Volumetric Flow Rate Explained: The Only Guide You Need!
Understanding volumetric flow rate is crucial for engineers at organizations like ASME, who rely on its accurate measurement for system design. Similarly, professions using Computational Fluid Dynamics (CFD) software benefit from a solid grasp of volumetric flow rate for precise simulations. This guide delves into the underlying principles of this measurement, explaining how it dictates the performance of systems that handle gases or liquids. By defining volumetric flow rate, we set the stage for understanding its applications in diverse fields, including applications in Chemical Engineering where precise measurements are critical.
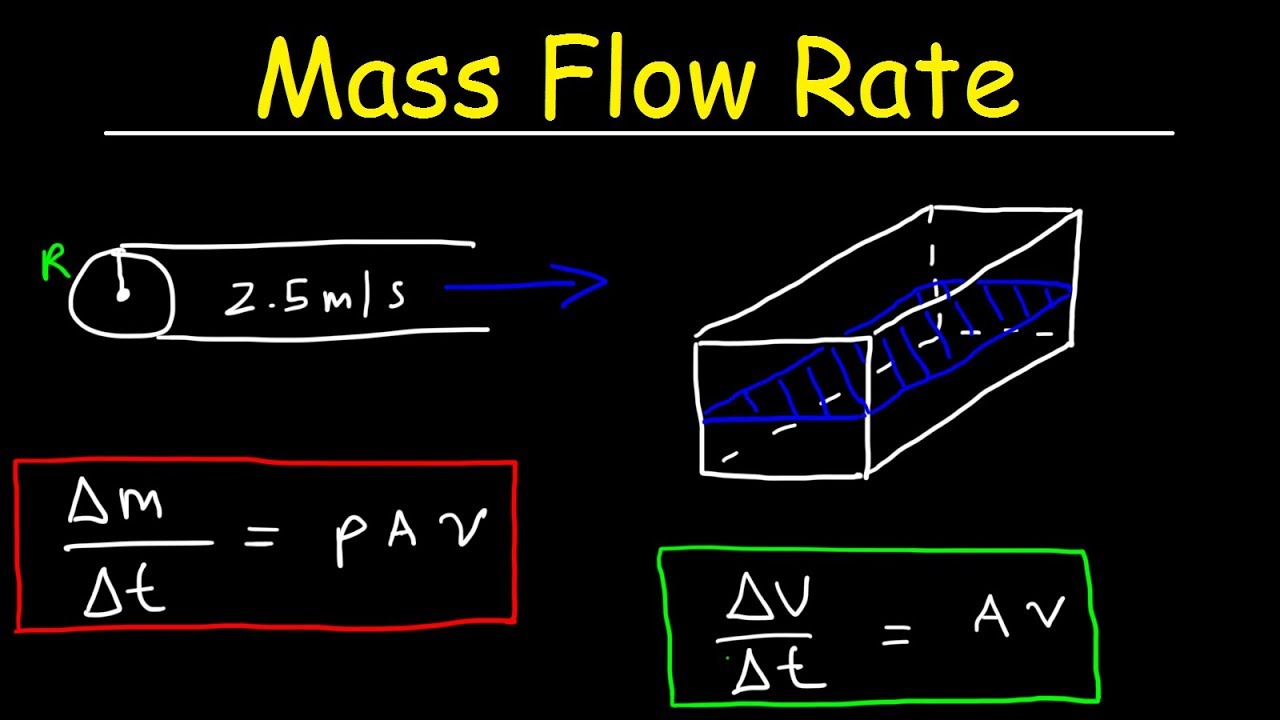
Image taken from the YouTube channel The Organic Chemistry Tutor , from the video titled Volume Flow Rate & Mass Flow Rate - Fluid Dynamics Physics Problems .
Flow rate is a fundamental concept that underpins numerous processes across diverse industries. From the controlled delivery of life-saving medications to the efficient transportation of natural gas across continents, the ability to quantify and manage flow is paramount.
But what exactly is flow rate, and why does it command such importance? Simply put, flow rate describes the quantity of a fluid that passes a particular point per unit of time. This "fluid" can be a liquid, a gas, or even a solid material conveyed in a fluid stream.
Its general importance stems from its direct impact on system performance, efficiency, and safety. Understanding flow rate helps to:
- Optimize processes
- Prevent equipment failure
- Ensure product quality
- Minimize waste
The Essence of Volumetric Flow Rate
Within the broader concept of flow rate lies volumetric flow rate, which is the specific focus of this guide. Volumetric flow rate quantifies the volume of fluid that passes a given point per unit of time.
Think of it like this: if you were filling a bucket with water, the volumetric flow rate would describe how many gallons (or liters) of water are entering the bucket every second or minute.
This measurement proves crucial in a vast array of applications:
- Chemical Engineering: Precisely controlling reactant flow rates for chemical reactions.
- Environmental Monitoring: Measuring wastewater discharge rates to ensure regulatory compliance.
- Healthcare: Delivering accurate doses of intravenous fluids to patients.
- Aerospace: Managing fuel flow to aircraft engines for optimal performance.
The sheer breadth of these applications underscores the critical nature of accurately determining and controlling volumetric flow rate.
What You Will Learn
This article serves as a comprehensive guide to understanding volumetric flow rate. We will delve into its definition, explore the units used to measure it, and dissect the formulas that govern its calculation.
We will also investigate the various tools employed to measure volumetric flow rate.
Furthermore, we will examine the factors that can influence flow rate and explore real-world examples of its applications across different sectors. By the end of this guide, you will possess a solid understanding of volumetric flow rate and its significance in the world around us.
Understanding Volumetric Flow Rate: Definition, Units, and Formula
The sheer breadth of these applications underscores the need for a solid understanding of volumetric flow rate, starting with its core definition, the units in which it is measured, and the formulas used to calculate it. This foundation is essential before delving into the complexities of flow measurement tools and influencing factors.
Defining Volumetric Flow Rate
Volumetric flow rate, at its essence, is the measure of how much volume of a fluid passes a specific point in a given amount of time. It’s a rate, indicating change per unit of time.
Consider a pipe through which water is flowing. The volumetric flow rate tells you how many cubic meters or gallons of water are moving past a particular cross-section of that pipe every second, minute, or hour.
The Dance of Volume and Time
The concept inherently links volume and time. A higher volumetric flow rate means a larger volume of fluid is passing by per unit of time, indicating a faster flow. Conversely, a lower volumetric flow rate signifies a smaller volume passing per unit of time, indicating a slower flow.
This relationship is crucial. In applications like intravenous fluid delivery, a precise volume needs to be administered over a specific time period. Understanding this relationship allows healthcare professionals to regulate the flow accordingly.
Common Units of Measurement
Volumetric flow rate is expressed in various units, each suitable for different scales and applications. Here are some of the most common:
-
Cubic meters per second (m³/s): This is the standard SI unit and is often used in large-scale industrial applications like measuring river flow or large chemical processes.
-
Liters per second (L/s): A more practical unit for medium-sized applications, such as municipal water supply or irrigation systems.
-
Gallons per minute (GPM): Commonly used in the United States, particularly in plumbing, HVAC systems, and automotive applications.
-
Cubic feet per minute (CFM): Often employed in HVAC systems for measuring air flow and in industries dealing with compressed gases.
The choice of unit depends on the context and the magnitude of the flow being measured.
The Formula: Q = A
**V
The fundamental formula for calculating volumetric flow rate is elegantly simple:
Q = A V**
Where:
- Q represents the volumetric flow rate.
- A represents the cross-sectional area of the flow.
- V represents the average velocity of the flow.
Deconstructing the Formula
The area (A) refers to the cross-sectional area through which the fluid is flowing. For a pipe, this would be the area of the circular opening.
The velocity (V) represents the average speed of the fluid moving through that area. It is important to note this is not necessarily the speed at one single point, but the average speed across the entire area.
Practical Applications of Q = A * V
Imagine determining the flow rate of water in a pipe with a diameter of 0.1 meters, where the water is flowing at an average velocity of 2 meters per second.
First, calculate the area: A = π (radius)² = π (0.05 m)² ≈ 0.00785 m².
Then, apply the formula: Q = A V = 0.00785 m² 2 m/s ≈ 0.0157 m³/s.
This tells us that approximately 0.0157 cubic meters of water are flowing through the pipe every second.
Understanding and applying this formula is a foundational step in managing and optimizing fluid flow in a myriad of engineering and scientific contexts. It provides a quantitative basis for controlling processes, ensuring efficiency, and maintaining safety in various applications.
Tools of the Trade: Measuring Volumetric Flow Rate
Having established a firm understanding of what volumetric flow rate is, how it's quantified, and the formulas that govern it, the natural progression is to explore how we actually measure it in the real world. A variety of instruments, each leveraging different physical principles, are employed to determine volumetric flow rate, catering to diverse applications and fluid types. Understanding these tools and their specific strengths and limitations is crucial for accurate flow measurement and effective process control.
Overview of Flow Meters
Flow meters are the workhorses of volumetric flow rate measurement, providing a means to quantify the amount of fluid moving through a pipe or channel. The landscape of flow meter technology is vast, with each design built upon distinct principles. Some common types include:
- Anemometers, ideal for air velocity measurements
- Pitot tubes, which utilize pressure differential
- Orifice plates and Venturi meters, both creating constrictions to measure flow
- Rotameters, which offer a direct visual reading
- Coriolis meters, known for their high accuracy
- Ultrasonic flow meters, offering a non-intrusive approach.
A Detailed Look at Specific Instruments
Let's examine the inner workings and applications of some of the most prevalent flow measurement instruments.
Anemometers
Anemometers are primarily used to measure air velocity, which can then be used to calculate volumetric flow rate if the cross-sectional area of the flow is known. These instruments are commonly employed in HVAC systems, weather monitoring, and wind tunnel experiments.
Types of Anemometers
Two common types are:
- Hot-wire anemometers use a heated wire to measure air velocity. As air flows past the wire, it cools the wire down. The amount of current required to maintain the wire at a constant temperature is proportional to the air velocity.
- Vane anemometers use a rotating vane to measure air velocity. The vane spins at a rate proportional to the air velocity. A sensor measures the rotational speed of the vane, which is then converted to air velocity.
Pitot Tubes
Pitot tubes are simple yet effective devices used to measure fluid velocity, particularly in pipes and ducts. They operate based on the principle of pressure differential.
Principle of Operation
A pitot tube measures two pressures: the static pressure of the fluid and the total pressure (also known as stagnation pressure). The difference between these two pressures, known as the dynamic pressure, is directly related to the fluid velocity. Using Bernoulli's equation, the velocity can be calculated from this pressure difference.
Orifice Plates
Orifice plates are a type of differential pressure flow meter that create a restriction in the flow path, causing a pressure drop. The magnitude of this pressure drop is related to the flow rate.
Principle of Operation
By measuring the pressure upstream and downstream of the orifice plate, the flow rate can be determined. Orifice plates are relatively inexpensive and easy to install, making them a popular choice for many applications. However, they can cause a significant permanent pressure loss in the system.
Venturi Meters
Venturi meters, like orifice plates, create a pressure drop to measure flow rate, but they do so with a more gradual constriction and expansion.
Advantages of Venturi Meters
This design results in a lower permanent pressure loss compared to orifice plates and generally higher accuracy. Venturi meters are commonly used in applications where minimizing pressure loss is important, such as in water distribution systems.
Rotameters
Rotameters are direct-reading flow meters that use a float inside a tapered tube.
Simplicity and Direct Reading
The fluid flows upward through the tube, causing the float to rise. The height of the float is proportional to the flow rate. Rotameters are simple, relatively inexpensive, and provide a direct visual indication of flow rate, making them suitable for a wide range of applications.
Coriolis Meters
Coriolis meters are sophisticated devices that directly measure mass flow rate.
High Accuracy and Mass Flow Measurement
They operate by inducing a vibration in a tube through which the fluid flows. The Coriolis effect causes a twist in the tube, which is proportional to the mass flow rate. While primarily used for mass flow, Coriolis meters can also determine volumetric flow rate by measuring the fluid density. These meters are highly accurate and suitable for a wide range of fluids, including viscous liquids and slurries.
Ultrasonic Flow Meters
Ultrasonic flow meters utilize sound waves to measure fluid velocity. They are non-intrusive, meaning they do not require any physical obstruction in the flow path.
Transit-Time and Doppler Principles
There are two main types of ultrasonic flow meters:
- Transit-time flow meters measure the time it takes for an ultrasonic pulse to travel upstream and downstream in the fluid. The difference in these transit times is related to the fluid velocity.
- Doppler flow meters rely on the Doppler effect. Ultrasonic waves are reflected off particles in the fluid, and the frequency shift of the reflected waves is proportional to the fluid velocity.
Ultrasonic flow meters are suitable for a wide range of liquid and gas applications and are particularly useful where non-intrusive measurement is required.
Considerations When Selecting Flow Meters
Choosing the right flow meter for a specific application requires careful consideration of several factors.
Accuracy and Uncertainty
Accuracy refers to how close a measurement is to the true value, while uncertainty quantifies the range of possible values within which the true value is likely to lie. In flow measurement, both accuracy and uncertainty are critical considerations.
Key Selection Factors
Several factors must be considered when selecting a flow meter:
- Fluid type: Different flow meters are suitable for different fluids. For example, some flow meters may not be compatible with corrosive fluids or fluids containing solids.
- Flow range: The flow meter must be able to accurately measure the expected range of flow rates.
- Accuracy requirements: The required accuracy of the flow measurement will influence the choice of flow meter.
- Cost: The cost of the flow meter is also a factor to consider. More accurate flow meters tend to be more expensive.
- Installation constraints: The installation requirements of the flow meter, such as space limitations or the need for straight pipe runs, must be considered.
Having examined the instruments used to quantify volumetric flow rate, it's equally important to recognize that the measurements they provide are not simply absolute values. The flow rate is influenced by a variety of factors inherent to the fluid itself and the external conditions surrounding the system. Accurately interpreting flow meter readings and effectively controlling flow processes requires an understanding of these influences.
Factors Influencing Volumetric Flow Rate
Volumetric flow rate isn't a static property; it's a dynamic characteristic shaped by both the fluid's inherent properties and the external environment in which it flows. These influencing factors must be considered to achieve accurate flow measurement and maintain optimal process control.
Fluid Properties
The characteristics of the fluid itself play a significant role in determining volumetric flow rate. These properties, governed by the principles of fluid mechanics and fluid dynamics, directly impact how a fluid behaves under specific conditions.
The Role of Fluid Mechanics and Fluid Dynamics
Fluid mechanics is the branch of physics that studies the behavior of fluids (liquids and gases) at rest and in motion. Fluid dynamics, a subdiscipline of fluid mechanics, focuses specifically on fluids in motion.
These fields provide the theoretical framework for understanding how fluid properties like viscosity, density, and compressibility affect flow. Viscosity, for instance, represents a fluid's resistance to flow.
Higher viscosity translates to a lower volumetric flow rate, assuming all other factors remain constant. Similarly, the principles of fluid dynamics explain how pressure gradients and flow geometry influence fluid velocity, which directly affects the volumetric flow rate.
The Impact of Fluid Density
Density, defined as mass per unit volume, is another crucial fluid property that influences flow rate. While density doesn't directly appear in the Q = A V formula for incompressible fluids (liquids), it plays a more critical role when considering compressible* fluids (gases).
Changes in density, often caused by variations in temperature or pressure, can significantly alter the volumetric flow rate of a gas. A higher density gas will exhibit a lower volumetric flow rate compared to a less dense gas, assuming the same mass flow rate is maintained.
External Factors
Beyond the fluid's inherent properties, external conditions can also exert a considerable influence on volumetric flow rate. Pressure and temperature are the primary external factors that can significantly alter flow behavior.
Pressure's Influence on Volumetric Flow Rate
Pressure has a direct and pronounced effect on volumetric flow rate, particularly for compressible fluids like gases. According to the ideal gas law, the volume of a gas is inversely proportional to its pressure (at constant temperature).
This means that increasing the pressure on a gas will compress it, reducing its volume and, consequently, its volumetric flow rate. Conversely, decreasing the pressure will allow the gas to expand, increasing its volumetric flow rate.
Therefore, when measuring the volumetric flow rate of gases, it's crucial to account for pressure variations and, if necessary, correct the measurements to standard conditions.
Temperature's Influence
Temperature affects nearly all fluid properties, including density, viscosity, and vapor pressure. As temperature increases, the density of most fluids decreases, while viscosity typically decreases as well.
For liquids, the change in density with temperature is usually less significant than for gases. However, the change in viscosity can still influence the flow rate, particularly in applications involving viscous fluids.
For gases, temperature has a more substantial impact on both density and volumetric flow rate. As temperature increases, the gas expands, leading to a higher volumetric flow rate, assuming the mass flow rate remains constant.
Therefore, accurate temperature measurement and compensation are vital for precise volumetric flow rate determination, especially in applications with significant temperature fluctuations.
Real-World Applications of Volumetric Flow Rate
Volumetric flow rate is not merely a theoretical concept confined to textbooks and laboratories. It's a fundamental parameter that underpins the operation and optimization of countless processes across diverse industries and everyday scenarios. Understanding its practical implications reveals just how critical accurate measurement and control of volumetric flow rate truly are.
Industrial Applications: The Backbone of Modern Processes
In the industrial sector, volumetric flow rate is a critical determinant of efficiency, safety, and product quality. Let's explore some key areas:
HVAC Systems: Maintaining Comfort and Efficiency
Heating, ventilation, and air conditioning (HVAC) systems rely heavily on precise volumetric flow rate control to ensure optimal performance. The correct airflow rate is essential for maintaining comfortable temperatures, providing adequate ventilation, and ensuring good air quality within buildings and industrial spaces.
Insufficient airflow can lead to stuffiness, poor temperature regulation, and the buildup of pollutants. Conversely, excessive airflow wastes energy and can create uncomfortable drafts. Sophisticated control systems, often incorporating flow meters and variable frequency drives, are used to modulate fan speeds and damper positions. This will optimize airflow rates based on real-time conditions and occupancy levels.
Chemical Processing: Precision for Reactions and Quality
In chemical processing plants, volumetric flow rate is paramount for controlling chemical reactions, mixing ingredients, and ensuring consistent product quality. Many chemical reactions require precise ratios of reactants, which are achieved through accurate flow rate control.
Variations in flow rates can lead to incomplete reactions, formation of unwanted byproducts, and inconsistent product properties. Flow meters are often integrated into sophisticated process control systems to monitor and adjust flow rates of various chemicals and solvents. This will ensure optimal reaction conditions and maintain high product standards.
Water Treatment: Ensuring Safe and Clean Water
Water treatment plants depend on volumetric flow rate control for a wide range of processes. These processes range from filtration and disinfection to distribution. Accurate flow rate measurement is essential for optimizing the performance of filters, ensuring adequate contact time for disinfection processes (e.g., chlorination, UV treatment), and maintaining consistent water pressure throughout the distribution network.
Overly rapid flow rates through filters can reduce their effectiveness, allowing contaminants to pass through. Insufficient flow rates during disinfection may not provide adequate exposure to kill harmful microorganisms. Flow meters and control valves are used to carefully manage flow rates at each stage of the water treatment process. This ensures the delivery of safe and clean drinking water to consumers.
Oil and Gas Industry: From Extraction to Distribution
The oil and gas industry relies on volumetric flow rate measurement and control for numerous operations. From pipeline transportation to refining processes, and the precise flow metering of crude oil and natural gas, it's an indispensable parameter.
Accurate flow measurement is crucial for custody transfer (the point at which ownership of the oil or gas changes hands). It is also crucial for optimizing pipeline throughput, and monitoring the efficiency of refining processes. Sophisticated flow meters, such as ultrasonic and Coriolis meters, are used to measure the flow rates of various fluids and gases. These advanced tools ensure accurate accounting and efficient operation of oil and gas facilities.
Other Applications: Tailoring Flow to Specific Needs
Beyond the large-scale industrial applications, volumetric flow rate plays a vital role in specialized settings.
Standard Cubic Feet per Minute (SCFM): Gases at Standard Conditions
SCFM provides a standardized measure of gas flow, accounting for variations in temperature and pressure. This is particularly important when comparing gas flows under different conditions, as the volume of a gas changes with temperature and pressure.
SCFM is widely used in industries that handle gases, such as manufacturing, electronics, and laboratories, to ensure accurate gas metering and process control. It provides a common reference point for comparing gas flow rates. This allows engineers and technicians to design and operate systems with predictable and reliable performance.
Liters per Minute (LPM): Precision in Medical and Laboratory Settings
LPM is a commonly used unit of volumetric flow rate in medical and laboratory applications. It’s especially useful when precision and control over small volumes of fluids or gases are essential.
In medical settings, LPM is used to measure and control the flow rate of oxygen delivered to patients, intravenous fluids administered during treatments, and gases used in anesthesia. In laboratories, LPM is used for dispensing precise volumes of reagents, controlling gas flows in analytical instruments, and conducting experiments that require accurate flow control. The small volumes of LPM allow for highly precise adjustments and measurements, critical for the sensitivity of these applications.
Delving Deeper: Advanced Concepts (Optional)
While a solid understanding of the basic principles of volumetric flow rate is sufficient for many applications, a deeper dive into related concepts can provide valuable insights and enhance problem-solving capabilities. This section explores some advanced topics for those seeking a more comprehensive understanding. Readers comfortable with their current grasp of volumetric flow rate can safely skip this section without losing the core message of this guide.
The Continuity Equation: Flow Rate and Conservation of Mass
The continuity equation is a fundamental principle in fluid dynamics that expresses the conservation of mass in a flowing fluid. In essence, it states that in a closed system, the mass flow rate remains constant. For an incompressible fluid (density remains constant), this principle directly relates to volumetric flow rate.
Mathematically, the continuity equation is often expressed as:
A₁V₁ = A₂V₂
Where:
- A₁ and A₂ are the cross-sectional areas at two different points in the flow path.
- V₁ and V₂ are the average fluid velocities at those respective points.
This equation tells us that if the cross-sectional area of a pipe decreases, the fluid velocity must increase to maintain a constant volumetric flow rate.
Applications of the Continuity Equation
The continuity equation has numerous practical applications in analyzing fluid flow. It is used to predict velocity changes in pipes with varying diameters, analyze flow distributions in branching systems, and design efficient flow networks. For example, in designing a nozzle, engineers use the continuity equation to determine the required outlet diameter to achieve a desired exit velocity for a given flow rate.
Bernoulli's Principle: Pressure, Velocity, and Flow
Bernoulli's principle describes the relationship between fluid pressure, velocity, and elevation in a flowing fluid. It states that an increase in fluid velocity occurs simultaneously with a decrease in pressure or a decrease in the fluid's potential energy (elevation). Bernoulli's principle is a cornerstone of fluid dynamics and has significant implications for flow measurement.
Applications in Flow Measurement
Bernoulli's principle forms the basis for several flow measurement devices, including Venturi meters and Pitot tubes.
-
Venturi Meters: Venturi meters use a converging section to increase fluid velocity, which results in a pressure drop. By measuring this pressure difference between the wider upstream section and the narrow throat, the volumetric flow rate can be accurately determined using Bernoulli's equation. The gradual expansion after the throat helps in recovering most of the pressure drop, making it energy-efficient.
-
Pitot Tubes: Pitot tubes measure the stagnation pressure of a fluid, which is the pressure at a point where the fluid is brought to rest. By comparing the stagnation pressure with the static pressure (the pressure of the fluid in undisturbed flow), the fluid velocity can be determined using Bernoulli's equation. This velocity measurement can then be used to calculate the volumetric flow rate.
It's worth noting that Bernoulli's principle assumes ideal fluid flow (inviscid and incompressible) and does not account for energy losses due to friction or turbulence. In real-world applications, correction factors are often applied to account for these effects.
Volumetric Flow Rate vs. Mass Flow Rate: Choosing the Right Metric
While volumetric flow rate measures the volume of fluid passing a point per unit time, mass flow rate measures the mass of fluid passing a point per unit time. The relationship between the two is straightforward:
Mass Flow Rate = Volumetric Flow Rate × Fluid Density
The choice between using volumetric flow rate and mass flow rate depends on the application.
-
Volumetric flow rate is often used when dealing with incompressible fluids (liquids) or when the density variations are negligible. It is convenient for applications where volume is the primary concern, such as filling containers or measuring liquid consumption.
-
Mass flow rate is more appropriate when dealing with compressible fluids (gases) or when density variations are significant. It is essential for applications where the amount of substance is critical, such as chemical reactions or combustion processes. Mass flow rate provides a more accurate representation of the amount of fluid being transferred, regardless of changes in temperature or pressure that can affect density and thus volumetric flow rate.
In summary, understanding the nuances of the continuity equation, Bernoulli's principle, and the distinction between volumetric and mass flow rate provides a more complete picture of fluid dynamics and enhances the ability to analyze and optimize flow-related processes.
Video: Volumetric Flow Rate Explained: The Only Guide You Need!
FAQs: Understanding Volumetric Flow Rate
Here are some frequently asked questions about volumetric flow rate to help solidify your understanding of this crucial concept.
What units are used to measure volumetric flow rate?
Common units for measuring volumetric flow rate include cubic meters per second (m³/s), liters per minute (L/min), cubic feet per second (ft³/s), and gallons per minute (GPM). The appropriate unit depends on the scale of the flow being measured.
How does temperature affect volumetric flow rate?
Temperature can affect the density of a fluid. Because volumetric flow rate measures volume per unit of time, a change in density due to temperature variations can influence the volumetric flow rate reading if mass flow rate is constant.
What's the difference between volumetric flow rate and mass flow rate?
Volumetric flow rate measures the volume of fluid passing a point per unit of time, while mass flow rate measures the mass of fluid passing a point per unit of time. Volumetric flow rate is affected by temperature and pressure changes that influence density, while mass flow rate is not.
Where is volumetric flow rate commonly used?
Volumetric flow rate calculations are essential in many fields, including fluid mechanics, chemical engineering, HVAC systems, and medical devices. They are used for designing pipelines, controlling fluid processes, and measuring respiratory function, among many other applications.