Selenium Lewis Structure: Demystifying the Dot Diagram!
Understanding the seleniumlewis dot structure is fundamental to grasping chemical bonding principles. Valence electrons, a crucial concept taught in general chemistry, determine how atoms interact. The octet rule, which dictates that atoms seek to have eight valence electrons, directly influences the construction of a seleniumlewis dot structure. Furthermore, resources like Khan Academy offer excellent explanations for visualizing and understanding these diagrams, ultimately simplifying the process of drawing an accurate seleniumlewis dot structure.
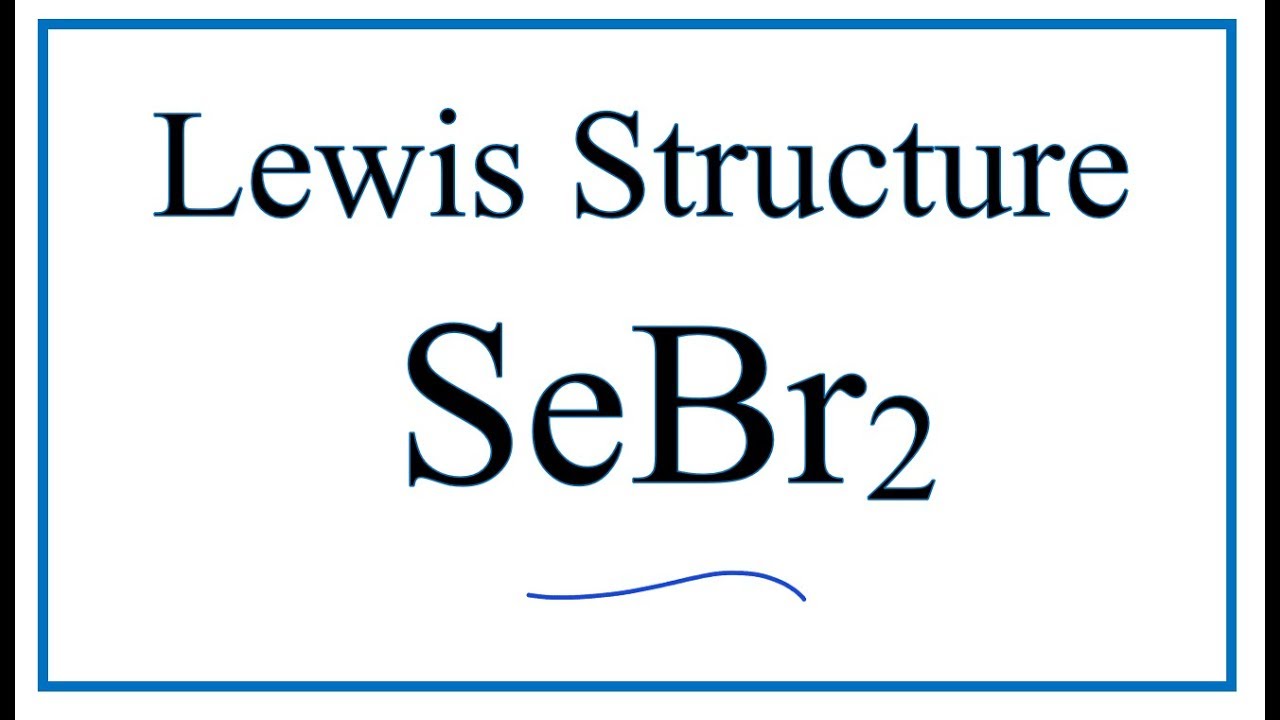
Image taken from the YouTube channel Wayne Breslyn (Dr. B.) , from the video titled How to Draw the Lewis Dot Structure for SeBr2: Selenium dibromide .
Selenium (Se), a fascinating element nestled in the periodic table, often flies under the radar. Yet, its unique properties make it indispensable in various fields, from electronics to nutritional supplements.
Understanding how selenium interacts with other elements to form compounds is crucial. This is where Lewis Dot Structures, also known as electron dot diagrams, come into play.
These diagrams offer a simple yet powerful visual representation of an atom's valence electrons. They show how these electrons are arranged and shared during chemical bonding.
By understanding these structures, we can gain insights into a molecule's stability, reactivity, and overall behavior.
This article serves as a comprehensive guide. It will walk you through the process of drawing and interpreting Lewis Dot Structures for Selenium.
Our aim is to equip you with the knowledge and skills necessary to visualize selenium's bonding behavior.
Selenium: An Overview of the Element
Selenium is a nonmetal belonging to Group 16 (also known as the chalcogens) of the periodic table. Its atomic number is 34.
It exhibits interesting properties that lie between those of sulfur and tellurium, its neighboring elements in the same group.
Selenium exists in several allotropic forms, including a red amorphous form and a gray, metallic form.
Its conductivity changes with light exposure, making it valuable in photocells and solar cells.
Beyond electronics, selenium plays a vital role in biology. It is an essential trace element for humans and animals, incorporated into selenoproteins that have antioxidant and other important functions.
The Elegance of Lewis Dot Structures
Lewis Dot Structures, named after Gilbert N. Lewis, are a fundamental tool in chemistry. They provide a simplified way to visualize the arrangement of valence electrons in atoms and molecules.
Valence electrons, those in the outermost shell, are the ones primarily involved in chemical bonding.
In a Lewis Dot Structure, the element's symbol represents the nucleus and core electrons. Dots surrounding the symbol represent the valence electrons.
These dots are arranged in pairs around the element's symbol, following the octet rule (which we will explore in more detail later).
Lewis Dot Structures are indispensable for predicting how atoms will bond to form molecules. They help us understand the distribution of electrons in a molecule and predict its shape and properties.
Your Guide to Mastering Selenium Lewis Dot Structures
This article is designed to be your go-to resource for understanding Selenium Lewis Dot Structures.
We will guide you through each step, from identifying selenium's valence electrons to constructing accurate and informative diagrams.
We'll cover:
- The fundamental principles behind Lewis Dot Structures.
- A step-by-step guide to drawing Selenium Lewis Dot Structures.
- Examples of various selenium compounds.
- More complex scenarios.
By the end of this guide, you will have a solid understanding of how to draw and interpret Lewis Dot Structures for Selenium, empowering you to visualize and understand its role in chemical bonding.
The Elegance of Lewis Dot Structures allows us to visualize how atoms share electrons and form bonds, providing a pathway to understanding the behavior of molecules. To fully appreciate selenium's role in this elegant dance, we need to first establish a firm understanding of its fundamental properties and how they relate to chemical bonding.
Fundamentals: Selenium's Place and Properties
Before we can dive into drawing Lewis Dot Structures for Selenium (Se), it's essential to grasp the basic principles governing its behavior. This involves understanding its position on the periodic table, the significance of its valence electrons, and the driving force behind chemical bonding: the Octet Rule. These fundamentals will provide the necessary context for understanding how selenium interacts with other atoms.
Selenium in the Periodic Table
Selenium resides in a specific location on the periodic table, and this placement dictates much of its chemical behavior.
Locating Selenium
Take a look at the periodic table. Find Selenium (Se). You'll notice it's situated in the Group 16 (also known as the chalcogens) and in the 4th period.
Selenium as a Chalcogen
Being a member of the chalcogen family means that Selenium shares certain characteristics with other elements in this group, such as oxygen and sulfur. This family is known for their tendency to form compounds with a wide range of elements.
The Significance of Valence Electrons
Most importantly, its group number reveals the number of valence electrons it possesses. These are the electrons in the outermost shell of an atom, and they are crucial for chemical bonding.
Valence Electrons: The Key to Bonding
Valence electrons dictate how an atom interacts with other atoms to form molecules. Understanding their role and how to determine their number is key to understanding Lewis Dot Structures.
Defining Valence Electrons
Valence electrons are the electrons located in the outermost electron shell or energy level of an atom. These electrons are responsible for participating in chemical bonds. They determine an atom’s chemical properties and reactivity.
Determining Selenium's Valence Electrons
As a member of Group 16, Selenium has six valence electrons. This means it needs two more electrons to achieve a stable electron configuration, similar to that of a noble gas. This electron deficiency explains Selenium's tendency to form bonds with other atoms.
The Octet Rule: Striving for Stability
The Octet Rule is a guiding principle in understanding how atoms form chemical bonds. It provides a framework for predicting how atoms will interact to achieve stability.
Introducing the Octet Rule
The Octet Rule states that atoms tend to gain, lose, or share electrons in order to achieve a full outer electron shell containing eight electrons. This stable configuration is isoelectronic with the noble gases, which are known for their inertness.
Influence on Bond Formation
The Octet Rule explains why atoms form chemical bonds. By sharing electrons, atoms can achieve a more stable electron configuration and lower their overall energy. This drive towards stability governs the formation of molecules and compounds.
Selenium's Quest for an Octet
Given that Selenium has six valence electrons, it typically forms two covalent bonds to complete its octet. However, it can also exhibit more complex bonding behavior, sometimes exceeding the octet, as we'll explore later. The drive to achieve this stable octet is the primary reason why selenium participates in chemical bonding.
Step-by-Step Guide: Constructing the Selenium Lewis Dot Structure
Having established the fundamental properties of selenium, we're now ready to translate this knowledge into a visual representation of its bonding behavior. The Lewis Dot Structure serves as our roadmap, guiding us through the arrangement of atoms and electrons in a molecule containing selenium. Let's embark on a step-by-step journey to master the art of constructing these insightful diagrams.
Identifying the Central Atom
The first step in drawing a Lewis Dot Structure is pinpointing the central atom.
This atom serves as the anchor around which all other atoms are arranged.
Rules for Central Atom Identification
Generally, the least electronegative atom in the molecule takes center stage.
Electronegativity measures an atom's attraction for electrons in a chemical bond.
Selenium is less electronegative than oxygen or fluorine but more electronegative than hydrogen, for instance.
Another useful guideline is that the central atom is often the one present in the smallest quantity in the molecule.
Selenium as the Central Atom
In simple compounds like selenium hydride (SeH2), selenium unequivocally assumes the role of the central atom.
This is because hydrogen atoms invariably occupy terminal positions, forming only one bond.
Selenium, with its capacity to form multiple bonds due to its two unpaired electrons, naturally becomes the focal point.
Counting Total Valence Electrons
Once the central atom is identified, the next crucial step is to tally up the total number of valence electrons present in the molecule.
This number represents the entire pool of electrons available for bonding and forming lone pairs.
Calculating Valence Electrons
To calculate the total valence electrons, refer to the periodic table.
Each element's group number corresponds to the number of valence electrons it contributes.
Sum the valence electrons of each atom in the molecule to obtain the total.
Examples of Valence Electron Calculation
Let's illustrate with two examples: SeH2 and SeO2.
-
SeH2: Selenium (Group 16) contributes 6 valence electrons, and each hydrogen (Group 1) contributes 1. Therefore, the total valence electrons are 6 + (2
**1) = 8.
-
SeO2: Selenium (Group 16) contributes 6 valence electrons, and each oxygen (Group 16) contributes 6. Thus, the total valence electrons are 6 + (2** 6) = 18.
This meticulous counting of valence electrons sets the stage for accurately distributing them in the subsequent steps.
Forming Single Bonds: The First Connections
With the total valence electron count in hand, we now begin constructing the framework of the Lewis structure by forming single bonds between the central atom and the surrounding atoms.
Drawing Single Bonds
Represent each single bond as a straight line connecting the atoms.
Each single bond represents the sharing of two electrons between the atoms involved.
Understanding Bonding Pairs
These shared electron pairs are known as bonding pairs.
They hold the atoms together, forming the foundation of the molecule.
For example, in SeH2, we would draw two single bonds: one connecting selenium to each hydrogen atom (H-Se-H).
This accounts for 4 of the 8 valence electrons calculated earlier, leaving 4 electrons left for distribution.
Distributing Lone Pairs: Satisfying the Octet Rule
Having established the basic connectivity through single bonds, our next task is to distribute the remaining valence electrons as lone pairs.
What are Lone Pairs?
Lone pairs are non-bonding pairs of electrons that reside on individual atoms.
They contribute to the electron density around the atom and influence its reactivity.
Placing Lone Pairs
The objective is to distribute these lone pairs in a manner that satisfies the octet rule for each atom (except hydrogen, which aims for a duet).
Start by placing lone pairs on the surrounding atoms until they achieve an octet (8 electrons).
Then, place any remaining lone pairs on the central atom.
In SeH2, after forming the two single bonds, we have 4 electrons remaining. These will be distributed as two lone pairs on the selenium atom.
Showing Lone Pair Placement
Each lone pair is represented by two dots placed next to the atom.
This visual representation clarifies the electron distribution around each atom.
Verifying the Octet Rule: A Final Check
The final step in constructing a Lewis Dot Structure is to meticulously verify that each atom (with the exception of hydrogen) has achieved an octet of electrons.
The Octet Rule in Practice
This means that each atom should be surrounded by eight electrons, either through bonding pairs or lone pairs.
Carefully count the electrons around each atom to ensure the octet rule is satisfied.
Hydrogen, being a smaller atom, is stable with just two electrons (a duet).
Exceptions to the Octet Rule
While the octet rule is a powerful guideline, there are exceptions, particularly with elements in the third period and beyond, such as selenium.
These elements can sometimes accommodate more than eight electrons in their valence shell, forming what are known as expanded octets.
We will address expanded octets in selenium compounds in a later section.
However, for many simple selenium compounds, the octet rule provides an accurate framework for understanding their Lewis Dot Structures.
Having mastered the fundamentals of constructing Selenium Lewis Dot Structures, and understanding the step-by-step process, it's time to broaden our horizons. We'll now explore more complex scenarios, examining diverse selenium compounds, delving into the concept of resonance where applicable, and confronting instances where the seemingly immutable Octet Rule bends, or even breaks, under the influence of selenium's unique electronic configuration.
Advanced Examples and Considerations
Exploring Selenium Compounds: Diverse Structures
Selenium, like other elements in its group, forms a variety of compounds with diverse structures and bonding characteristics. Understanding these compounds requires applying the principles we've already established and appreciating the nuances that arise in each specific case. Let's explore a few representative examples.
Selenium Hydride (SeH2)
Selenium hydride (SeH2) is a simple molecule where selenium acts as the central atom, bonded to two hydrogen atoms. As we’ve discussed, hydrogen only forms single bonds.
The Lewis structure reflects this, with two single bonds between Se and H, and two lone pairs on the selenium atom to complete its octet. The bent molecular geometry arises from the repulsion of these electron pairs.
Selenium Dioxide (SeO2)
Selenium dioxide (SeO2) presents a slightly more complex scenario. Selenium is bonded to two oxygen atoms. Oxygen, like selenium, typically needs two bonds to achieve an octet.
The most common representation involves double bonds between selenium and each oxygen atom. This satisfies the octet rule for all atoms.
However, resonance structures can also be drawn with one single and one triple bond. This is a valid representation, although less favored due to formal charge considerations.
Selenium Dichloride (SeCl2)
Selenium dichloride (SeCl2) is analogous to SeH2 in structure. Selenium is the central atom, bonded to two chlorine atoms.
Chlorine, like hydrogen, typically forms only single bonds. Each chlorine atom is connected to selenium through a single bond.
Selenium, in turn, has two lone pairs to satisfy its octet. The molecule adopts a bent shape due to the lone pair repulsion.
Resonance Structures: Multiple Valid Representations
The concept of resonance becomes important when a single Lewis structure cannot accurately represent the electron distribution in a molecule. This is particularly relevant when dealing with molecules containing multiple bonds and lone pairs.
For some Selenium compounds, multiple valid Lewis structures can be drawn.
These structures differ only in the arrangement of electrons, not the arrangement of atoms. The actual molecule is a resonance hybrid, a weighted average of all contributing resonance structures.
Consider SeO2, as mentioned previously. The double-bonded structure is a major contributor, but resonance structures with single and triple bonds also contribute, albeit to a lesser extent.
The true structure is somewhere in between, with the electron density delocalized across the molecule.
Deviations from the Octet Rule: Expanded Octets
While the Octet Rule is a powerful guideline, it's not universally applicable. Certain elements, including selenium, can sometimes accommodate more than eight electrons in their valence shell.
This phenomenon is known as octet expansion or forming an expanded octet. It occurs primarily in elements from the third period and beyond due to the availability of vacant d-orbitals.
Selenium, being in the fourth period, can utilize its d-orbitals to accommodate extra electrons.
Examples of Expanded Octets
Selenium hexafluoride (SeF6) is a prime example of a compound where selenium violates the Octet Rule. In SeF6, selenium is bonded to six fluorine atoms.
Each bond contributes two electrons to selenium's valence shell, resulting in a total of twelve electrons around the selenium atom. This is possible because selenium can utilize its d-orbitals to form these additional bonds.
Selenium oxyfluoride (SeOF2) is another example. Here, selenium is double bonded to oxygen and single bonded to two fluorine atoms. While this seems to satisfy the octet rule at first glance, more complex structures can be drawn and are seen under certain conditions. This results in selenium exceeding its octet.
Understanding when and how the Octet Rule can be broken is crucial for accurately depicting the bonding in various selenium compounds. While it requires a deeper understanding of electronic structure and orbital theory, it expands our ability to describe the diverse world of chemical bonding.
Having explored diverse structures and considered various exceptions, our understanding of selenium's bonding behavior is becoming more refined. But the story doesn't end with just the arrangement of electrons; the nature of the bonds themselves – their polarity and the underlying electronegativity differences – play a crucial role in determining the properties of selenium compounds.
Electronegativity and Bond Polarity
The characteristics of chemical bonds are not uniform; they possess a spectrum of behaviors, influenced greatly by the electronegativity of the atoms involved. This section will dissect the concept of electronegativity, explore how it dictates the polarity of bonds involving selenium, and understand the cascading effects on molecular properties.
Understanding Electronegativity
Electronegativity is a fundamental property of an atom that describes its ability to attract electrons towards itself in a chemical bond. It is not a directly measurable quantity but is rather based on observations of bond strengths and dipole moments.
Several scales exist for quantifying electronegativity, with the Pauling scale being the most widely used. Elements with high electronegativity values, like fluorine, have a strong pull on electrons, while elements with low electronegativity values, like cesium, have a weaker pull.
The electronegativity difference between two bonded atoms is critical. It dictates the distribution of electron density within the bond.
Selenium's Electronegativity
Selenium, positioned in Group 16 (the chalcogens) of the periodic table, has a moderate electronegativity value. This intermediate value dictates the types of bonds it forms.
It is neither highly electron-attracting nor strongly electron-donating. On the Pauling scale, selenium’s electronegativity is around 2.55. This positions it between metals and highly electronegative nonmetals.
Bond Polarity and Dipole Moments
When selenium bonds with an element of significantly different electronegativity, the bond becomes polar. In a polar bond, the electrons are not shared equally, resulting in a partial positive charge (δ+) on the less electronegative atom and a partial negative charge (δ-) on the more electronegative atom.
For instance, in selenium dioxide (SeO2), oxygen is considerably more electronegative than selenium. This creates a significant dipole moment in each Se-O bond. The oxygen atoms bear a partial negative charge, and the selenium atom bears a partial positive charge.
This unequal charge distribution influences the overall polarity of the molecule.
The dipole moment is a measure of the polarity of a molecule. It is defined as the product of the magnitude of the partial charges and the distance between them.
A molecule with polar bonds may or may not have an overall dipole moment, depending on its geometry. If the bond dipoles cancel each other out due to symmetry, the molecule is nonpolar, despite having polar bonds.
Impact on Molecular Properties
Bond polarity dramatically affects the physical and chemical properties of selenium compounds.
Polar molecules tend to have higher melting and boiling points than nonpolar molecules of similar size because the intermolecular forces are stronger in polar compounds. Dipole-dipole interactions and hydrogen bonding (if hydrogen is bonded to a highly electronegative atom) contribute to these stronger forces.
Solubility is also profoundly affected. Polar molecules are more soluble in polar solvents (like water), while nonpolar molecules are more soluble in nonpolar solvents (like hexane). This "like dissolves like" principle is a cornerstone of understanding solubility.
Chemical reactivity is also influenced by bond polarity. Polar bonds are often more reactive than nonpolar bonds because the partial charges make the atoms more susceptible to attack by nucleophiles or electrophiles.
Examples of Bond Polarity in Selenium Compounds
Consider these examples:
-
Selenium Hydride (SeH2): Selenium is slightly more electronegative than hydrogen. Therefore, the Se-H bonds are slightly polar, with a small partial negative charge on selenium and a small partial positive charge on hydrogen. The bent geometry of SeH2 ensures that the bond dipoles do not cancel, resulting in a net dipole moment and a polar molecule.
-
Selenium Tetrachloride (SeCl4): Chlorine is significantly more electronegative than selenium. The Se-Cl bonds are quite polar. Selenium bears a partial positive charge, and each chlorine atom bears a partial negative charge. The see-saw shape of the molecule ensures that the bond dipoles do not completely cancel out, resulting in a net dipole moment and a polar molecule.
Understanding electronegativity and bond polarity provides a deeper insight into the behavior of selenium compounds. It goes beyond simply drawing Lewis structures to explaining why these compounds exhibit the properties they do. This knowledge is crucial for predicting and manipulating the behavior of selenium compounds in various chemical applications.
Video: Selenium Lewis Structure: Demystifying the Dot Diagram!
FAQs: Understanding Selenium Lewis Structures
Here are some frequently asked questions to help you better understand the selenium Lewis dot structure and its representation.
What does the selenium Lewis structure tell us?
The selenium Lewis structure visually represents how valence electrons are arranged around a selenium atom and within a molecule containing selenium. It shows which electrons are involved in bonding and which are lone pairs, providing insights into the molecule's stability and reactivity. It's essentially a map of electron distribution.
Why are lone pairs important in a selenium Lewis structure?
Lone pairs of electrons on selenium influence the molecule's shape due to electron repulsion. These lone pairs contribute to the electron domain geometry and ultimately affect the molecular geometry. Recognizing the number and arrangement of these lone pairs is crucial for predicting molecular properties.
How do you determine the number of valence electrons for selenium in a selenium Lewis dot structure?
Selenium (Se) belongs to Group 16 (also known as Group 6A) of the periodic table. Therefore, it has 6 valence electrons. These valence electrons are what we represent as dots around the selenium symbol in its Lewis structure.
What if selenium is part of a polyatomic ion; how does that affect the selenium lewis dot structure?
If selenium is part of a polyatomic ion (like selenite, SeO3^2-), you must account for the ion's charge. If it's a negative charge, add electrons to the total valence electron count, and if it's a positive charge, subtract electrons. This adjustment ensures the correct selenium lewis dot structure for the ion is drawn.