Why is the Sky Blue? Rayleigh Scattering Explained
The daytime sky exhibits a captivating blue hue because of a phenomenon known as Rayleigh scattering, a principle first comprehensively explained by British physicist Lord Rayleigh. Sunlight, a form of electromagnetic radiation composed of various wavelengths, interacts with the Earth's atmosphere, a gaseous envelope primarily constituted of nitrogen and oxygen molecules. These atmospheric molecules, functioning as scattering particles, preferentially scatter shorter wavelengths of light, specifically blue and violet, more efficiently than longer wavelengths, such as red and orange; this wavelength-dependent scattering explains why is the sky blue rayleigh scattering. The intensity of this scattering is quantified by the Rayleigh scattering equation, demonstrating an inverse fourth-power relationship between scattering intensity and wavelength, thus solidifying the understanding of atmospheric optics.
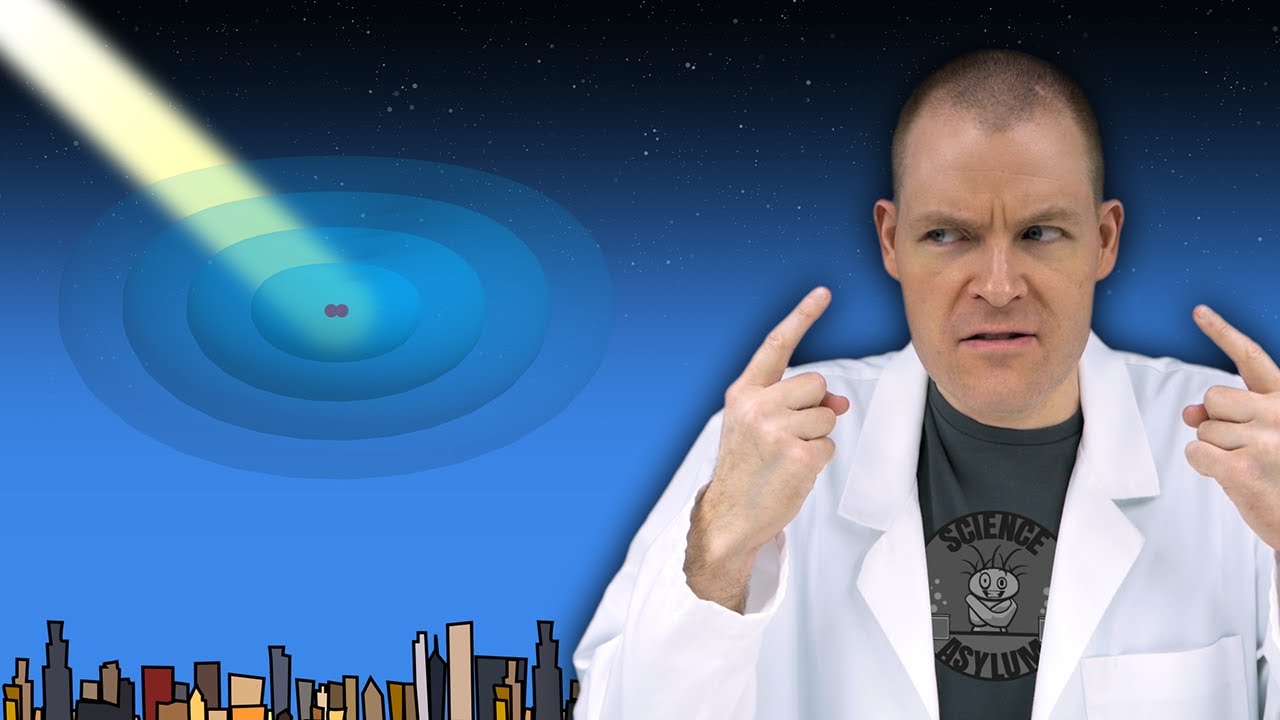
Image taken from the YouTube channel The Science Asylum , from the video titled How Does Rayleigh Scattering ACTUALLY Work? (The Blue Sky) .
Unveiling the Secrets of Rayleigh Scattering
The cerulean hue of the daytime sky is a vista so familiar, so commonplace, that we rarely pause to consider its origins. This ubiquitous blueness, however, is no mere accident of nature, but the direct consequence of a fascinating physical phenomenon: Rayleigh Scattering.
Defining Rayleigh Scattering
Rayleigh Scattering is the elastic scattering of electromagnetic radiation—light, in simpler terms—by particles of a wavelength much smaller than that of the radiation itself. Imagine tiny marbles (atmospheric particles) deflecting larger billiard balls (light waves). This interaction, occurring constantly within our atmosphere, is the key to understanding why the sky appears blue.
Unlike inelastic scattering processes where energy exchange occurs, elastic scattering implies conservation of energy. The photon's energy remains unaltered; only its direction of propagation changes.
The Scope of Our Exploration
This article embarks on a comprehensive exploration of Rayleigh Scattering. We will delve into the historical context of its discovery, tracing the intellectual lineage back to Lord Rayleigh's seminal work.
Beyond history, we will unpack the underlying scientific principles that govern this phenomenon, with a keen focus on the crucial relationship between wavelength and scattering intensity.
Finally, we will explore the myriad practical implications of Rayleigh Scattering, from its role in atmospheric optics to its relevance in other scientific and engineering disciplines.
Our goal is to provide a clear and accessible understanding of this fundamental process, empowering readers to appreciate the science behind the simple beauty of a blue sky.
Why This Matters
Understanding Rayleigh Scattering is not merely an academic exercise. It's a window into the intricate workings of our atmosphere and the very nature of light itself. It highlights how microscopic interactions, happening continuously around us, shape our macroscopic reality. It further highlights the value of curiosity-driven scientific investigation, for it shows how an explanation for a seemingly simple observation can have far-reaching consequences for the progress of all knowledge.
Historical Roots: Lord Rayleigh and Early Discoveries
The story of Rayleigh Scattering is inextricably linked to the scientific inquiries of the 19th century, a period marked by groundbreaking advancements in optics and electromagnetism. At the heart of this narrative stands John William Strutt, the 3rd Baron Rayleigh, whose theoretical insights provided the first comprehensive explanation for the sky's captivating blue hue. While Rayleigh’s name is most prominently associated with this phenomenon, the broader context of scientific progress, including the foundational work of Albert Einstein in electromagnetism, is crucial for a complete understanding.
Lord Rayleigh's Seminal Contribution
Lord Rayleigh's meticulous investigations into the scattering of light represent a cornerstone in the history of physics.
His theoretical framework, developed in the late 19th century, elegantly connected the observed blue color of the sky to the interaction of sunlight with tiny particles in the atmosphere.
Prior to Rayleigh's work, the prevailing explanations for the sky's color were largely speculative and lacked a rigorous scientific basis.
Rayleigh's genius lay in his ability to formulate a mathematical model that precisely described the relationship between the wavelength of light and the intensity of scattering.
The Wavelength-Intensity Nexus
The crux of Rayleigh's theory resides in the inverse relationship between wavelength and scattering intensity. He demonstrated that the intensity of scattered light is inversely proportional to the fourth power of its wavelength (1/λ4).
This means that shorter wavelengths, corresponding to blue and violet light, are scattered much more effectively than longer wavelengths, like red and orange.
As sunlight enters the Earth's atmosphere, these shorter wavelengths are preferentially scattered in all directions by the numerous air molecules.
This widespread scattering of blue light is what gives the sky its characteristic color.
At sunrise and sunset, when sunlight travels through a greater distance within the atmosphere, the blue light is scattered away, leaving the longer wavelengths of red and orange to dominate, hence the vibrant colors we observe at these times of day.
Einstein's Electromagnetism: Laying the Groundwork
While Lord Rayleigh directly addressed the scattering phenomenon, the broader understanding of light as an electromagnetic wave, significantly advanced by Albert Einstein's work, provided the crucial foundation upon which Rayleigh's theory could flourish.
Einstein's contributions to electromagnetism, particularly his work on the photoelectric effect and his development of special relativity, profoundly impacted the understanding of light's nature.
These advancements in electromagnetic theory provided a more comprehensive framework for understanding the behavior of light as it interacts with matter.
By establishing the particle-wave duality of light, Einstein's work further solidified the theoretical underpinnings necessary to fully grasp the intricacies of Rayleigh Scattering.
Therefore, while Rayleigh focused specifically on the scattering phenomenon, Einstein's broader contributions to electromagnetism were also critical for understanding the underlying physics.
In conclusion, the explanation of why the sky is blue is a scientific narrative that highlights the power of theoretical physics, with Lord Rayleigh's insights and Einstein's contributions to electromagnetic theory standing as testaments to human intellect's ability to illuminate some of the most basic natural phenomena.
The Science Behind the Blue: Wavelength and Scattering Intensity
Understanding why the sky appears blue necessitates delving into the fundamental properties of light and its interaction with matter. At the heart of this explanation lies the concept of wavelength, a critical parameter that dictates how light interacts with the particles present in our atmosphere. The interplay between wavelength and scattering intensity, as elucidated by Rayleigh's theory, is the key to unraveling this captivating phenomenon.
Wavelength: The Defining Characteristic of Light
Light, in its wave-like nature, is characterized by its wavelength, which is the distance between successive crests or troughs of the wave.
Wavelength is typically measured in nanometers (nm), with visible light spanning a range from approximately 400 nm (violet) to 700 nm (red).
Different wavelengths correspond to different colors in the visible spectrum. For instance, blue light has a shorter wavelength compared to red light.
This seemingly simple difference in wavelength has profound consequences for how light interacts with the tiny particles that constitute our atmosphere.
The Inverse Relationship: Unveiling the Scattering Mechanism
Rayleigh's groundbreaking contribution was to establish the mathematical relationship between wavelength and scattering intensity.
His theory demonstrates that the intensity of scattered light is inversely proportional to the fourth power of the wavelength (1/λ4).
This relationship is paramount in understanding why blue light dominates the sky's appearance.
The significance of this inverse relationship is that shorter wavelengths are scattered much more efficiently than longer wavelengths.
Preferential Scattering of Blue Light
Because blue light has a shorter wavelength than red light, it is scattered far more intensely by the air molecules in the atmosphere.
As sunlight enters the atmosphere, the shorter wavelengths of blue and violet light are preferentially scattered in all directions.
This widespread scattering of blue light is what imparts the sky its characteristic blue hue.
The fact that violet light has an even shorter wavelength than blue light, one might wonder, why is the sky not violet? Because sunlight has less violet than blue light, and because the atmosphere absorbs some violet light.
Red Sunsets: A Consequence of Wavelength-Dependent Scattering
At sunrise and sunset, when sunlight traverses a longer path through the atmosphere, the blue light is scattered away, leaving the longer wavelengths of red and orange to dominate.
These longer wavelengths are less susceptible to scattering and can therefore penetrate the atmosphere more effectively over greater distances.
This phenomenon explains the captivating red and orange hues that paint the sky during these times of day.
The interplay between wavelength and scattering intensity is a fundamental principle that elucidates not only the blue color of the sky but also a range of other atmospheric optical phenomena, demonstrating the elegance and power of scientific inquiry.
Understanding Elastic Scattering: Energy Conservation in Action
Having established the relationship between wavelength and scattering intensity, it is crucial to examine the nature of the scattering process itself. Rayleigh scattering is a prime example of elastic scattering, a fundamental interaction in physics governed by the principles of energy conservation. Understanding the implications of elastic scattering provides deeper insight into the phenomenon responsible for the sky's blue hue.
What is Elastic Scattering?
Elastic scattering, in its simplest form, is a collision in which the total kinetic energy of the system remains constant. In the context of light and particles, this means that when a photon interacts with a particle and is scattered, the photon's energy is conserved.
No energy is transferred to the scattering particle, and no internal excitation or ionization of the particle occurs. This energy conservation is a defining characteristic of elastic scattering events.
Energy Conservation in Rayleigh Scattering
In Rayleigh scattering, a photon interacts with an air molecule (typically nitrogen or oxygen) and is deflected from its original path. Crucially, the energy of the photon remains virtually unchanged after the interaction.
The photon's wavelength, and therefore its frequency and energy, are the same before and after scattering. The air molecule experiences a negligible change in its kinetic energy.
This contrasts sharply with other types of scattering where energy transfer does occur. This is what makes elastic scattering so uniquely useful in applications like remote sensing and atmospheric studies.
A Brief Note on Inelastic Scattering
To fully appreciate elastic scattering, it's helpful to briefly consider its counterpart: inelastic scattering.
In inelastic scattering, the total kinetic energy of the system is not conserved. This means that during the scattering event, some of the photon's energy is transferred to the scattering particle.
This can lead to excitation of the particle to a higher energy level or even ionization. Examples of inelastic scattering include Raman scattering and Compton scattering, which involve changes in the wavelength of the scattered light.
The Significance of Elasticity in Atmospheric Optics
The elastic nature of Rayleigh scattering is essential for the sky's appearance. Because the scattered light retains its original energy (and therefore its wavelength), the blue light scattered by air molecules remains blue.
If Rayleigh scattering were inelastic, the scattered light would undergo a change in wavelength. Then the sky could appear in a different color, and the sunset color gradient we observe might not exist.
Elastic scattering allows us to study the atmosphere without significantly altering the energy of the light being scattered. This makes it a valuable tool for atmospheric research and remote sensing applications.
The Atmosphere's Role: A Scattering Medium
Having established the importance of energy conservation in Rayleigh scattering, it is time to explore the arena where this phenomenon plays out: the Earth's atmosphere. The atmosphere isn't merely a backdrop; it's an active participant in the creation of the blue sky. Its unique properties determine the efficiency and characteristics of Rayleigh scattering.
Understanding the atmosphere's role is essential to fully appreciating why the sky appears as it does.
The Atmosphere as the Primary Scattering Environment
The atmosphere provides the necessary medium for Rayleigh scattering to occur on a large scale. It is composed primarily of nitrogen (N2) and oxygen (O2) molecules.
These molecules, being much smaller than the wavelengths of visible light, act as the scattering particles. Without the atmosphere, sunlight would travel unimpeded through space, and the sky would appear black, similar to what astronauts observe on the Moon.
The density and composition of the atmosphere are crucial determinants of the intensity and color distribution of the scattered light we perceive.
Impact of Atmospheric Composition on Scattering
The specific mix of gases within the atmosphere affects which wavelengths are most effectively scattered.
While nitrogen and oxygen are the dominant players, other trace gases and aerosols can also contribute to scattering, although usually to a lesser extent.
The presence of these particles can alter the color of the sky, especially near the horizon, where the path length of light through the atmosphere is greatest. Consider how air pollution contributes to haziness and color changes in the sky.
Different altitudes also have differing compositions, affecting the overall scattering profile.
The Influence of Atmospheric Density
Atmospheric density plays a critical role in the efficiency of Rayleigh scattering.
Density generally decreases with increasing altitude, meaning that there are fewer air molecules available to scatter light at higher altitudes.
This explains why the sky appears darker as one ascends to higher altitudes. The reduced density leads to less scattering, and subsequently less light reaching the observer's eye.
Changes in atmospheric density, caused by temperature variations or weather patterns, can also influence the intensity of scattered light.
Density Fluctuations and Scattering Intensity
Even at a given altitude, there are localized fluctuations in air density due to random molecular motion.
These density fluctuations contribute to the scattering of light, even in a perfectly uniform gas. This explains why even "empty" space is not truly empty with respect to light scattering.
These minute variations in density are sufficient to initiate the cascade of scattering events that ultimately give rise to the sky's color.
Understanding these dynamic interactions is key to predicting light behavior in the atmosphere.
The interplay between atmospheric composition and density is a dynamic process. These atmospheric qualities are critical to the propagation and scattering of light. This in turn paints the backdrop against which we observe the daily phenomenon of a blue sky.
Without this unique atmospheric environment, the familiar blue hue would be replaced by the stark blackness of space.
Intensity of Scattered Light: Factors at Play
Building upon the understanding of atmospheric scattering, it is crucial to explore what determines the actual brightness or intensity of the scattered light that reaches our eyes. The vibrant blue we perceive isn't just a consequence of preferential short-wavelength scattering; it's also governed by a complex interplay of factors that modulate the overall light intensity.
These factors—wavelength, scattering angle, and particle concentration—each wield a significant influence on the resulting visual spectacle. A deeper dive into each aspect reveals the intricate mechanisms that shape our perception of the sky's color.
Wavelength Dependence and Perceived Brightness
As previously established, shorter wavelengths are scattered more effectively by Rayleigh scattering. However, the intensity of the scattered light is not merely a binary function of wavelength. It's a continuous, inversely proportional relationship.
This means that while blue light is scattered more than red light, the difference in intensity is a gradated spectrum rather than an absolute threshold.
Consequently, the specific shade of blue we see is influenced by the precise mix of wavelengths present in the incident sunlight and the atmosphere's scattering efficiency across that range. The scattered light's intensity is proportionally inverse to the fourth power of its wavelength.
Scattering Angle and Distribution of Light
The angle at which light is scattered relative to its original direction is another crucial determinant of intensity. Rayleigh scattering is not isotropic. Light is scattered more intensely in the forward and backward directions than at right angles.
This angular dependence explains why the sky near the sun appears brighter than the sky further away. The observer's position relative to the sun affects their perceived scattering intensity.
Furthermore, the polarization of light changes with the scattering angle. The intensity of light is not uniform across all angles. These variations influence the color gradients and perceived brightness across the sky.
Particle Concentration: Amplifying the Effect
The concentration of scattering particles, i.e., air molecules, directly impacts the overall intensity of scattered light. A higher concentration means more scattering events, leading to a brighter sky.
This is why the sky is generally brighter at lower altitudes, where the atmospheric density and thus particle concentration are higher. Conversely, at higher altitudes, the lower density results in a fainter, darker sky.
However, extremely high concentrations can lead to multiple scattering events, where light is scattered multiple times before reaching the observer. This can effectively "blur" the color and reduce the overall intensity of the direct light.
Putting it All Together: A Dynamic System
The intensity of scattered light is not determined by any single factor in isolation but rather by their combined effect. Wavelength determines the potential for scattering. The scattering angle affects the distribution of that scattered light. The atmospheric concentration serves as a multiplier on the overall intensity.
Understanding these intertwined relationships allows us to appreciate the complexity of Rayleigh scattering and how subtle changes in atmospheric conditions can dramatically alter the appearance of the sky. The balance of these elements creates the daily panorama we often take for granted.
The Electromagnetic Spectrum: Contextualizing Rayleigh Scattering
Having explored the individual factors influencing scattered light, it is essential to situate Rayleigh scattering within the broader context of the electromagnetic spectrum. Understanding the spectrum allows us to appreciate which types of radiation are most susceptible to this phenomenon and why the visible light portion is so crucial to our perception of the world around us. It’s not just about blue skies; it’s about understanding the interaction of electromagnetic energy with matter.
This section aims to paint that broader picture, highlighting the portion of the electromagnetic spectrum most relevant to Rayleigh scattering and illuminating why visible light plays such a prominent role.
Understanding the Electromagnetic Spectrum
The electromagnetic spectrum encompasses the entire range of electromagnetic radiation, from low-frequency radio waves to high-frequency gamma rays. Each type of radiation is characterized by its wavelength and frequency, which are inversely proportional.
The spectrum is a continuum, with no strict boundaries between different regions, but it's typically divided into categories such as radio waves, microwaves, infrared radiation, visible light, ultraviolet radiation, X-rays, and gamma rays. Each region interacts with matter in distinct ways.
Rayleigh scattering is most pronounced when the scattering particles are much smaller than the wavelength of the incident radiation. Therefore, this phenomenon has significantly variable effects across the electromagnetic spectrum.
Rayleigh Scattering and Visible Light
While Rayleigh scattering can theoretically occur with any electromagnetic radiation, it is most noticeable and consequential within the visible light portion of the spectrum.
The wavelengths of visible light—ranging from approximately 400 nanometers (violet) to 700 nanometers (red)—are on a scale where they interact strongly with the air molecules present in our atmosphere.
This interaction is precisely what gives rise to the blue color of the sky: shorter wavelengths (blue and violet) are scattered far more efficiently than longer wavelengths (red and orange). Note: our eyes are also less sensitive to violet light.
Visible Light: A Closer Look at the Colors Affected
Within the visible light spectrum, different colors are affected by Rayleigh scattering to varying degrees. Blue and violet light are scattered approximately ten times more efficiently than red light. However, because violet is absorbed more readily than blue, and our eyes are also less sensitive to violet, the sky appears blue.
The scattering of green and yellow light also occurs, albeit to a lesser extent. This wavelength-dependent scattering is why sunsets and sunrises often appear reddish: the blue light has been scattered away by the time the sunlight reaches our eyes, leaving the longer, redder wavelengths to dominate.
Understanding the interplay between wavelength and scattering efficiency within the visible light spectrum is essential to grasp how Rayleigh scattering shapes our visual experience of the sky and atmosphere.
Beyond Visible Light: Rayleigh Scattering at Other Wavelengths
While Rayleigh scattering is most prominent within the visible light spectrum, it also occurs at other wavelengths, albeit often with less noticeable effects. For example, ultraviolet (UV) radiation is strongly scattered by atmospheric particles.
The scattering of UV radiation is crucial in protecting life on Earth. The ozone layer plays a primary role in absorbing harmful UV rays.
At longer wavelengths, such as infrared radiation, Rayleigh scattering is much less efficient because the wavelengths are significantly larger than the scattering particles (air molecules). Other types of scattering, such as Mie scattering, become more dominant at these wavelengths.
By placing Rayleigh scattering within the context of the entire electromagnetic spectrum, we gain a fuller appreciation for its specific role in shaping our perception of the world and its broader significance in atmospheric optics and radiative transfer.
Scattering Cross Section: Quantifying the Likelihood of Light's Deflection
Having dissected the various factors influencing the intensity of scattered light, we now turn to a more precise measure of the scattering phenomenon: the scattering cross section. This concept allows us to quantify the probability of a photon being scattered by a particle, providing a powerful tool for understanding and predicting the behavior of light in various media. It's not merely about observing scattering; it's about assigning a concrete value to its likelihood.
This section will explore the concept of the scattering cross section, its physical interpretation, its units of measurement, and its relation to the probability of collision between photons and scattering particles.
Defining the Scattering Cross Section
The scattering cross section, denoted by σ (sigma), can be best understood as the effective area that a scattering particle presents to an incident photon. If the photon passes through this area, scattering occurs.
It’s a theoretical construct, not the physical size of the particle, but rather an indicator of the likelihood of interaction. The larger the scattering cross section, the higher the probability that the photon will be scattered.
The Scattering Cross Section as a Probability Measure
The primary function of the scattering cross section is to quantify the probability that a photon will be scattered by a given particle. A high scattering cross section implies a high probability of scattering, while a low value indicates that scattering is less likely to occur.
It is not a certainty, but rather a statistical measure, averaging the interactions of many photons. It's a bit like saying, "If I throw a dart at this target, what are my chances of hitting the bullseye?"
Units of Measurement and Physical Interpretation
The scattering cross section is measured in units of area, typically expressed in square meters (m2) or square centimeters (cm2).
This seemingly simple unit offers a powerful insight: the scattering cross section represents the effective area that the scattering particle presents to the incoming photon.
Imagine shining a beam of light onto a collection of these particles; the scattering cross section essentially describes the fraction of the beam that will be diverted due to scattering.
Relation to Collision Probability
The scattering cross section can be directly related to the probability of a "collision" between a photon and a scattering particle. Although photons are not solid objects, their interaction with particles can be mathematically described in a way that resembles a collision.
If we know the density of scattering particles in a given medium and the scattering cross section of each particle, we can calculate the mean free path of a photon – the average distance a photon travels before being scattered.
A higher particle density or a larger scattering cross section will result in a shorter mean free path, indicating more frequent scattering events. This concept is crucial for understanding radiative transfer in atmospheres and other scattering media.
Video: Why is the Sky Blue? Rayleigh Scattering Explained
FAQs: Why is the Sky Blue? Rayleigh Scattering Explained
Is the sky *always* blue?
No. The sky is blue because of Rayleigh scattering, which scatters shorter wavelengths of light like blue and violet more effectively. At sunrise and sunset, the light travels through more atmosphere, scattering away much of the blue. This leaves longer wavelengths like red and orange to dominate, creating those colors.
If violet light is scattered more, why isn't the sky violet?
While violet light is scattered slightly more than blue light by Rayleigh scattering, the sun emits less violet light than blue light. Additionally, our eyes are more sensitive to blue. This combination means that we perceive the sky as blue rather than violet, even though Rayleigh scattering is the root cause.
Does Rayleigh scattering explain why the ocean is blue too?
Not entirely. The ocean's blue color is primarily due to the absorption and scattering of light by water molecules. While Rayleigh scattering does play a role in water, selective absorption, particularly of red and orange light, is the dominant process in making the ocean appear blue. So, while Rayleigh scattering contributes, it's not the main reason.
Is Rayleigh scattering only related to the color of the sky?
No. Rayleigh scattering affects light propagation in various situations involving particles much smaller than the wavelength of the light. While it’s most commonly associated with explaining why is the sky blue due to the interaction of sunlight with air molecules, it’s a general principle of physics applicable in many contexts.
So, next time you're gazing up at that beautiful blue expanse, remember Rayleigh scattering! Why is the sky blue? Rayleigh scattering is the reason! It's pretty cool to think that something as simple as the way light bounces around can explain one of nature's most captivating sights. Hopefully, this cleared things up, and you can now impress your friends with your newfound atmospheric knowledge!