Oxygen's Freezing Point: The Chilling Truth You Need to Know
Understanding the freezing point of oxygen is crucial in several fields, from cryogenics research to industrial processes. Consider liquid oxygen, a vital component in rocket propulsion; its behavior is directly linked to the freezing point. The National Institute of Standards and Technology (NIST) provides the reference data for the freezing point of oxygen, ensuring accuracy across scientific applications. Furthermore, knowing the freezing point is fundamental when designing equipment that operates in extreme cold environments, where oxygen could solidify, impacting system functionality.
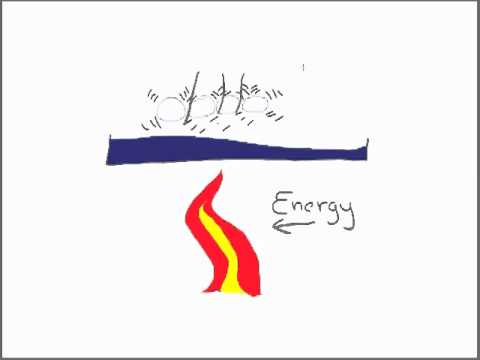
Image taken from the YouTube channel mrkscientific , from the video titled Boiling and Freezing Point .
Oxygen, the life-sustaining gas that makes up approximately 21% of Earth's atmosphere, is a cornerstone of our planet's ecosystems and numerous industrial processes.
It is an element so fundamental to our existence that its presence is often taken for granted.
However, oxygen's properties extend far beyond its gaseous form at room temperature.
Like all matter, oxygen can exist in solid, liquid, and gaseous states, each with unique characteristics and behaviors.
Understanding the freezing point of oxygen—the temperature at which it transitions from a liquid to a solid—is crucial for comprehending its fundamental nature and its applications in diverse fields.
Oxygen: A Vital Element
Oxygen plays an indispensable role in a wide range of processes, both natural and artificial.
In biology, it is essential for respiration in most living organisms, driving the metabolic processes that produce energy.
In industry, it is used extensively in steel production, welding, and chemical manufacturing.
Oxygen's reactive nature makes it a powerful oxidizing agent, facilitating combustion and other chemical reactions.
Its versatility has cemented its place as one of the most important elements in modern society.
The Significance of the Freezing Point
The freezing point of a substance is a fundamental physical property that reveals insights into the intermolecular forces that govern its state of matter.
It represents the temperature at which the kinetic energy of molecules is sufficiently reduced to allow attractive forces to dominate, causing the substance to transition from a disordered liquid state to an ordered solid state.
The freezing point is not merely a data point; it is a window into the microscopic world, revealing the delicate balance between energy and attraction that dictates the behavior of matter.
For oxygen, understanding its freezing point is critical for applications involving cryogenics, where extremely low temperatures are employed.
Exploring Oxygen's Frozen Frontier
This article embarks on a journey to explore the freezing point of oxygen in detail.
We will delve into its significance, examining the measurement scales used to quantify it and the related scientific principles, such as phase transitions, that govern its behavior.
We will also explore the practical applications of frozen and liquid oxygen in cryogenics and other industries, showcasing the transformative potential of matter at extreme temperatures.
Ultimately, this exploration will illuminate the fascinating intersection of science, technology, and the fundamental properties of one of the most vital elements on Earth.
The Science Behind Oxygen's Freezing Point and Phase Transition
Having established the foundational importance of oxygen and its freezing point, it's time to delve into the scientific underpinnings that govern this phenomenon. Understanding the physics and chemistry at play is crucial for appreciating the behavior of oxygen at cryogenic temperatures.
Defining the Freezing Point: A Molecular Perspective
In scientific terms, the freezing point is the temperature at which a substance transitions from a liquid to a solid state at a specific pressure (typically 1 atmosphere, or standard pressure). This transition isn't simply a matter of cooling; it's a result of changes in the balance between kinetic energy and intermolecular forces.
Kinetic energy is the energy of motion of the molecules. At higher temperatures, molecules possess greater kinetic energy, allowing them to move freely and overcome the attractive forces that hold them together.
As the temperature decreases, the kinetic energy of the molecules diminishes. Eventually, a point is reached where the intermolecular forces—such as Van der Waals forces or dipole-dipole interactions—become strong enough to overcome the disruptive effects of kinetic energy.
At the freezing point, these attractive forces lock the molecules into a relatively fixed, ordered arrangement, forming a solid.
For oxygen, a nonpolar molecule, the primary intermolecular force is the weak London dispersion force. This force arises from temporary fluctuations in electron distribution. Because these forces are relatively weak, oxygen's freezing point is quite low.
Phase Transitions of Oxygen: A Journey Through States of Matter
Oxygen's journey from a gas to a solid involves distinct phase transitions, each characterized by changes in energy and molecular arrangement.
At room temperature, oxygen exists as a gas (O₂). The molecules move freely and independently. As the gas is cooled, the molecules slow down.
When the temperature reaches approximately -183°C (-297°F or 90 K), oxygen undergoes a phase transition to become liquid oxygen (LOx).
At this point, the intermolecular forces are strong enough to hold the molecules closer together, but they can still move and slide past one another.
Further cooling of liquid oxygen leads to another phase transition at its freezing point, approximately -218.79°C (-361.82°F or 54.36 K).
Below this temperature, oxygen solidifies, forming solid oxygen. In its solid state, oxygen molecules are locked into a crystalline structure, with minimal movement. The molecules vibrate in place, but no longer move freely.
Each phase transition is associated with a change in enthalpy (heat content). The transition from gas to liquid releases energy (exothermic), while the transition from liquid to solid also releases energy.
The Influence of Pressure: Navigating the Phase Diagram
The freezing point of oxygen, like that of any substance, is not a fixed value, but is influenced by pressure. This relationship is best represented by a phase diagram, which illustrates the stable phases of a substance at different temperatures and pressures.
The phase diagram of oxygen shows that increasing the pressure generally increases the freezing point.
This is because higher pressure forces the molecules closer together, making it easier for intermolecular forces to dominate and solidify the substance.
However, the effect of pressure on the freezing point of oxygen is relatively small compared to its effect on the boiling point. This is because liquids and solids are already quite dense and less compressible than gases.
At extremely high pressures, oxygen can exhibit different solid phases with distinct crystal structures. These phases have varying densities and stabilities.
Understanding the interplay between temperature and pressure is crucial in many applications. It is especially important where oxygen is stored and used at cryogenic temperatures.
Having explored the scientific principles governing oxygen's transformation into solid form, we now turn our attention to the methods we use to quantify these extreme temperatures. After all, understanding the freezing point is only useful if we can accurately measure and express it.
Measuring Extreme Cold: Temperature Scales and Oxygen's Freezing Point
The freezing point of oxygen, a critical benchmark in cryogenics, isn't a universal, unitless number. Its value depends entirely on the temperature scale used for measurement. Understanding these scales and their relationship to each other is fundamental to working with cryogenic substances like oxygen.
Oxygen's Freezing Point Across Different Scales
The freezing point of oxygen is expressed differently depending on whether we're using Celsius, Kelvin, or Fahrenheit.
-
Celsius (°C): On the Celsius scale, oxygen freezes at approximately -218.79 °C. This scale, defined by the freezing and boiling points of water at 0 °C and 100 °C respectively, is commonly used in many parts of the world for everyday temperature measurements.
-
Kelvin (K): The Kelvin scale, an absolute thermodynamic temperature scale, sets its zero point at absolute zero, the theoretical absence of all thermal energy. Oxygen freezes at 54.36 K. Because it begins at absolute zero, Kelvin is especially useful in scientific contexts for its direct correlation to molecular kinetic energy.
-
Fahrenheit (°F): Predominantly used in the United States, the Fahrenheit scale places the freezing point of water at 32 °F and the boiling point at 212 °F. Oxygen freezes at -361.82 °F on this scale.
Temperature Scale Conversions: Bridging the Gaps
Converting between these temperature scales is essential for communicating and comparing data across different fields and regions. The following formulas are used for these conversions:
- Celsius to Kelvin: K = °C + 273.15
- Kelvin to Celsius: °C = K - 273.15
- Celsius to Fahrenheit: °F = (°C
**9/5) + 32
- Fahrenheit to Celsius: °C = (°F - 32)** 5/9
- Fahrenheit to Kelvin: K = (°F + 459.67)
**5/9
- Kelvin to Fahrenheit: °F = (K** 9/5) - 459.67
These conversions are crucial when working with data from different sources or when applying scientific principles that are scale-dependent.
The Importance of Kelvin: Absolute Zero and Scientific Accuracy
While Celsius and Fahrenheit are useful for everyday measurements, Kelvin is the preferred scale in scientific contexts for several key reasons:
-
Absolute Zero: The Kelvin scale's absolute zero point eliminates negative temperatures, simplifying many scientific calculations, especially those involving thermodynamics.
-
Direct Proportionality to Energy: Temperature in Kelvin is directly proportional to the average kinetic energy of molecules. This direct relationship simplifies understanding and modeling physical phenomena at the molecular level.
-
Thermodynamic Calculations: Many thermodynamic equations and principles are based on absolute temperature, making Kelvin the most suitable scale for these applications.
In essence, the Kelvin scale provides a more fundamental and consistent framework for understanding and quantifying temperature, especially in the realm of cryogenics where temperatures approach absolute zero. It's the gold standard for scientific accuracy and consistency in measuring extreme cold.
Having explored the scientific principles governing oxygen's transformation into solid form, we now turn our attention to the methods we use to quantify these extreme temperatures. After all, understanding the freezing point is only useful if we can accurately measure and express it.
The Triple Point of Oxygen: A Thermodynamic Crossroads
Beyond simply freezing, substances exhibit a range of behaviors dictated by temperature and pressure. One of the most fascinating of these is the triple point, a state where solid, liquid, and gaseous phases exist simultaneously. Understanding this point for oxygen provides invaluable insight into its thermodynamic properties.
Defining the Elusive Triple Point
The triple point is a unique set of conditions – a precise temperature and pressure – at which a substance can stably exist in all three phases of matter: solid, liquid, and gas.
This isn't a chaotic jumble of phases, but rather a state of thermodynamic equilibrium, where the rates of transition between the phases are equal. It represents a fundamental characteristic of a substance.
Think of it as a crossroads on a phase diagram, the unique intersection where the sublimation curve, fusion curve, and vaporization curve all meet.
Oxygen's Triple Point: A Calibration Standard
For oxygen, the triple point occurs at approximately 54.36 K (-218.79 °C) and a pressure of 0.15 kPa (1.13 Torr).
These meticulously determined values aren't just academic curiosities; they serve a crucial purpose in calibrating scientific instruments, especially thermometers and pressure gauges used in cryogenic research.
Why the Triple Point is Ideal for Calibration
The triple point's defining characteristic – its fixed temperature and pressure – makes it an ideal reference point.
Because it is an intrinsic property of oxygen, it is reproducible anywhere in the world, regardless of external conditions.
By precisely defining these instruments against the known triple point of oxygen, scientists can ensure the accuracy and consistency of their measurements, leading to reliable experimental results.
Furthermore, it's more precise than using melting points or boiling points which are more susceptible to variations in atmospheric pressure.
In conclusion, the triple point of oxygen isn't just a peculiar phenomenon; it's a cornerstone of accurate measurement in low-temperature physics, enabling progress in fields ranging from materials science to space exploration.
Having established oxygen's triple point as a cornerstone of low-temperature calibration, it's important to acknowledge the pioneers who first ventured into this realm of extreme cold. Their work laid the foundation for our current understanding and technological capabilities.
A Pioneer in Cryogenics: Kamerlingh Onnes and the Solidification of Oxygen
Heike Kamerlingh Onnes wasn't just a scientist; he was an explorer of the ultra-cold. His meticulous work not only earned him the Nobel Prize in Physics in 1913 but also ushered in the age of cryogenics, forever changing our understanding of matter at its coldest extremes. He was a true pioneer, venturing into uncharted scientific territory.
Onnes's Groundbreaking Contributions and Nobel Recognition
Kamerlingh Onnes established a world-class low-temperature laboratory at Leiden University. His dedication to precision and innovation was unparalleled. He saw the potential of low-temperature research, recognizing its importance long before its practical applications became widespread.
His liquefaction of helium in 1908 was a monumental achievement. It provided scientists with access to temperatures previously unattainable. This opened up entirely new avenues of research into superconductivity and the behavior of matter near absolute zero. This work formed the basis of his Nobel Prize.
The 1913 Nobel Prize in Physics recognized Kamerlingh Onnes "for his investigations on the properties of matter at low temperatures which led, inter alia, to the production of liquid helium." But beyond the liquid helium, his contributions were far-reaching. They provided a foundation for all subsequent cryogenic research.
The Quest to Solidify Oxygen: Challenges and Breakthroughs
Before Onnes, the solidification of oxygen remained elusive. Scientists had theorized about its existence in solid form, but actually achieving it presented immense technical challenges. Reaching the necessary temperatures and maintaining them consistently was a significant hurdle.
Onnes's meticulous experiments built upon earlier work to liquefy oxygen. He then ingeniously decreased the temperature even further using advanced cooling techniques. By carefully controlling the temperature and pressure, he successfully solidified oxygen, confirming its existence in a solid state.
The experiments weren't without their difficulties. The extremely low temperatures required posed significant technical hurdles. Maintaining stable conditions and accurately measuring properties at these temperatures demanded innovative solutions.
Onnes meticulously documented his work, providing a detailed account of his methods and observations. His published papers became invaluable resources for other scientists. They outlined both the successes and the challenges encountered during the oxygen solidification experiments.
The solidification of oxygen was a crucial step in validating the theoretical understanding of phase transitions. It offered invaluable empirical data. It allowed scientists to probe the behavior of matter at the quantum level. This cemented Onnes's place as a father of cryogenics. His work extended far beyond a single element, establishing a foundation for the modern study of low-temperature physics.
Practical Applications of Liquid Oxygen: Leveraging Extreme Cold
Having charted the course of oxygen's solidification and the pioneering work that unlocked its cryogenic potential, we now turn our attention to the tangible benefits this extreme cold offers across various sectors. Liquid oxygen, a substance once confined to the realm of theoretical physics, has become an indispensable tool, driving advancements in medicine, industry, and even space exploration.
Liquid Oxygen in Medicine: Life Support and Preservation
Liquid oxygen plays a critical role in modern medicine, supporting life and pushing the boundaries of medical possibility.
Its most well-known application is in respiratory therapy, where it provides supplemental oxygen to patients with breathing difficulties. Portable liquid oxygen systems offer greater autonomy and longer usage times compared to compressed gas cylinders, improving the quality of life for individuals with chronic respiratory conditions.
Beyond respiratory support, liquid oxygen is essential for cryopreservation, the process of preserving biological tissues, cells, and even organs at ultra-low temperatures. This allows for long-term storage of samples for research, fertility treatments, and potential future transplantation. The ability to halt biological processes through extreme cold holds immense promise for regenerative medicine and extending human lifespan.
Industrial Applications: Powering Steel and Beyond
The industrial sector heavily relies on liquid oxygen for a range of processes, capitalizing on its powerful oxidizing properties and cryogenic capabilities.
In steel production, liquid oxygen is used to increase the efficiency of blast furnaces, accelerating the combustion of carbon and impurities. This results in higher quality steel produced at a faster rate, driving down costs and improving productivity.
Welding is another area where liquid oxygen is invaluable. When combined with a fuel gas like acetylene, it produces an intensely hot flame capable of melting and fusing metals together. This process is crucial in construction, manufacturing, and repair work.
Liquid Oxygen as Rocket Propellant: Fueling Space Exploration
Perhaps the most awe-inspiring application of liquid oxygen lies in its use as a rocket propellant.
As a powerful oxidizer, it enables the rapid combustion of fuel, generating the thrust needed to propel rockets into space. The high energy density of liquid oxygen makes it an ideal choice for powering spacecraft, allowing them to overcome Earth's gravity and explore the cosmos.
Liquid oxygen's role in space exploration is undeniable. From launching satellites to enabling crewed missions to the Moon and beyond, it is a critical component of our efforts to reach for the stars.
The Crucial Role of Cryogenics in Handling Liquid Oxygen
The safe and effective utilization of liquid oxygen hinges on the principles and practices of cryogenics.
Cryogenics, the science of very low temperatures, encompasses the techniques and technologies required to handle, store, and transport cryogenic fluids like liquid oxygen.
Specialized equipment is essential for working with liquid oxygen. This includes insulated storage tanks, transfer lines, and pumps designed to minimize heat leak and prevent vaporization.
Insulation techniques are also critical. Vacuum insulation, multi-layer insulation, and other advanced materials are used to create a thermal barrier between the liquid oxygen and the surrounding environment, reducing boil-off and maintaining the liquid's cryogenic state.
Proper training and safety protocols are paramount when handling liquid oxygen due to the risks associated with extreme cold and its oxidizing properties. Adherence to these guidelines ensures the safe and responsible use of this valuable resource.
Video: Oxygen's Freezing Point: The Chilling Truth You Need to Know
FAQs: Oxygen's Freezing Point
Here are some frequently asked questions to further clarify the freezing point of oxygen and its implications.
At what temperature does oxygen freeze?
Oxygen freezes at approximately -218.79 degrees Celsius (-361.82 degrees Fahrenheit). This extremely low temperature highlights the strong intermolecular forces needed to transition oxygen from a liquid to a solid state.
Why is the freezing point of oxygen so low?
The freezing point of oxygen is low because of the weak van der Waals forces between oxygen molecules. These forces are relatively easy to overcome, requiring a very low temperature for oxygen to solidify.
Is it possible to freeze oxygen in everyday life?
No, it is not possible to freeze oxygen under typical everyday conditions. The extremely low temperature required is far below what household freezers or most industrial freezers can achieve. Freezing oxygen requires specialized equipment and cryogenic cooling techniques.
What are some practical uses of liquid or frozen oxygen despite its extremely low freezing point?
Despite the challenge of maintaining the extreme cold, liquid and sometimes solidified oxygen have practical uses in various industries. These include rocket propellant, medical applications such as respiratory support, and industrial processes requiring extremely low temperatures.
So, there you have it – the chilling truth about the freezing point of oxygen! Hopefully, you found this helpful. Feel free to share this article if you did! Now you know a bit more about why understanding freezing point of oxygen is essential in various scenarios.