Ice's Secret: Unlocking the Specific Heat of Ice Mystery
Understanding the behavior of water in its solid form necessitates examining its thermal properties. Calorimetry, a crucial measurement technique, directly relates to the specific heat of ice, an essential value in thermodynamics. Changes in the phase of water, such as melting, profoundly affect glacier dynamics and sea levels. The Joule (J), a standard unit of energy, quantifies the amount of heat needed to alter the temperature of a substance, including ice. Therefore, this article explores the specific heat of ice, unraveling the complex interplay between energy, temperature, and the physical state of water.
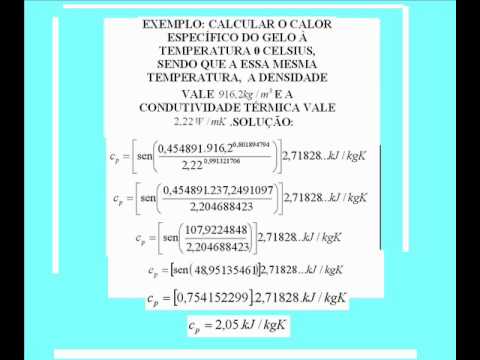
Image taken from the YouTube channel PROF CÉSAR MONTEIRO DE BARROS , from the video titled The specific heat of ice as a function of thermal conductivity and density. .
Imagine stepping onto a frozen lake. The air is frigid, yet beneath your skates, the water remains liquid, supporting the ice above. This seemingly simple observation hints at a complex interplay of thermal properties, where specific heat capacity plays a pivotal role. Understanding this concept is crucial to grasping how materials respond to heat, and ice, with its unique characteristics, offers a fascinating case study.
The Significance of Specific Heat Capacity
At its core, specific heat capacity is a measure of a substance's ability to absorb heat energy without undergoing drastic temperature changes. It quantifies the amount of heat required to raise the temperature of one gram of a substance by one degree Celsius (or one Kelvin). This property dictates how readily a material heats up or cools down in response to its surroundings.
A substance with a high specific heat capacity requires a significant amount of energy to alter its temperature. Conversely, a substance with a low specific heat capacity will experience a more pronounced temperature change for the same amount of heat input. This fundamental difference dictates the thermal behavior of everything around us.
Specific Heat of Ice: A Unique Case
Ice, the solid form of water, possesses a specific heat capacity that is distinct from liquid water and other materials. Understanding the specific heat of ice is paramount in various scientific and practical applications. From predicting the rate of glacial melting to designing effective cooling systems, the thermal properties of ice are of considerable importance.
The specific heat of ice influences a wide range of phenomena, including weather patterns, climate regulation, and even the preservation of food. Its value is crucial for accurate climate modeling and predicting the behavior of frozen environments. Moreover, understanding the specific heat of ice helps in optimizing industrial processes involving freezing and thawing.
Hook: The Curious Case of Freezing Lakes
Consider the phenomenon of lakes freezing over in winter. Despite prolonged exposure to sub-zero temperatures, the entire lake does not solidify instantly. Instead, a layer of ice forms on the surface, insulating the water below and allowing aquatic life to survive.
This is due, in part, to water's relatively high specific heat capacity compared to other common substances. However, the specific heat of ice itself also plays a crucial role. As the surface water cools and freezes, the heat released during the phase transition warms the water below, slowing down the freezing process. This intricate balance highlights the real-world significance of understanding the specific heat capacity of ice.
Imagine stepping onto a frozen lake. The air is frigid, yet beneath your skates, the water remains liquid, supporting the ice above. This seemingly simple observation hints at a complex interplay of thermal properties, where specific heat capacity plays a pivotal role. Understanding this concept is crucial to grasping how materials respond to heat, and ice, with its unique characteristics, offers a fascinating case study.
But before we delve deeper into the intricacies of ice, let's first solidify our understanding of the foundational principle at play: specific heat capacity itself. What exactly does this term mean, and why is it so fundamental to how the world around us functions?
Demystifying Specific Heat Capacity
At its heart, specific heat capacity is a measure of a substance's ability to absorb heat energy without undergoing drastic temperature changes. It's a quantitative representation of how much energy is required to nudge the temperature of a substance. More precisely, it's defined as the amount of heat needed to raise the temperature of one gram of a substance by one degree Celsius (or one Kelvin). This property dictates how readily a material heats up or cools down in response to its surroundings.
A substance boasting a high specific heat capacity needs a significant infusion of energy to alter its temperature. Conversely, a substance with a low specific heat capacity will experience a more pronounced temperature change for the same amount of heat input. This fundamental difference dictates the thermal behavior of everything around us.
The Dance of Heat Transfer
Heat transfer is the process by which thermal energy moves from one place to another. It occurs through three primary mechanisms: conduction, convection, and radiation. The specific heat capacity of a substance directly influences how effectively it can absorb or release heat during these processes.
For example, materials with high specific heat capacities, like water, can absorb large amounts of heat without experiencing a significant temperature increase. This makes them excellent coolants, as they can efficiently carry heat away from a source. Conversely, materials with low specific heat capacities heat up quickly, making them suitable for applications where rapid temperature changes are desired.
A World of Thermal Diversity
The specific heat capacity is far from a universal constant; it varies significantly from one substance to another. This variance stems from the unique molecular structure and bonding characteristics of each material. For instance, metals generally have low specific heat capacities due to their tightly packed atomic structure and free electrons, which readily conduct heat. This explains why a metal spoon heats up quickly when placed in a hot beverage.
In contrast, substances like water and ammonia possess high specific heat capacities due to their strong intermolecular forces, such as hydrogen bonds. These bonds require significant energy to break or stretch, resulting in a greater capacity to absorb heat without a substantial temperature increase. The nature of the substance dictates its thermal response.
Energy's Role in Temperature Change
At the molecular level, temperature is directly proportional to the average kinetic energy of the molecules within a substance. Introducing heat energy into a system increases this kinetic energy, causing the molecules to move faster and collide more frequently.
The specific heat capacity determines how efficiently this added energy translates into a temperature increase. A substance with a high specific heat capacity will require more energy to achieve the same temperature change as a substance with a low specific heat capacity. This highlights the crucial relationship between energy input, specific heat capacity, and the resulting temperature change within a material.
Imagine stepping onto a frozen lake. The air is frigid, yet beneath your skates, the water remains liquid, supporting the ice above. This seemingly simple observation hints at a complex interplay of thermal properties, where specific heat capacity plays a pivotal role. Understanding this concept is crucial to grasping how materials respond to heat, and ice, with its unique characteristics, offers a fascinating case study.
But before we delve deeper into the intricacies of ice, let's first solidify our understanding of the foundational principle at play: specific heat capacity itself. What exactly does this term mean, and why is it so fundamental to how the world around us functions?
Demystifying Specific Heat Capacity
At its heart, specific heat capacity is a measure of a substance's ability to absorb heat energy without undergoing drastic temperature changes.
It's a quantitative representation of how much energy is required to nudge the temperature of a substance.
More precisely, it's defined as the amount of heat needed to raise the temperature of one gram of a substance by one degree Celsius (or one Kelvin).
This property dictates how readily a material heats up or cools down in response to its surroundings.
A substance boasting a high specific heat capacity needs a significant infusion of energy to alter its temperature.
Conversely, a substance with a low specific heat capacity will experience a more pronounced temperature change for the same amount of heat input.
This fundamental difference dictates the thermal behavior of everything around us.
The dance between energy and temperature change is unique to each substance, and now it's time to consider the unique case of ice.
Ice's Unique Thermal Fingerprint: A Deep Dive
Ice, in its crystalline glory, presents a thermal profile unlike any other substance. Its unique specific heat capacity stems from its molecular architecture, a structure governed by the fascinating phenomenon of hydrogen bonding.
The Molecular Structure of Ice: A Foundation for Thermal Properties
The water molecule (H₂O) is simple in its composition, yet complex in its interactions.
In its liquid state, water molecules are in constant motion, forming and breaking bonds with their neighbors.
However, as water cools and transitions into ice, these molecules settle into a highly ordered, crystalline lattice structure.
This lattice isn't just any arrangement; it's a tetrahedral structure, where each oxygen atom is connected to four hydrogen atoms – two covalently bonded within the molecule and two through hydrogen bonds with neighboring molecules.
This organized arrangement is the very foundation that governs ice's unique thermal properties.
The Significance of Hydrogen Bonding
Hydrogen bonds are the unsung heroes behind many of water's peculiar characteristics, and its specific heat capacity is no exception.
These relatively weak, yet numerous, bonds demand energy to be stretched or broken.
When heat is applied to ice, a significant portion of that energy goes into vibrating and manipulating these hydrogen bonds, rather than directly increasing the kinetic energy of the water molecules (which would manifest as a temperature increase).
Think of it like this: the hydrogen bonds act as tiny shock absorbers, buffering the impact of added heat.
This absorption of energy by the hydrogen bonds contributes significantly to ice's specific heat capacity, allowing it to absorb a considerable amount of heat before its temperature rises substantially.
It's important to note that the specific heat capacity of ice varies with temperature. As ice approaches its melting point, the specific heat increases. This is due to the increased vibrational energy within the crystal lattice, which softens the structure and prepares it for the phase transition to liquid water.
Density and Specific Heat: An Intertwined Relationship
The open, tetrahedral structure created by hydrogen bonding also explains another unusual property of ice: its density.
Unlike most substances, ice is less dense than its liquid form.
This is because the rigid crystalline structure of ice forces the water molecules to be further apart than they are in liquid water, where they can pack more closely together.
This lower density impacts its thermal behavior.
Because ice is less dense, there are fewer molecules per unit volume compared to water.
However, the specific heat capacity is defined per unit mass.
Therefore, even though the hydrogen bonding influences both density and specific heat, the relationship isn't a simple, direct correlation.
The complex interplay of hydrogen bonding, molecular arrangement, and vibrational energy dictates that ice can absorb a substantial amount of heat energy for a relatively small temperature change, making its thermal fingerprint truly unique.
The dance between specific heat capacity and a substance's inherent properties sets the stage for a truly fascinating phenomenon: phase transition. When we apply heat to ice, we're not just raising its temperature; we're initiating a fundamental change in its very state of matter. This transformation, governed by the laws of thermodynamics, unveils the intricate relationship between heat, energy, and the molecular structure of ice.
Thermodynamics and the Transformation of Ice
Thermodynamics provides the framework for understanding how energy dictates the behavior of ice as it responds to heat. The principles of thermodynamics, especially the First Law (conservation of energy), dictate that any heat added to ice must either increase its internal energy (raising its temperature) or be used to perform work (changing its phase).
Understanding how ice behaves when heat is applied involves understanding thermodynamic principles.
The Phase Transition: Ice to Water
The most apparent transformation occurs when ice melts into water.
This phase transition is not merely a change in appearance; it's a fundamental shift in the arrangement and energy of the water molecules.
Ice, in its solid state, possesses a highly ordered crystalline structure held together by hydrogen bonds.
As heat is applied, these bonds begin to weaken, allowing the molecules to move more freely.
At the melting point, the ice absorbs enough energy to break free from its rigid structure entirely, transitioning into the liquid state of water.
Energy Requirements: Specific Heat vs. Latent Heat
The energy required to transform ice into water is not solely determined by its specific heat capacity.
Two key concepts govern the energy needed: specific heat and latent heat.
Specific heat dictates the energy required to raise the temperature of ice before it reaches its melting point.
Once at 0°C (32°F), further heat input doesn't increase the temperature but instead fuels the phase change.
This energy, used to break the intermolecular bonds and convert the ice to water, is known as the latent heat of fusion.
Latent heat explains why ice at 0°C feels much colder than water at 0°C; the ice is still absorbing heat to melt.
The Role of Specific and Latent Heat
Specific heat and latent heat work in tandem during the melting process.
First, specific heat increases the ice's temperature to its melting point.
Then, latent heat takes over, providing the energy for the phase transition without any further temperature increase.
Only after all the ice has melted will the specific heat of water come into play, determining how the water's temperature rises with further heat input.
This two-stage process highlights the complex energy dynamics involved in phase transitions.
Melting Point: A Thermodynamic Threshold
The melting point is a critical thermodynamic property of ice.
It represents the temperature at which the solid and liquid phases can coexist in equilibrium.
At this specific temperature (0°C or 32°F under standard conditions), the rate of melting equals the rate of freezing, resulting in a dynamic balance.
The melting point is influenced by factors like pressure and the presence of impurities.
The specific heat of ice prior to reaching the melting point determines how quickly it reaches this crucial thermodynamic threshold.
Ultimately, the thermodynamics governing ice's behavior when heated underscores the intricate interplay between energy, molecular structure, and the resulting phase transitions. Understanding these principles is fundamental to comprehending the broader thermal behavior of matter and its implications across various scientific and practical domains.
The energy required to transform ice into water is not solely dictated by the substance's specific heat; the latent heat of fusion also plays a crucial role. To truly understand the thermal dance of ice, we need a method for quantifying these properties directly. This is where the science of calorimetry enters the stage, providing the tools and techniques to measure the specific heat capacity of ice with precision.
Measuring Ice's Heat Capacity: The Science of Calorimetry
Calorimetry is the science of measuring heat transfer during physical and chemical processes. It allows scientists and engineers to quantify the amount of heat absorbed or released by a substance, providing critical data for understanding its thermal behavior. In the context of ice, calorimetry is essential for determining its specific heat capacity and understanding how it responds to changes in temperature.
Calorimetry: Quantifying Heat Transfer
At its core, calorimetry relies on the principle of energy conservation. A calorimeter is essentially an insulated container designed to minimize heat exchange with the surroundings. By carefully measuring the temperature changes within the calorimeter when a substance like ice is introduced, scientists can calculate the amount of heat transferred.
This calculation is based on the equation:
Q = mcΔT
Where:
- Q represents the heat transferred,
- m is the mass of the substance,
- c is the specific heat capacity,
- and ΔT is the change in temperature.
Experimental Techniques for Ice
Determining the specific heat of ice requires careful experimental design and execution. Several techniques can be employed, each with its own advantages and limitations.
The Method of Mixtures
One common approach is the method of mixtures. This involves adding a known mass of ice at a known temperature to a calorimeter containing a known mass of water at a different temperature. The final temperature of the mixture is then carefully measured.
By applying the principle of energy conservation (heat lost by the water equals heat gained by the ice), the specific heat of ice can be calculated.
This method requires accurate measurements of mass and temperature, as well as careful consideration of heat losses to the surroundings.
Differential Scanning Calorimetry (DSC)
Another powerful technique is Differential Scanning Calorimetry (DSC). DSC measures the heat flow required to maintain a substance and a reference material at the same temperature as they are subjected to a controlled temperature program.
By analyzing the heat flow data, the specific heat capacity of ice can be determined as a function of temperature. DSC is particularly useful for studying phase transitions, such as melting, and for identifying subtle changes in the thermal properties of ice.
Considerations for Accurate Measurement
Regardless of the technique used, accurate determination of the specific heat of ice requires careful attention to several factors.
-
Thermal equilibrium: Ensuring that the ice and the surrounding medium have reached thermal equilibrium is crucial for accurate measurements.
-
Heat losses: Minimizing heat losses to the surroundings is essential for preventing errors in the calculation of heat transfer.
-
Purity of ice: The purity of the ice sample can also affect the measured specific heat capacity. Impurities can alter the thermal properties of ice and lead to inaccurate results.
By carefully controlling these factors and employing appropriate experimental techniques, scientists can obtain reliable and accurate measurements of the specific heat of ice, contributing to a deeper understanding of its thermal behavior.
The experiments and calculations we've explored reveal the specific heat capacity of ice, but the story doesn't end there. To fully appreciate the thermal behavior of ice, it's crucial to compare it with its liquid counterpart: water.
Ice vs. Water: A Tale of Two Specific Heats
The contrast between the specific heat of ice and that of liquid water is striking. Ice possesses a specific heat of approximately 2.05 J/g°C, while water boasts a significantly higher value of around 4.18 J/g°C. This disparity means that it takes more than twice as much energy to raise the temperature of a given mass of water by one degree Celsius compared to ice.
Molecular Basis for the Difference
The reason for this difference lies in the molecular structure and bonding characteristics of the two phases. In ice, water molecules are arranged in a rigid, crystalline lattice held together by hydrogen bonds. When heat is applied, much of the energy goes into breaking or weakening these hydrogen bonds, allowing the molecules to vibrate more vigorously, but not necessarily increasing their kinetic energy (temperature) to the same extent as in water.
In liquid water, although hydrogen bonds are still present, they are more flexible and dynamic. Water molecules can move more freely, rotating and translating in addition to vibrating. This increased freedom of movement allows water to absorb more energy as kinetic energy, resulting in a higher temperature increase for the same amount of heat input.
Environmental and Practical Implications
The higher specific heat of water has profound environmental implications. Oceans and large bodies of water act as massive heat reservoirs, moderating global temperatures. They absorb vast amounts of solar energy during the day and release it slowly at night, preventing extreme temperature fluctuations. This is why coastal regions tend to have milder climates compared to inland areas.
The high specific heat of water is also crucial for aquatic life. The slow temperature changes in water provide a stable environment for aquatic organisms, protecting them from sudden temperature shocks.
In contrast, the lower specific heat of ice makes it useful in certain practical applications. Ice can be used as a cooling agent because it absorbs heat as it melts, but its lower specific heat means it warms up more quickly than water if not undergoing a phase change. This difference is considered in everything from designing effective ice packs to understanding the behavior of glaciers.
The Role of Hydrogen Bonds
Ice's Structure
The hydrogen bonds in ice form a highly ordered, three-dimensional network. This structure restricts the movement of water molecules and requires energy to disrupt when heated.
Water's Flexibility
In liquid water, hydrogen bonds are constantly breaking and reforming, allowing water molecules more freedom of movement. This dynamic arrangement enables water to absorb more energy as kinetic energy, hence the higher specific heat.
The differing specific heats of ice and water are not merely numbers; they are key determinants of our planet's climate and play a crucial role in numerous everyday applications. Understanding these differences provides a deeper appreciation for the unique properties of water and its various phases.
The contrast between the specific heat of ice and that of liquid water is striking. Ice possesses a specific heat of approximately 2.05 J/g°C, while water boasts a significantly higher value of around 4.18 J/g°C. This disparity means that it takes more than twice as much energy to raise the temperature of a given mass of water by one degree Celsius compared to ice. Understanding these thermal properties allows scientists and engineers to harness the unique characteristics of ice for a wide range of applications.
Real-World Applications: Harnessing the Specific Heat of Ice
The specific heat of ice, a seemingly simple physical property, underpins a surprising array of technologies and natural phenomena. From regulating the Earth's climate to preserving our food, the ability of ice to absorb a significant amount of heat with a relatively small temperature change is invaluable.
Climate Science: Ice's Role in Global Climate Regulation
The presence of ice, whether in the form of glaciers, ice sheets, or sea ice, has a profound impact on global climate patterns. Ice acts as a massive thermal reservoir, absorbing heat during melting and releasing it during freezing.
This buffering effect moderates temperature fluctuations, particularly in polar regions. The slow melting of glaciers provides a steady source of freshwater, while the extent of sea ice influences ocean currents and atmospheric circulation.
Changes in ice cover, driven by rising global temperatures, directly impact the Earth’s albedo, or reflectivity. As ice melts, darker surfaces like water or land are exposed, absorbing more solar radiation and accelerating warming—a dangerous feedback loop.
Understanding the specific heat of ice is, therefore, crucial for accurately modeling climate change and predicting its consequences. Climate models rely on precise knowledge of ice's thermal properties to simulate the complex interactions within the Earth's climate system.
Engineering Applications: Cooling, Storage, and Insulation
The high specific heat of ice makes it an ideal material for various engineering applications, particularly those involving cooling and thermal energy storage.
Cooling Systems and Ice Storage
Ice-based cooling systems are used in a variety of settings, from industrial processes to air conditioning. These systems take advantage of the large amount of energy required to melt ice, providing efficient and cost-effective cooling.
Ice storage systems, for example, can generate and store ice during off-peak hours when electricity is cheaper, and then use the ice to provide cooling during peak demand periods. This reduces energy consumption and lowers electricity costs.
Insulation Materials
The thermal properties of ice also influence the design of insulation materials. While ice itself isn't used as direct insulation, understanding its specific heat helps engineers develop materials that can effectively slow down heat transfer.
For example, the formation of ice within porous materials can significantly alter their thermal conductivity, impacting their ability to insulate buildings or equipment.
Food Preservation: Keeping Things Cool
One of the most familiar applications of ice is in food preservation. The high specific heat of ice allows it to absorb a significant amount of heat from its surroundings, keeping food cold and preventing spoilage.
By maintaining low temperatures, ice slows down the growth of bacteria and other microorganisms that cause food to decay.
This principle is used in a wide range of applications, from simple iceboxes to sophisticated refrigerated transport systems. The effectiveness of ice in preserving food depends on factors such as the amount of ice used, its surface area, and the insulation of the container.
Video: Ice's Secret: Unlocking the Specific Heat of Ice Mystery
FAQs: Decoding Ice's Specific Heat Mystery
Here are some frequently asked questions to help you better understand the specific heat of ice and its implications.
What exactly is specific heat?
Specific heat is the amount of heat energy needed to raise the temperature of one gram of a substance by one degree Celsius. Different materials require different amounts of energy to heat up; water, for example, has a high specific heat.
How does the specific heat of ice differ from liquid water?
The specific heat of ice is about half that of liquid water. This means it takes roughly half the energy to raise the temperature of ice by one degree compared to raising the temperature of the same amount of liquid water.
Why is understanding the specific heat of ice important?
Knowing the specific heat of ice helps us predict and understand how ice responds to temperature changes. This is crucial for various applications, from climate modeling and food preservation to understanding glacial melt rates.
Does the specific heat of ice change with temperature?
Yes, the specific heat of ice isn't constant across all temperatures. It varies slightly, generally increasing as the ice approaches its melting point. This variation is considered in precise scientific calculations.
And there you have it! Hopefully, you now have a better grasp on the specific heat of ice and how it impacts everything from your frozen drinks to the planet's climate. Keep exploring, and stay curious!