicological data.
- Ecological Information: Addresses environmental impact.
- Disposal Considerations: Offers guidance on safe disposal methods.
- Transport Information: Details transportation requirements.
- Regulatory Information: Lists applicable regulations.
- Other Information: Includes additional relevant information.
Carefully review each section relevant to your work to understand the risks involved and how to mitigate them.
Personal Protective Equipment (PPE) for Solvent Handling
PPE acts as a barrier between you and hazardous solvents, minimizing exposure and reducing the risk of injury or illness. The correct PPE is crucial for mitigating the risks identified in the SDS.
Gloves: The First Line of Defense
Gloves are essential for protecting your skin from direct contact with solvents. However, not all gloves are created equal. The type of glove you need depends on the solvent you're using.
- Nitrile gloves are generally suitable for a wide range of solvents.
- Neoprene gloves offer good protection against many solvents, oils, and acids.
- Butyl rubber gloves provide excellent resistance to ketones, esters, and alcohols.
- PVC gloves are often used for handling aqueous solutions and some acids.
Always check the glove manufacturer's chemical resistance chart to ensure the gloves you choose are compatible with the specific solvent you're using.
Eye Protection: Shielding Your Vision
Eye protection is critical for preventing solvent splashes or vapor exposure from damaging your eyes.
- Safety glasses provide basic protection against splashes and impacts.
- Goggles offer a tighter seal around the eyes, providing better protection against vapors and splashes.
- Face shields provide full-face protection and are recommended when handling large volumes of solvents or when there's a high risk of splashes.
Choose eye protection that meets ANSI Z87.1 standards for impact resistance and chemical splash protection.
Respiratory Protection: Breathing Safely
Solvent vapors can be harmful or even deadly if inhaled. Respiratory protection is necessary when working with solvents in poorly ventilated areas or when the concentration of vapors exceeds permissible exposure limits.
- Respirators filter out airborne contaminants, protecting your lungs.
- Air-purifying respirators (APRs) use cartridges or filters to remove specific contaminants.
- Supplied-air respirators (SARs) provide a supply of clean air from an external source.
A proper fit test is essential to ensure the respirator provides adequate protection. Consult with a safety professional to determine the appropriate type of respirator for your specific needs.
The Importance of Proper Ventilation
Adequate ventilation is a critical component of solvent safety. Ventilation helps to remove solvent vapors from the air, reducing the risk of inhalation and preventing the build-up of flammable concentrations.
- Natural ventilation (opening windows and doors) can be sufficient for some applications.
- Mechanical ventilation (using fans or exhaust systems) is necessary for most solvent-handling operations.
- Local exhaust ventilation (LEV) systems capture vapors at the source, preventing them from spreading into the work area.
Regularly inspect and maintain ventilation systems to ensure they are functioning properly.
By diligently utilizing SDSs, selecting appropriate PPE, and ensuring adequate ventilation, you can significantly reduce the risks associated with solvent handling and create a safer working environment. Safety is a continuous process that requires vigilance and a commitment to best practices.
Ensuring Safety: SDS and PPE arms you with the knowledge to handle solvents responsibly. However, understanding the potential for solvents to interact with and potentially degrade the materials they contact is equally critical for long-term performance and reliability. This is where the concept of chemical resistance comes into play, influencing material selection and preventative measures.
Chemical Resistance: Preventing Degradation and Failure
Chemical resistance is a material's ability to withstand exposure to chemicals, including solvents, without undergoing significant degradation or alteration of its properties. It dictates a material's suitability for use in environments where it will come into contact with specific solvents.
The Significance of Chemical Resistance
In material selection, chemical resistance is paramount. Choosing materials with poor chemical resistance can lead to premature failure of components and products. This results in costly repairs, replacements, and potentially hazardous situations.
Conversely, selecting chemically resistant materials ensures longevity, reliability, and safety in various applications. This includes storage containers, pipelines, protective coatings, and even everyday consumer products.
Mechanisms of Solvent-Induced Degradation
Solvents can degrade materials through various mechanisms. Understanding these mechanisms is crucial for predicting and preventing material failure.
Swelling
Swelling occurs when a solvent penetrates the material's structure, causing it to expand in volume. This can lead to dimensional changes, loss of mechanical strength, and eventual cracking. Polymers are particularly susceptible to swelling.
Dissolution
Dissolution is the complete dissolving of a material in a solvent. This is most common with polymers and other organic materials. Dissolution results in a complete loss of the material's structural integrity.
Cracking
Certain solvents can induce cracking in materials, especially under stress. This phenomenon, known as environmental stress cracking (ESC), can lead to sudden and catastrophic failure.
Other Degradation Mechanisms
Other forms of degradation include:
- Discoloration: Change in the material's color due to chemical reactions.
- Embrittlement: Loss of flexibility, making the material prone to fracture.
- Surface crazing: Formation of fine cracks on the surface.
Selecting Compatible Solvents: A Proactive Approach
Preventing material degradation begins with selecting solvents that are compatible with the materials they will contact. Several strategies can be employed to achieve this.
Understanding Material Properties
Familiarize yourself with the chemical resistance properties of different materials. Information about a material's resistance to specific solvents can be found in material datasheets and technical specifications.
Considering Solvent Properties
Assess the properties of the solvent, including its polarity, proticity, and chemical reactivity. As a general rule, “like dissolves like.” Polar solvents tend to dissolve polar materials, while non-polar solvents dissolve non-polar materials.
Performing Compatibility Testing
Conduct small-scale compatibility tests to evaluate the interaction between a solvent and a material. This involves exposing the material to the solvent under relevant conditions and monitoring for signs of degradation.
Material Compatibility Charts: Your Quick Reference Guide
Material compatibility charts are valuable resources that provide guidance on the compatibility of different materials with various solvents. These charts typically rate compatibility using terms such as:
- Excellent: Material is highly resistant to the solvent.
- Good: Material is resistant to the solvent under certain conditions.
- Fair: Material may exhibit some degradation with prolonged exposure.
- Poor: Material is not resistant to the solvent and will likely degrade rapidly.
Limitations of Compatibility Charts
While helpful, compatibility charts should be used as a general guide. Actual compatibility can vary depending on factors such as:
- Temperature
- Concentration
- Exposure Time
- Stress Levels
Therefore, it's always recommended to supplement compatibility charts with your own testing under specific application conditions.
Ensuring Safety: SDS and PPE arms you with the knowledge to handle solvents responsibly. However, understanding the potential for solvents to interact with and potentially degrade the materials they contact is equally critical for long-term performance and reliability. This is where the concept of chemical resistance comes into play, influencing material selection and preventative measures.
Regulatory Compliance: Navigating the Legal Landscape of Solvent Use
Working with solvents isn't just about understanding their chemical properties; it's also about adhering to a complex web of regulations designed to protect human health and the environment. Ignoring these regulations can lead to significant legal and financial repercussions.
Understanding and complying with these regulations is therefore not optional; it’s a fundamental aspect of responsible solvent management. This section will unpack some key regulatory frameworks that govern solvent use, focusing on REACH (in Europe) and OSHA (in the United States).
The Importance of Regulatory Compliance
Regulatory compliance is paramount when handling solvents. It ensures the safety of workers, minimizes environmental impact, and avoids costly penalties. These regulations are not arbitrary; they are based on scientific evidence and risk assessments aimed at mitigating the inherent hazards associated with solvent use.
Moreover, demonstrating compliance enhances a company's reputation and builds trust with customers and stakeholders. It signals a commitment to responsible business practices and environmental stewardship.
REACH: Managing Chemicals in the European Union
REACH, which stands for Registration, Evaluation, Authorisation and Restriction of Chemicals, is a comprehensive European Union regulation that governs the production and use of chemical substances. It places the burden of proof on companies to demonstrate that their chemicals are safe for human health and the environment.
Registration Requirements
One of the core tenets of REACH is the requirement for companies manufacturing or importing chemicals into the EU to register these substances with the European Chemicals Agency (ECHA).
This registration process involves submitting detailed information about the chemical's properties, uses, and potential hazards. ECHA then evaluates this information to assess the risks associated with the chemical and determine whether further action is needed.
Restrictions on Solvent Use
REACH also includes provisions for restricting the use of certain solvents deemed to pose unacceptable risks. These restrictions can range from complete bans to limitations on specific applications.
For example, certain chlorinated solvents, known for their toxicity and environmental persistence, are subject to strict controls under REACH. It’s crucial to stay informed about these restrictions, as they can change over time based on evolving scientific understanding and risk assessments.
OSHA: Protecting Workers in the United States
In the United States, the Occupational Safety and Health Administration (OSHA) is responsible for ensuring safe and healthful working conditions. OSHA has established a range of regulations that apply to solvent use in the workplace.
Permissible Exposure Limits (PELs)
OSHA sets permissible exposure limits (PELs) for many common solvents. These PELs represent the maximum concentration of a solvent that workers can be exposed to over an eight-hour workday.
PELs are designed to protect workers from the acute and chronic health effects associated with solvent exposure. Employers are required to monitor workplace air quality to ensure that PELs are not exceeded and implement engineering controls and personal protective equipment (PPE) to minimize worker exposure.
Hazard Communication and Worker Training
OSHA's Hazard Communication Standard requires employers to provide workers with information about the hazards of the chemicals they work with, including solvents. This information is typically conveyed through Safety Data Sheets (SDS) and worker training programs.
Training must cover topics such as the proper handling and storage of solvents, the use of PPE, and emergency procedures. Effective hazard communication and training are essential for empowering workers to protect themselves from solvent-related hazards.
Staying Up-to-Date with Regulatory Changes
The regulatory landscape surrounding solvent use is constantly evolving. New scientific information, technological advancements, and societal concerns can all lead to changes in regulations.
It is therefore essential to stay informed about the latest regulatory developments. This can involve subscribing to industry newsletters, participating in professional organizations, and regularly reviewing the websites of regulatory agencies like ECHA and OSHA. Proactive monitoring of regulatory changes is crucial for maintaining compliance and ensuring a safe and environmentally responsible workplace.
Video: Solvent Compatibility: The Ultimate Guide You Need Now!
Frequently Asked Questions About Solvent Compatibility
Here are some frequently asked questions to help clarify aspects of solvent compatibility, ensuring you choose the right solvents for your applications.
What does "solvent compatibility" actually mean?
Solvent compatibility refers to the ability of two or more solvents to mix together and form a stable, homogenous solution. Incompatible solvents, when mixed, may separate into distinct layers, cloud, or precipitate. Understanding this is crucial for successful chemical processes.
Why is solvent compatibility important?
Choosing compatible solvents prevents unwanted phase separation, ensures consistent reaction conditions, and avoids damage to equipment. Proper solvent compatibility is essential for efficient extractions, clean reactions, and reliable product formulations.
How do I determine if two solvents are compatible?
You can assess solvent compatibility using resources like solvent miscibility charts or software that predicts compatibility based on solubility parameters. Experimental testing, such as mixing the solvents in different ratios and observing for cloudiness or separation, is also a reliable method.
What happens if I mix incompatible solvents?
Mixing incompatible solvents can lead to a variety of problems. These issues range from decreased reaction efficiency and product yield to potential equipment damage due to precipitation or unwanted chemical reactions. In some instances, it can even create hazardous conditions.