Positive Control: Your Key To Valid Results Every Time!
In scientific validation, the National Institute of Standards and Technology (NIST) recognizes accurate measurement as crucial; therefore, employing a positive control is paramount. A positive control, when performed correctly, verifies that the assay system is functioning as expected. This ensures the reagents are not damaged. Good Laboratory Practice (GLP) guidelines advocate for its routine incorporation in experiments to ensure that any negative result isn't due to system failure but rather reflects the absence of the target analyte. Without the rigorous application of positive control measures, the reliability of results could be compromised, leading to inaccurate conclusions and hindering scientific advancement.
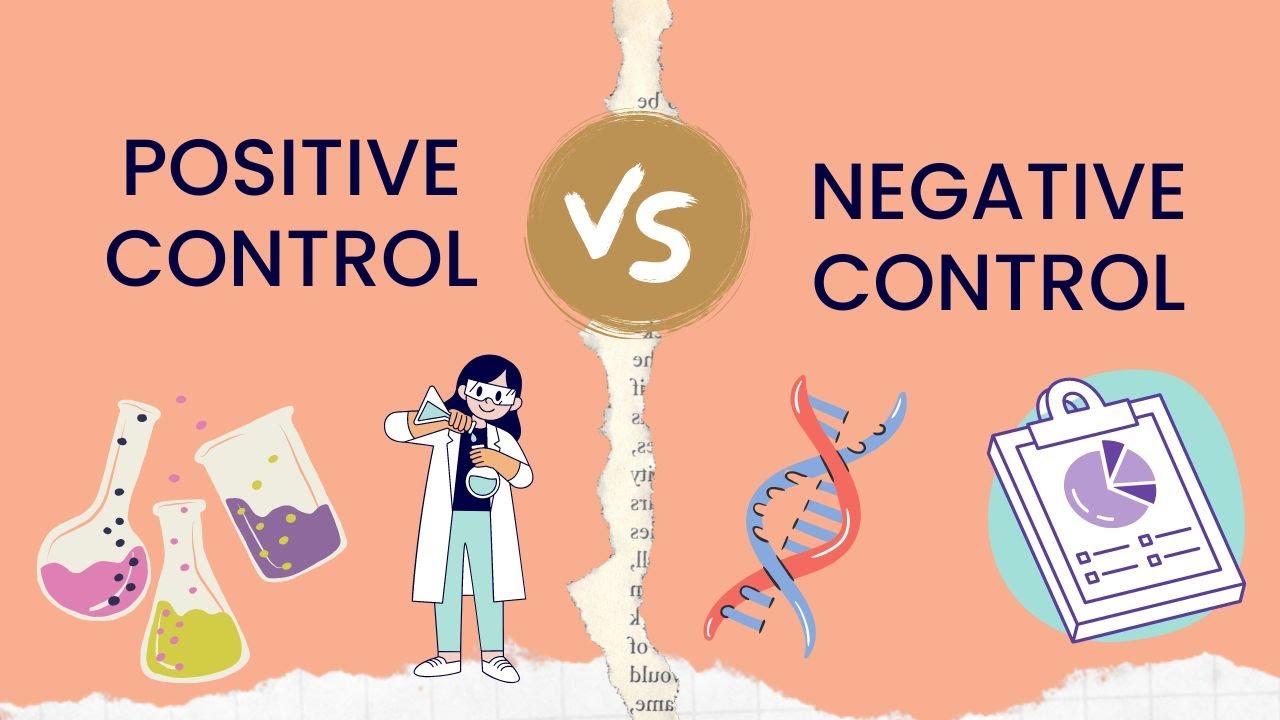
Image taken from the YouTube channel WInspire , from the video titled Positive Control vs Negative Control | Experimental Group .
Imagine a scenario all too familiar in research labs: weeks spent meticulously conducting experiments, only to be met with ambiguous, inconsistent, or outright unreliable results.
The frustration is palpable, the wasted resources a significant setback. Often, the culprit isn't a flaw in the core hypothesis but rather a deficiency in the experimental design, specifically, the omission or improper use of a positive control.
Defining the Positive Control
In the realm of laboratory testing, a positive control is a sample or substance known to produce a positive result for the test being performed. It serves as a benchmark, a guiding light confirming that the testing system is indeed capable of detecting the target analyte or phenomenon.
Its importance cannot be overstated, particularly in fields where accuracy and reliability are paramount. Consider its role in clinical diagnostics, pharmaceutical research, and environmental monitoring: without a properly functioning positive control, we risk misdiagnosis, ineffective treatments, and compromised safety standards.
The Linchpin of Laboratory Testing
The absence of a reliable positive control undermines the entire experimental process. Without it, we cannot confidently assert that a negative result truly indicates the absence of the target analyte, or that a positive result is not due to some confounding factor.
It’s akin to attempting to bake a cake with an oven that may or may not be working, or using faulty equipment to measure the density of a substance.
The Core of Scientific Validity
The thesis underpinning this exploration is simple yet profound: the proper use of positive controls is not merely a best practice, but a fundamental requirement for ensuring valid scientific outcomes.
They are the bedrock upon which reliable research is built, the cornerstone of scientific confidence. Ignoring their significance is to compromise the integrity and reproducibility of our investigations, hindering progress and eroding trust in scientific findings.
Imagine a scenario all too familiar in research labs: weeks spent meticulously conducting experiments, only to be met with ambiguous, inconsistent, or outright unreliable results. The frustration is palpable, the wasted resources a significant setback. Often, the culprit isn't a flaw in the core hypothesis but rather a deficiency in the experimental design, specifically, the omission or improper use of a positive control. Defining the Positive Control In the realm of laboratory testing, a positive control is a sample or substance known to produce a positive result for the test being performed. It serves as a benchmark, a guiding light confirming that the testing system is indeed capable of detecting the target analyte or phenomenon. Its importance cannot be overstated, particularly in fields where accuracy and reliability are paramount. Consider its role in clinical diagnostics, pharmaceutical research, and environmental monitoring: without a properly functioning positive control, we risk misdiagnosis, ineffective treatments, and compromised safety standards. The Linchpin of Laboratory Testing The absence of a reliable positive control undermines the entire experimental process. Without it, we cannot confidently assert that a negative result truly indicates the absence of the target analyte, or that a positive result is not due to some confounding factor. It’s akin to attempting to bake a cake with an oven that may or may not be working, or using faulty equipment to measure the density of a substance. The Core of Scientific Validity The thesis underpinning this exploration is simple yet profound: the proper use of positive controls ensures valid scientific outcomes.
This brings us to a critical question: what exactly constitutes a positive control, and how does it function within the landscape of experimental design? Understanding its purpose, how it differs from other control types, and its interaction with the experimental group is crucial for building a foundation of sound scientific practice.
What is a Positive Control? A Detailed Explanation
At its core, a positive control is a specimen or intervention designed to yield an expected, affirmative result in a particular test or assay. It's a substance with a proven track record of eliciting a positive reaction, serving as a reliable indicator that the testing system is functioning correctly.
Defining the Positive Result
The key lies in the predefined expectation.
We know, with a high degree of certainty, that this control should trigger a specific response. This response, in turn, validates the assay's ability to detect the target analyte or phenomenon.
Without this confirmation, any subsequent results obtained from experimental samples would be rendered questionable, if not entirely invalid.
Confirming Sensitivity: The Purpose of a Positive Control
The primary purpose of a positive control is to confirm the sensitivity of the testing system. Sensitivity, in this context, refers to the assay's ability to correctly identify a positive instance.
In other words, can the test accurately detect the presence of the target analyte when it is, in fact, present?
The positive control acts as the yardstick against which the assay's performance is measured.
If the positive control fails to produce the expected positive result, it signals a potential problem with the reagents, equipment, or the assay protocol itself.
This prompts immediate troubleshooting, preventing erroneous results from propagating through the experiment.
Positive vs. Negative Controls: Two Sides of the Same Coin
To fully appreciate the significance of a positive control, it's essential to contrast it with its counterpart: the negative control.
While the positive control confirms the assay's ability to detect a positive result, the negative control validates its ability to exclude a positive result when the target analyte is absent.
The negative control should, ideally, produce no reaction or a baseline level of reaction. It guards against false positives.
Both controls are vital for ensuring the accuracy and reliability of the results. A flawed assay will either produce false positives or false negatives or both.
A well-designed experiment incorporates both positive and negative controls to provide a comprehensive assessment of the testing system's performance.
Positive Controls Within the Control Group
The positive control doesn't operate in isolation; it is an integral part of the broader control group.
The control group serves as a baseline for comparison, providing a reference point against which the effects of the experimental intervention can be evaluated.
Alongside the positive and negative controls, the control group might include other controls designed to account for potential confounding factors.
By meticulously controlling various parameters, researchers can isolate the specific effect of the experimental variable with greater confidence.
The Experimental Group and its Relationship with the Positive Control
The experimental group is the group of samples or subjects that are subjected to the experimental manipulation or treatment.
The results obtained from the experimental group are then compared to those of the control group, including the positive control, to determine the effect of the intervention.
If the experimental group yields a positive result comparable to that of the positive control, it suggests that the experimental intervention is effective in eliciting the desired response.
Conversely, a negative result in the experimental group, coupled with a positive result in the positive control, indicates that the intervention did not produce the intended effect.
In summary, the positive control is not merely an ancillary component of an experiment; it is a cornerstone upon which the validity of the entire investigation rests. Its function is to give a reference for comparison to indicate the efficacy of the experiment.
Imagine a scenario all too familiar in research labs: weeks spent meticulously conducting experiments, only to be met with ambiguous, inconsistent, or outright unreliable results. The frustration is palpable, the wasted resources a significant setback. Often, the culprit isn't a flaw in the core hypothesis but rather a deficiency in the experimental design, specifically, the omission or improper use of a positive control. Defining the Positive Control In the realm of laboratory testing, a positive control is a sample or substance known to produce a positive result for the test being performed. It serves as a benchmark, a guiding light confirming that the testing system is indeed capable of detecting the target analyte or phenomenon. Its importance cannot be overstated, particularly in fields where accuracy and reliability are paramount. Consider its role in clinical diagnostics, pharmaceutical research, and environmental monitoring: without a properly functioning positive control, we risk misdiagnosis, ineffective treatments, and compromised safety standards. The Linchpin of Laboratory Testing The absence of a reliable positive control undermines the entire experimental process. Without it, we cannot confidently assert that a negative result truly indicates the absence of the target analyte, or that a positive result is not due to some confounding factor. It’s akin to attempting to bake a cake with an oven that may or may not be working, or using faulty equipment to measure the density of a substance. The Core of Scientific Validity The thesis underpinning this exploration is simple yet profound: the proper use of positive controls ensures valid scientific outcomes. But why is this seemingly simple element so vital? Its true value lies in its multifaceted ability to ensure accuracy, validate processes, and ultimately, uphold the integrity of scientific inquiry.
Why Are Positive Controls So Important? Ensuring Accuracy and Validity
The positive control is not merely an optional addition to an experiment; it is an indispensable component that underpins the reliability and interpretability of the results. Its presence is the key to validating the entire laboratory testing process and confirming its functionality, thereby safeguarding the integrity of the data produced.
Validating the Entire Laboratory Testing Process
The positive control acts as a global validator, confirming that all steps in the testing process are functioning as expected. This includes proper sample preparation, accurate reagent delivery, appropriate incubation times, and effective detection methods.
If the positive control fails to produce the expected positive result, it indicates a systemic issue within the process.
This failure signals the need for immediate investigation and corrective action before any further tests are conducted.
Confirming Reagent Functionality
Reagents are the workhorses of any laboratory test. Their integrity and proper function are critical for accurate results. Positive controls provide a direct means of verifying that reagents are active and capable of producing the expected reaction.
A malfunctioning reagent can lead to false negative results or, even worse, misleading positive signals.
By routinely including a positive control, we can proactively identify compromised reagents and prevent inaccurate data from being generated.
Troubleshooting Errors
When unexpected results occur, the positive control serves as a powerful diagnostic tool. It helps to narrow down the potential sources of error.
If the positive control produces the expected result, the issue is likely related to the sample itself or to a step in the procedure specific to the experimental group. However, if the positive control fails, the problem lies within the general testing system, such as a malfunctioning instrument, compromised reagents, or procedural errors.
This targeted troubleshooting approach saves time and resources by focusing the investigation on the most likely causes of the discrepancy.
The Positive Control and the Scientific Method
The inclusion of positive controls is deeply intertwined with the rigorous principles of the scientific method. Science relies on controlled experimentation to draw valid conclusions. The positive control provides a critical element of this control, enabling scientists to differentiate between true negative results and those caused by a failure of the testing system.
By adhering to this rigorous approach, we strengthen the reliability and reproducibility of our findings, thus contributing to the advancement of scientific knowledge.
Minimizing False Positives and False Negatives
False positive and false negative results can have dire consequences in various fields, leading to incorrect diagnoses, ineffective treatments, or flawed environmental assessments.
Positive controls play a crucial role in minimizing these errors.
By confirming the sensitivity of the assay, they reduce the likelihood of false negative results, ensuring that true positives are not missed. Furthermore, when combined with negative controls, they help to refine the specificity of the assay, minimizing the risk of false positives.
Achieving Specificity
Specificity refers to the ability of a test to accurately identify the target analyte without producing a positive result for other, similar substances.
The positive control helps to establish and maintain the specificity of the assay. By demonstrating a clear positive signal for the target analyte, while negative controls confirm the absence of a signal for non-target substances, the assay's specificity is validated.
This ensures that the test is truly measuring what it is intended to measure, preventing misleading results and ensuring the reliability of the data.
Imagine those experiments that yield consistently reliable results, experiments that not only confirm your hypothesis but also withstand the scrutiny of peer review. The key to achieving such scientific rigor often lies not just in the experimental design itself, but in the meticulous implementation of positive controls.
Best Practices for Implementing Positive Controls
Choosing the right positive control and using it correctly is paramount to obtaining meaningful data. A poorly selected or mishandled positive control can be just as detrimental as having none at all. Let's look at some best practices.
Selecting the Right Positive Control: A Critical First Step
The selection of an appropriate positive control is not an arbitrary decision. It requires careful consideration of the assay being performed and the target analyte being investigated.
The ideal positive control should:
- Contain the target analyte or exhibit the specific activity being measured.
- Be as similar as possible to the samples being tested, to account for matrix effects.
- Be stable and readily available, ensuring consistent performance over time.
For example, when conducting a PCR assay for a specific virus, the positive control should contain a known quantity of that viral DNA or RNA. In enzyme activity assays, a purified enzyme with known activity is often used.
It's also crucial to consider the concentration of the positive control. It should be high enough to generate a clearly detectable signal, but not so high as to saturate the assay and mask potential issues.
Preparation and Storage: Maintaining Integrity
Once a suitable positive control is selected, proper preparation and storage are essential for maintaining its integrity and ensuring reliable performance.
This includes:
- Accurate Reconstitution: If the positive control is supplied in a lyophilized (freeze-dried) form, follow the manufacturer's instructions precisely for reconstitution. Use the correct diluent and ensure complete dissolution.
- Appropriate Aliquoting: Avoid repeated freeze-thaw cycles by aliquoting the reconstituted positive control into smaller volumes. This prevents degradation and maintains consistent activity.
- Proper Storage Conditions: Store the aliquots at the recommended temperature (typically -20°C or -80°C) in a frost-free freezer to prevent temperature fluctuations.
- Regular Monitoring: Establish a system for regularly monitoring the positive control's performance. This could involve running it periodically with a known standard or comparing its results to historical data.
Any signs of degradation or inconsistent performance should prompt immediate investigation and replacement of the positive control.
Running Positive Controls Alongside Samples: Ensuring Concurrent Validity
The practice of running positive controls concurrently with experimental samples is not merely a procedural step; it’s a cornerstone of valid experimental design. This ensures that the entire testing system, including reagents, equipment, and operator technique, is functioning correctly at the time of the experiment.
If the positive control fails to produce the expected result, it indicates a systemic problem that could invalidate the results obtained for the experimental samples.
This concurrent approach allows for immediate troubleshooting and corrective action, preventing the propagation of erroneous data.
Documenting Control Results: Maintaining a Clear Audit Trail
Meticulous documentation of positive control results is essential for maintaining a clear audit trail and ensuring the reproducibility of experimental findings.
This documentation should include:
- The identity and lot number of the positive control.
- The date and time of the experiment.
- The results obtained for the positive control, including raw data and calculated values.
- Any observations or deviations from the expected performance.
- The initials of the person performing the experiment.
This comprehensive record not only facilitates troubleshooting and quality control, but it also provides valuable information for future experiments and assay development.
Standard Operating Procedures (SOPs): Promoting Consistency and Reliability
Developing robust Standard Operating Procedures (SOPs) for the use of positive controls is crucial for promoting consistency and reliability across all experiments.
These SOPs should outline:
- The selection criteria for positive controls.
- The procedures for preparation, storage, and handling.
- The frequency and timing of control runs.
- The acceptance criteria for control performance.
- The corrective actions to be taken if a control fails.
- The documentation requirements for control results.
By adhering to well-defined SOPs, laboratories can minimize variability, reduce the risk of errors, and ensure the consistent and reliable use of positive controls in all experiments.
In conclusion, implementing these best practices for positive controls is not just about following a checklist; it's about fostering a culture of scientific rigor and ensuring the validity of your experimental results.
Imagine those experiments that yield consistently reliable results, experiments that not only confirm your hypothesis but also withstand the scrutiny of peer review. The key to achieving such scientific rigor often lies not just in the experimental design itself, but in the meticulous implementation of positive controls.
Best practices are paramount for ensuring controls produce meaningful data. A poorly selected or mishandled positive control can be just as detrimental as having none at all. With a firm understanding of appropriate controls, we can turn our attention to assay validation and how positive controls are used within that process.
The Positive Control in Assay Validation: A Critical Step
Assay validation is the process of demonstrating that an assay is fit for its intended purpose. It's a critical step in ensuring the reliability and accuracy of laboratory results. Without proper validation, the data generated from an assay may be meaningless or, worse, misleading.
Defining Assay Validation and Its Necessity
Assay validation is a comprehensive evaluation of an assay's performance characteristics. This includes assessing its accuracy, precision, sensitivity, specificity, and robustness.
The need for assay validation stems from the inherent variability in laboratory testing. Factors such as reagent quality, equipment performance, and operator technique can all influence assay results.
Assay validation provides the documented evidence that the assay can perform reliably and consistently, even when subjected to these variables. It is also a regulatory requirement in many fields, including pharmaceuticals and clinical diagnostics.
Positive Controls: Demonstrating Sensitivity and Reproducibility
Positive controls are indispensable tools in assay validation, playing a crucial role in demonstrating both sensitivity and reproducibility.
Sensitivity
Sensitivity refers to an assay's ability to detect even small amounts of the target analyte. A positive control with a known, low concentration of the analyte should consistently produce a positive result if the assay is sufficiently sensitive.
If the positive control fails to register a positive result, it indicates a problem with the assay's sensitivity, potentially due to reagent degradation, instrument malfunction, or improper technique.
Reproducibility
Reproducibility refers to an assay's ability to generate consistent results when repeated multiple times under the same conditions.
By running multiple replicates of the positive control, you can assess the variability of the assay and determine whether it meets the predefined acceptance criteria.
Significant variations in the positive control results may indicate instability of the assay, matrix effects, or operator-dependent variability.
Establishing Acceptance Criteria for Positive Control Performance
Establishing clear acceptance criteria for positive control performance is a critical part of assay validation. These criteria define the acceptable range of results for the positive control. Results outside this range indicate that the assay is not performing as expected.
Acceptance criteria should be based on the assay's intended use and the acceptable level of variability. They can be statistically derived from historical data or based on manufacturer's specifications.
Documenting the rationale behind the acceptance criteria is also crucial for transparency and auditability.
Examples of Positive Controls in Validating Different Types of Assays
The specific type of positive control used will depend on the type of assay being validated. Here are some examples:
- PCR Assays: A known quantity of target DNA or RNA is used to ensure the PCR reagents and equipment are functioning correctly. The acceptance criteria usually include the presence of a band of the expected size and intensity.
- ELISA Assays: A purified antigen or antibody is used to verify that the assay can detect the target molecule. The acceptance criteria typically involve a specific range of absorbance values.
- Cell-Based Assays: A cell line known to respond to a particular stimulus is used to confirm that the assay can measure the desired cellular response. The acceptance criteria may include a specific change in cell viability, proliferation, or gene expression.
- Mass Spectrometry Assays: A known standard of the target analyte is used to calibrate the instrument and verify its ability to accurately quantify the analyte. Acceptance criteria often include the signal-to-noise ratio and the accuracy of the measured concentration.
In each of these examples, the positive control serves as an independent check on the assay's performance, helping to ensure the reliability and accuracy of the results.
Examples of Positive Controls in Different Scientific Fields
Assay validation provides confidence in our experimental results. But where do we see these principles put into practice? The utility of positive controls spans a multitude of scientific disciplines. Let's explore a few concrete examples across clinical diagnostics, pharmaceutical research, and environmental monitoring to illustrate their practical application.
Clinical Diagnostics: Ensuring Accurate Patient Results
In clinical diagnostics, accuracy is paramount. Patient lives depend on the reliable detection of diseases and conditions. Positive controls play a critical role in ensuring the validity of diagnostic tests.
COVID-19 PCR Testing
Consider the example of COVID-19 PCR (Polymerase Chain Reaction) testing.
A positive control in this scenario would consist of a sample containing a known quantity of the SARS-CoV-2 virus RNA.
If the PCR test fails to detect the viral RNA in the positive control sample, it indicates a problem with the reagents, equipment, or the testing procedure itself.
This failure would invalidate any negative results obtained from patient samples, as the test system's ability to detect the virus is compromised.
The positive control confirms that the PCR assay is working correctly, ensuring that true positive cases are not missed.
Pharmaceutical Research: Validating Drug Efficacy
Pharmaceutical research relies heavily on positive controls to validate drug screening assays and ensure the efficacy of potential therapeutic compounds.
Drug Screening Assays
In drug screening, researchers often use cell-based assays to identify compounds that can inhibit or activate a specific biological target.
A positive control in this context might be a known drug that is already established to have the desired effect on the target.
For example, if screening for inhibitors of a particular enzyme, a known enzyme inhibitor would serve as the positive control.
If the assay fails to show the expected inhibitory effect with the known drug, it suggests that the assay conditions are not optimal.
This could be due to issues with cell viability, reagent quality, or assay protocol.
By including a positive control, researchers can confirm that the assay is capable of detecting the desired effect, increasing confidence in the results obtained with novel compounds.
Environmental Monitoring: Safeguarding Public Health
Environmental monitoring utilizes positive controls to assess the quality of water, air, and soil samples, safeguarding public health and environmental integrity.
Testing Water Samples for Contaminants
Imagine testing water samples for the presence of harmful bacteria, such as E. coli.
A positive control would consist of a water sample spiked with a known concentration of E. coli bacteria.
If the test fails to detect the E. coli in the positive control sample, it indicates a problem with the testing procedure.
This could include issues with sample preparation, reagent contamination, or equipment malfunction.
The positive control ensures that the testing method is sensitive enough to detect the presence of the target contaminant, providing reliable data for environmental risk assessment and management.
Pharmaceutical research relies heavily on positive controls to validate drug screening assays and ensure the efficacy of potential therapeutic compounds. So, by incorporating positive controls into our experimental designs across these diverse fields, we greatly enhance the reliability and accuracy of our scientific endeavors. Now, let's pivot our focus to the crucial role of quality control in maintaining the integrity of laboratory testing.
Quality Control and the Positive Control: Maintaining Standards
Quality Control (QC) is the bedrock of any reliable laboratory operation. It's the systematic process of ensuring that analytical results are accurate, precise, and dependable. In essence, QC acts as a vigilant guardian, safeguarding the integrity of the entire testing process.
The Importance of Quality Control in Laboratory Testing
At its core, QC minimizes errors and variability.
It does so by establishing predefined standards and procedures. These standards govern everything from instrument calibration to reagent preparation and data analysis. By adhering to these rigorous protocols, laboratories can significantly reduce the risk of generating inaccurate or misleading results.
Moreover, QC programs are essential for regulatory compliance.
Many industries, such as healthcare and pharmaceuticals, are subject to strict regulatory oversight. These regulations often mandate the implementation of comprehensive QC programs to ensure the safety and efficacy of products and services.
Integrating Positive Controls into the QC Program
Positive controls are indispensable components of a robust QC program.
They serve as a benchmark for assessing the performance of the entire analytical system. By including positive controls in each analytical run, laboratories can verify that the test system is capable of detecting the target analyte or condition when it is present.
Specifically, consider the following integration points:
- Regular Inclusion: Positive controls should be included in every batch of samples analyzed. This provides ongoing monitoring of the test system's performance.
- Defined Acceptance Criteria: Clear acceptance criteria must be established for positive control results. These criteria should be based on the expected performance of the assay and should be documented in standard operating procedures (SOPs).
- Trend Analysis: The results of positive controls should be tracked over time to identify any trends or shifts in performance. This allows for early detection of potential problems before they impact patient results.
What to Do When a Positive Control Fails
Despite best efforts, positive controls can sometimes fail to perform as expected. When this occurs, it is crucial to have a predefined protocol for investigating and addressing the issue.
Here are the essential steps:
- Immediate Action: If a positive control fails, all patient results from that run should be considered suspect and should not be reported. The analytical run must be repeated.
- Troubleshooting: Investigate the potential causes of the failure. This may involve checking reagent integrity, instrument calibration, and procedural errors.
- Documentation: Thoroughly document the failure, the troubleshooting steps taken, and the corrective actions implemented. This documentation is essential for maintaining a complete audit trail.
- Corrective Action: Implement appropriate corrective actions to address the root cause of the failure. This may involve replacing reagents, recalibrating instruments, or revising procedures.
- Verification: Before resuming patient testing, verify that the corrective actions have resolved the problem and that the test system is performing as expected. This may involve running additional positive controls or performing proficiency testing.
In summary, quality control, with the positive control as a cornerstone, ensures the reliability of laboratory results. Adhering to robust QC programs is not merely a regulatory requirement; it's an ethical obligation to provide accurate and dependable information that informs critical decisions.
Video: Positive Control: Your Key To Valid Results Every Time!
FAQs: Understanding Positive Controls
[Positive controls are vital for reliable results. Here are some common questions answered:]
What exactly is a positive control?
A positive control is a test sample that you know should produce a positive result. It contains the substance or condition you're trying to detect in your experiment. It confirms that your experiment is working correctly.
Why do I need a positive control?
Without a positive control, a negative result in your experiment could mean a few things: your sample truly lacks what you're testing for, or your experiment simply failed. A positive control confirms that the reagents and procedures are working as expected.
What happens if my positive control doesn't work?
If the positive control fails to produce the expected positive result, it indicates a problem with the experimental setup. You'll need to troubleshoot the reagents, equipment, or procedure before proceeding. Your results are invalid until the positive control performs as it should.
How do I choose the right positive control?
Select a positive control that is as similar as possible to your test samples, but known to contain the target substance or condition. Consider the concentration of the target and make sure it's detectable by your assay. A well-chosen positive control is crucial for a valid experiment.