Potassium's Oxidation Number: Explained Simply! (60 Char)
The octet rule in chemistry establishes a foundation for understanding how elements achieve stability. Potassium (K), an alkali metal, tends to lose one electron to achieve this stable state, influencing its characteristic potassium oxidation number. Specifically, the concept of electronegativity, as quantified by the Pauling scale, helps explain why potassium readily gives up its electron. Understanding how these properties interact is crucial for various applications, including predicting the behavior of potassium salts in chemical reactions.
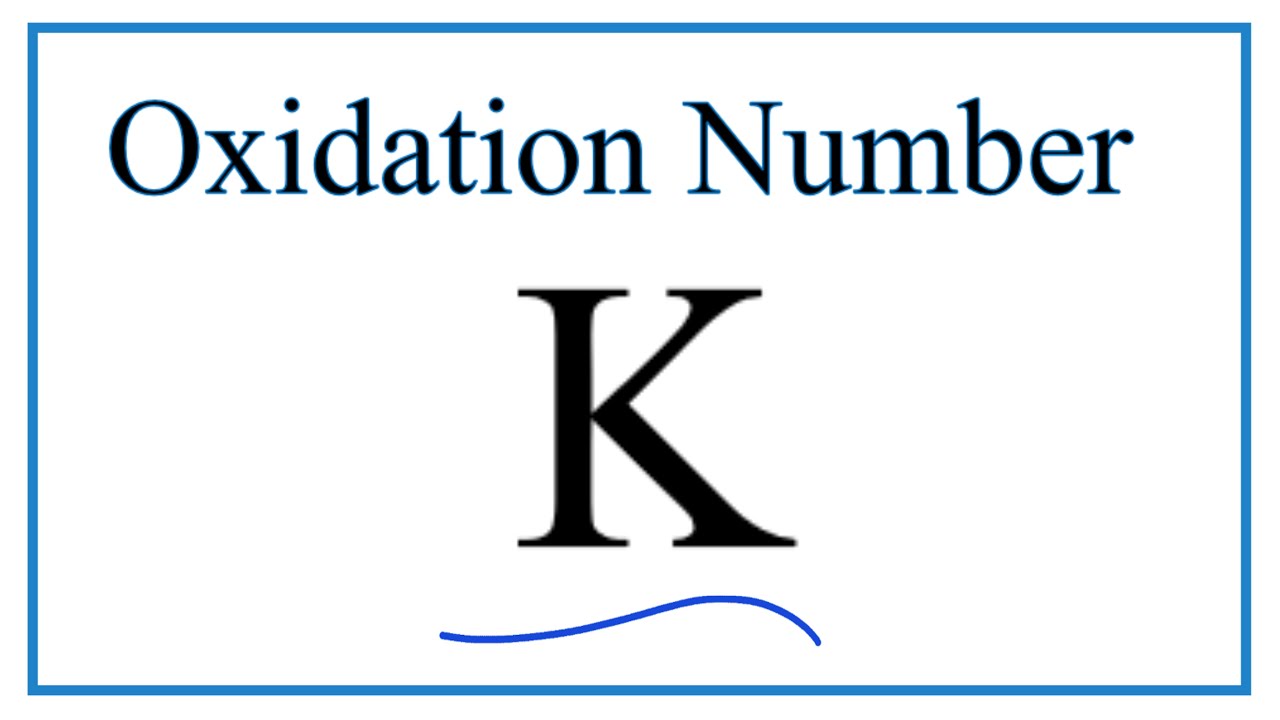
Image taken from the YouTube channel Wayne Breslyn (Dr. B.) , from the video titled How to find the Oxidation Number for Potassium (K) .
Potassium (K), a silvery-white metal, holds a pivotal position in the world of chemistry and beyond. Its reactivity and unique properties make it essential to numerous biological processes, industrial applications, and chemical reactions. From maintaining electrolyte balance in the human body to acting as a crucial component in fertilizers, potassium's influence is undeniable.
This article aims to provide a clear and accessible explanation of potassium's oxidation number, a fundamental concept for understanding its chemical behavior. We will delve into the underlying principles that govern this value, offering insights into why potassium consistently exhibits a specific oxidation state in most of its compounds.
Why Understanding Oxidation Numbers Matters
Oxidation numbers, also known as oxidation states, represent the hypothetical charge an atom would have if all bonds were completely ionic. While not actual charges in covalent compounds, they provide a powerful tool for predicting and explaining chemical reactivity.
By understanding oxidation numbers, chemists can:
-
Predict the outcome of chemical reactions: Knowing the oxidation states of reactants helps determine the products formed.
-
Balance chemical equations: Oxidation numbers are crucial for ensuring mass and charge conservation in redox reactions.
-
Understand electron transfer: Oxidation numbers directly relate to the gain or loss of electrons during chemical processes.
-
Classify chemical compounds: They provide insights into the nature of bonding and the overall chemical behavior of substances.
In essence, mastering oxidation numbers unlocks a deeper understanding of the chemical world, enabling one to decipher the intricate dance of electrons that governs interactions between atoms and molecules. Specifically, understanding the rules and consistent nature of potassium's oxidation number serves as a valuable entry point into this broader chemical understanding.
Oxidation numbers are not just abstract concepts; they are the foundation upon which we build our understanding of chemical reactions and the behavior of elements within compounds. They allow us to predict how atoms will interact and what products will form.
Demystifying Oxidation Number: The Basics
To fully grasp potassium's predictable behavior, it's crucial to first understand the fundamental concept of oxidation number. What exactly is an oxidation number, and how do we determine it?
Defining Oxidation Number/Oxidation State
The oxidation number, also known as the oxidation state, represents the hypothetical charge an atom would have if all bonds were perfectly ionic. This means we're assuming that electrons are completely transferred from one atom to another, even when the bond is actually covalent (where electrons are shared).
It's important to remember that oxidation numbers are a bookkeeping method.
They don't necessarily reflect the actual charge on an atom in a molecule or ion.
However, they provide invaluable information about the distribution of electrons and the potential for an atom to participate in chemical reactions.
Oxidation numbers are typically written with a plus or minus sign before the number (e.g., +1, -2), which distinguishes them from ionic charges (e.g., 1+, 2-).
General Rules for Assigning Oxidation Numbers
Assigning oxidation numbers follows a set of rules. These rules prioritize electronegativity differences and allow us to systematically determine the oxidation state of each atom in a compound or ion.
While a comprehensive list exists, we will focus on the rules most relevant for understanding potassium:
-
Rule 1: Elemental Form: The oxidation number of an atom in its elemental form is always 0. For example, potassium metal (K), solid sulfur (S8), and diatomic oxygen (O2) all have oxidation numbers of 0. This is because there is no electronegativity difference between atoms of the same element.
-
Rule 2: Neutral Compounds: The sum of the oxidation numbers of all atoms in a neutral compound must equal 0. This reflects the overall electrical neutrality of the compound. For example, in sodium chloride (NaCl), the oxidation number of sodium (Na) is +1, and the oxidation number of chlorine (Cl) is -1. The sum (+1 + -1 = 0) confirms the compound's neutrality.
-
Rule 3: Polyatomic Ions: The sum of the oxidation numbers of all atoms in a polyatomic ion must equal the charge of the ion.
For example, in the sulfate ion (SO42-), the sum of the oxidation numbers of sulfur and oxygen must equal -2. This rule is vital for understanding the oxidation states of elements in complex ions.
The Role of Electronegativity
Oxidation numbers are assigned based on electronegativity differences. Electronegativity is a measure of an atom's ability to attract electrons in a chemical bond. The more electronegative atom in a bond is assigned a negative oxidation number, as if it has gained electrons. The less electronegative atom is assigned a positive oxidation number, as if it has lost electrons.
Understanding electronegativity is crucial for predicting the oxidation states of elements. It’s the driving force behind electron distribution. The greater the electronegativity difference, the more "ionic" the bond is considered for oxidation number assignment purposes.
Oxidation numbers are not just abstract concepts; they are the foundation upon which we build our understanding of chemical reactions and the behavior of elements within compounds. They allow us to predict how atoms will interact and what products will form.
Now that we’ve established the fundamental principles of oxidation numbers, let's delve into what makes potassium so predictable. Its position on the periodic table, specifically its membership in the alkali metal family, holds the key to understanding its consistent +1 oxidation state.
Potassium's Place: The Alkali Metal Family
Potassium's behavior isn't arbitrary; it's intrinsically linked to its location within the periodic table. Understanding the characteristics of the alkali metals sheds light on why potassium so readily adopts a +1 oxidation number.
Introducing the Alkali Metals
The alkali metals, found in Group 1 (IA) of the periodic table, are a family of elements renowned for their high reactivity. This group includes lithium (Li), sodium (Na), potassium (K), rubidium (Rb), cesium (Cs), and francium (Fr).
Several key characteristics define them:
-
They are all shiny, silvery-white metals.
-
They are highly reactive, readily reacting with water and air.
-
They are soft and can be cut with a knife.
-
They are excellent conductors of heat and electricity.
Their reactivity stems from their electronic structure. Each alkali metal possesses only one valence electron – a single electron in its outermost shell.
Potassium: A Group 1 Element
Potassium (K) resides in the fourth period of Group 1. This placement dictates its properties and behavior.
As an alkali metal, potassium shares the group's characteristic high reactivity.
It's a soft, silvery-white metal that rapidly tarnishes in air.
It reacts vigorously with water, generating heat and hydrogen gas.
Potassium's electron configuration explains its tendencies. It readily loses one electron to achieve a stable, noble gas configuration.
The +1 Connection: Losing an Electron
The defining trait of Group 1 elements is their tendency to lose one electron. This is the direct cause of their consistent +1 oxidation number.
When an alkali metal loses its single valence electron, it forms a positively charged ion with a +1 charge. This electron loss is energetically favorable because it results in a full outer electron shell.
This stable configuration mirrors that of the nearest noble gas.
The resulting +1 charge directly corresponds to the element's oxidation number.
Therefore, potassium, like other alkali metals, almost always exhibits a +1 oxidation number in its compounds. This predictable behavior simplifies the analysis of chemical reactions involving potassium.
Potassium's behavior isn't arbitrary; it's intrinsically linked to its location within the periodic table. Understanding the characteristics of the alkali metals sheds light on why potassium so readily adopts a +1 oxidation number.
Losing Electrons: Potassium's Ion Formation
The consistent +1 oxidation number of potassium is intimately linked to its proclivity for losing a single electron. This process, known as ionization, transforms a neutral potassium atom into a positively charged potassium ion (K+). Understanding this transformation is crucial to grasping the predictability of potassium in chemical reactions.
The Role of Valence Electrons
At the heart of potassium's reactivity lies its electronic configuration. Specifically, potassium possesses one valence electron. This lone electron resides in its outermost electron shell.
This single valence electron is held relatively loosely compared to the electrons closer to the nucleus. This is because it is shielded from the full positive charge of the nucleus by the inner electron shells.
The Formation of the K+ Ion
Potassium readily donates its single valence electron to achieve a more stable electron configuration. By losing this electron, potassium attains the same electron configuration as the noble gas argon (Ar). Noble gases are known for their exceptional stability due to their full outer electron shells.
This process of electron loss results in the formation of the potassium ion, symbolized as K+. The resulting ion carries a positive charge because it now has one more proton (positive charge) than electrons (negative charge).
Oxidation Number and Ionic Charge
The direct consequence of potassium's ionization is the creation of a positively charged ion. This brings us full circle to the concept of oxidation number.
The +1 charge on the K+ ion directly corresponds to potassium's +1 oxidation number. This positive value signifies that the potassium atom has lost one electron. This fundamentally illustrates the relationship between electron transfer and the assignment of oxidation numbers.
Potassium's behavior isn't arbitrary; it's intrinsically linked to its location within the periodic table. Understanding the characteristics of the alkali metals sheds light on why potassium so readily adopts a +1 oxidation number.
The consistent +1 oxidation number of potassium is intimately linked to its proclivity for losing a single electron. This process, known as ionization, transforms a neutral potassium atom into a positively charged potassium ion (K+). Understanding this transformation is crucial to grasping the predictability of potassium in chemical reactions.
At the heart of potassium's reactivity lies its electronic configuration. Specifically, potassium possesses one valence electron. This lone electron resides in its outermost electron shell. This single valence electron is held relatively loosely compared to the electrons closer to the nucleus. This is because it is shielded from the full positive charge of the nucleus by the inner electron shells.
Potassium readily donates its single valence electron to achieve a more stable electron configuration. By losing this electron, potassium attains the same electron configuration as the noble gas argon (Ar). Noble gases are known for their exceptional stability due to their full outer electron shells.
This process of electron loss results in the formation of the potassium ion, symbolized as K+. The resulting ion carries a positive charge because it now has one more proton (positive charge) than electrons (negative charge). The direct consequence of potassium's ionization sets the stage for the discussion of electronegativity.
Electronegativity's Influence on Potassium
Electronegativity plays a pivotal role in dictating how elements interact and form chemical bonds. It's a fundamental concept for understanding potassium's characteristic +1 oxidation state. Considering electronegativity reveals why potassium so readily relinquishes its valence electron.
Defining Electronegativity
Electronegativity quantifies an atom's ability to attract shared electrons in a chemical bond. Introduced by Linus Pauling, it's a relative measure.
Higher electronegativity values indicate a stronger pull on electrons.
The Pauling scale, the most common scale, assigns values ranging from approximately 0.7 to 4.0. Fluorine, the most electronegative element, has a value of 3.98.
Electronegativity differences between bonding atoms determine the type of bond formed. Large differences lead to ionic bonds, while small differences result in covalent bonds.
Potassium's Low Electronegativity and Electron Loss
Potassium's electronegativity is relatively low, around 0.82 on the Pauling scale. This low value indicates a weak attraction for electrons in a chemical bond.
Consequently, potassium readily loses its valence electron when interacting with more electronegative elements. This electron loss drives the formation of the K+ ion and the +1 oxidation state.
The ease with which potassium loses its electron is a direct consequence of this low electronegativity. The nucleus has a weaker hold.
Electronegativity Differences in Potassium Compounds
When potassium bonds with other elements, the electronegativity difference is typically significant. Oxygen, chlorine, and fluorine, for example, are considerably more electronegative than potassium.
Consider potassium chloride (KCl). Chlorine (electronegativity = 3.16) is far more electronegative than potassium. This difference leads to chlorine "stealing" potassium's valence electron.
In potassium oxide (K2O), oxygen (electronegativity = 3.44) exerts a stronger pull on electrons, resulting in the same effect. These electronegativity differences drive the formation of ionic bonds and stabilize the K+ ion.
The consistent pattern in potassium compounds reveals that elements bonding with potassium are almost always more electronegative. This confirms potassium's predisposition for electron loss and its unwavering +1 oxidation state.
Electronegativity dictates potassium's behavior at the atomic level, but to understand how this translates into real-world compounds, we must consider the role of chemical bonding. The way potassium interacts with other elements to form molecules and ionic structures directly influences its observed oxidation number.
Bonding Basics: Potassium's Role in Compounds
Chemical bonds are the glue that holds atoms together, forming the molecules and extended structures that make up the world around us. To understand potassium's consistent +1 oxidation number in compounds, it's crucial to understand the fundamental types of chemical bonds: ionic and covalent.
A Quick Review of Chemical Bonding
Ionic bonds arise from the complete transfer of electrons from one atom to another. This creates positively charged ions (cations) and negatively charged ions (anions), which are then held together by electrostatic attraction.
Covalent bonds, on the other hand, involve the sharing of electrons between atoms. This sharing allows atoms to achieve a more stable electron configuration.
Potassium's Predilection for Ionic Bonds
Potassium's behavior in bonding is dictated by its electronic structure and relatively low electronegativity. With its single valence electron, potassium readily gives it up to achieve a stable noble gas configuration.
Because of its low electronegativity, potassium doesn't hold onto its valence electron very strongly. It is also not very good at attracting electrons from other atoms.
This makes it much more likely to lose its valence electron to a more electronegative atom than to share electrons in a covalent bond.
This results in the formation of a K+ ion and an ionic bond. This strong tendency to form ionic bonds is key to understanding its consistent +1 oxidation state.
The +1 Rule in Ionic Compounds
In most ionic compounds, potassium exhibits a +1 oxidation number. This is because it almost always loses one electron to form the K+ ion.
For example, in potassium chloride (KCl), potassium donates its electron to chlorine, forming K+ and Cl- ions. The resulting electrostatic attraction between these oppositely charged ions creates a stable ionic compound.
The same principle applies to many other potassium compounds, such as potassium oxide (K2O) and potassium bromide (KBr). In each case, potassium loses one electron and acquires a +1 oxidation number.
It's important to remember that while potassium primarily forms ionic bonds and exhibits a +1 oxidation number, exceptions may occur in very specific and unusual circumstances. These exceptions are beyond the scope of introductory chemistry. However, in the vast majority of compounds encountered, assuming a +1 oxidation number for potassium is a safe and reliable approach.
Bonding dictates how potassium behaves in stable compounds, but what happens when potassium is actively changing its oxidation state? To understand this, we need to explore the world of redox reactions.
Potassium in Action: Redox Reactions Explained
Redox reactions, short for reduction-oxidation reactions, are fundamental processes in chemistry that involve the transfer of electrons between chemical species.
These reactions are not just theoretical concepts; they are the driving force behind countless natural phenomena and industrial applications, from the rusting of iron to the generation of electricity in batteries.
Understanding Oxidation and Reduction
At the heart of every redox reaction lies two complementary processes: oxidation and reduction.
Oxidation is the loss of electrons by a species, resulting in an increase in its oxidation number.
Reduction, conversely, is the gain of electrons by a species, leading to a decrease in its oxidation number.
It's crucial to remember that oxidation and reduction always occur simultaneously. One species cannot be oxidized without another being reduced, and vice versa. This is why they are collectively termed "redox" reactions.
Potassium as a Reducing Agent
Potassium, with its strong tendency to lose its single valence electron, is an excellent reducing agent. A reducing agent is a substance that donates electrons to another species, causing the reduction of that species and, in the process, becoming oxidized itself.
In essence, potassium readily gives up its electron to form the K+ ion, increasing its own oxidation number from 0 (in its elemental form) to +1. This electron transfer facilitates the reduction of another species in the reaction.
A Simplified Redox Example: Potassium and Water
A classic example of a redox reaction involving potassium is its reaction with water:
2K(s) + 2H₂O(l) → 2KOH(aq) + H₂(g)
In this reaction, elemental potassium (K) reacts vigorously with liquid water (H₂O) to produce potassium hydroxide (KOH) and hydrogen gas (H₂).
Let's break down the oxidation states:
- Potassium (K) starts with an oxidation state of 0.
- It then becomes part of potassium hydroxide (KOH), where it has an oxidation state of +1.
- Thus, potassium is oxidized.
At the same time:
- Hydrogen in water (H₂O) has an oxidation state of +1.
- It ends up as hydrogen gas (H₂), where it has an oxidation state of 0.
- Thus, hydrogen is reduced.
Potassium's role is crucial here: it provides the electrons that allow hydrogen to be reduced. This confirms that potassium acts as a reducing agent in this reaction, solidifying its characteristic +1 oxidation state upon forming a compound.
Bonding dictates how potassium behaves in stable compounds, but what happens when potassium is actively changing its oxidation state? To understand this, we need to explore the world of redox reactions.
Practical Examples: Oxidation Numbers in Potassium Compounds
To solidify our understanding of potassium's oxidation number, let's examine some common potassium compounds. We will dissect each compound and apply the rules we've learned to determine potassium's oxidation state. This will not only reinforce the concept but also demonstrate how it applies in real-world chemical scenarios.
Potassium Chloride (KCl)
Potassium chloride (KCl) is a simple ionic compound. Chlorine, being a halogen, almost always has an oxidation number of -1.
Since KCl is a neutral compound, the sum of the oxidation numbers must equal zero. Therefore, the oxidation number of potassium in KCl is +1. This balances out the -1 charge of the chlorine ion.
Potassium Hydroxide (KOH)
Potassium hydroxide (KOH) is another common compound. In this case, we have a hydroxide ion (OH-). Oxygen usually has an oxidation number of -2, and hydrogen typically has +1.
Therefore, the hydroxide ion as a whole has a -1 charge (-2 + 1 = -1). Since KOH is neutral, potassium must have an oxidation number of +1 to balance the charge of the hydroxide ion.
Potassium Oxide (K2O)
Potassium oxide (K2O) is formed between potassium and oxygen. Oxygen typically has an oxidation number of -2.
Since there are two potassium atoms in the compound, the total positive charge must be +2 to balance the -2 charge of the oxygen.
Therefore, each potassium atom has an oxidation number of +1 (+2 / 2 = +1).
Potassium Permanganate (KMnO4): A More Complex Example
Potassium permanganate (KMnO4) presents a slightly more complex example. It’s a good illustration of how to apply the rules when dealing with polyatomic ions.
Here, we need to consider the permanganate ion (MnO4-) as a whole. The overall charge of this ion is -1.
We know that oxygen usually has an oxidation number of -2, and there are four oxygen atoms, contributing a total of -8 to the permanganate ion.
Let 'x' be the oxidation number of manganese (Mn). We can set up the following equation: x + (4 * -2) = -1
Solving for x, we get x = +7. This means that manganese has an oxidation number of +7 in the permanganate ion.
Now, considering the entire KMnO4 compound, potassium balances the -1 charge of the MnO4- ion. As such, potassium still maintains an oxidation number of +1.
This example demonstrates that even within a complex ion, potassium's oxidation number remains consistent.
These examples illustrate that potassium almost invariably exhibits a +1 oxidation number in its compounds. This consistency is a direct result of its electronic structure and its position in the periodic table, solidifying its role as a reliable provider of positive charge in chemical compounds.
Bonding dictates how potassium behaves in stable compounds, but what happens when potassium is actively changing its oxidation state? To understand this, we need to explore the world of redox reactions.
Avoiding Pitfalls: Common Mistakes and Misconceptions
Even with a firm grasp of the rules, the concept of oxidation numbers can sometimes be tricky.
It's important to address common misunderstandings and clarify potential points of confusion.
Let's tackle some common pitfalls that can trip up even seasoned chemistry students.
Oxidation Number vs. Actual Charge
One of the most frequent misconceptions is equating oxidation number with the actual ionic charge.
While they often coincide, oxidation numbers are not always equal to the real charge on an ion.
Oxidation numbers are assigned based on a set of rules that prioritize electronegativity differences.
They represent a formalism used to track electron distribution, rather than a direct measurement of charge.
Consider covalent compounds: atoms share electrons rather than fully transferring them.
However, oxidation numbers are still assigned based on the assumption of complete electron transfer.
This can lead to oxidation numbers that don't reflect the true partial charges on the atoms involved in the bond.
Potassium's Consistent +1 Oxidation State
It's tempting to think that elements can exhibit a wide range of oxidation states, which is true for many transition metals.
However, potassium is an alkali metal and almost always displays a +1 oxidation number in its compounds.
This consistency is a key characteristic stemming from its electronic structure.
Its strong tendency to lose its single valence electron dictates its behavior.
While theoretically possible, finding potassium in any other oxidation state (+2, -1, etc.) is exceptionally rare under normal chemical conditions.
Navigating Complex Potassium Compounds
While potassium predominantly exhibits a +1 oxidation state, some compounds might appear more complex at first glance.
Potassium permanganate (KMnO4) is a prime example.
In this compound, the oxidation number of manganese (Mn) is +7, a far cry from potassium's +1.
However, to confirm, we can calculate and check that potassium still maintains its consistent +1 oxidation state.
The oxygen atoms, each with an oxidation state of -2, contribute a total of -8 (4 x -2 = -8).
For KMnO4 to be neutral, the sum of oxidation states must equal zero.
Therefore, Mn + (+1) + (-8) = 0, which rearranges to Mn = +7.
While the overall calculation appears complex, it is crucial to recognize that potassium still maintains its +1 oxidation state!
Always remember to systematically apply the rules for assigning oxidation numbers.
Break down complex molecules into their constituent atoms, determine the known oxidation states, and solve for the unknown.
By avoiding these common pitfalls and applying a systematic approach, you can confidently determine potassium's oxidation number in any compound.
Video: Potassium's Oxidation Number: Explained Simply! (60 Char)
Potassium's Oxidation Number: FAQs
Here are some frequently asked questions to help you understand the oxidation number of potassium and how it works.
Why is potassium's oxidation number almost always +1?
Potassium (K) is an alkali metal, belonging to Group 1 of the periodic table. Elements in Group 1 readily lose one electron to achieve a stable electron configuration. This loss of one electron results in a +1 oxidation number for potassium in nearly all its compounds.
Does potassium ever have a different oxidation number?
While extremely rare, potassium can exhibit an oxidation number other than +1 in exotic compounds under specific laboratory conditions. However, for virtually all practical applications and in everyday chemistry, you can confidently assume the potassium oxidation number is +1.
How does potassium's oxidation number relate to ionic compounds?
Potassium's tendency to lose one electron and achieve a +1 oxidation number makes it prone to forming ionic bonds. It donates its electron to a nonmetal, such as chlorine, forming potassium chloride (KCl). In KCl, potassium has a +1 oxidation number, and chlorine has a -1 oxidation number, reflecting the electron transfer.
How do I determine the oxidation number of other elements if potassium is present?
Knowing that potassium's oxidation number is almost always +1 simplifies determining oxidation numbers of other elements in a compound. Because the sum of oxidation numbers in a neutral compound must equal zero, the known +1 contribution from potassium allows you to calculate the oxidation number of the remaining elements.