Unlock the Rainbow: Roy G. Biv Wavelengths Explained!
Understanding the visual spectrum requires exploring roy g biv wavelengths, the foundation of color perception. Isaac Newton's experiments with prisms provided the initial framework for understanding this phenomenon. The Electromagnetic Spectrum encompasses these visible wavelengths, defining their place among other forms of radiation. Detailed analysis using tools such as a spectroscope allows scientists to precisely measure the specific wavelengths associated with each color in rainbows.
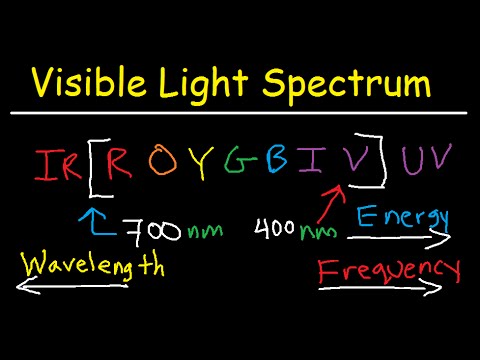
Image taken from the YouTube channel The Organic Chemistry Tutor , from the video titled Visible Light Spectrum Explained - Wavelength Range / Color Chart Diagram - Chemistry .
Unveiling the Secrets of Roy G. Biv: A Journey Through the Rainbow's Hues
What child hasn't tilted their head back, mesmerized by the arc of a rainbow painted across the sky? These fleeting spectacles, born from the interplay of light and water, hold a profound secret: they are a visible manifestation of the electromagnetic spectrum, a concept that governs much of the universe around us.
But how do we, as humans, begin to grasp the vastness of this phenomenon?
Enter Roy G. Biv, a simple yet powerful mnemonic device.
Roy G. Biv: Your Guide to the Visible Spectrum
Roy G. Biv isn't just a quirky name; it's a key. It's an acronym standing for Red, Orange, Yellow, Green, Blue, Indigo, and Violet – the colors that make up the visible spectrum. These are the colors we perceive when white light, like sunlight, is separated into its constituent parts, as happens when light passes through a prism or a raindrop.
This clever little memory tool helps us to order and recall the sequence of colors within the spectrum. Remembering Roy G. Biv is the first step to understanding that each color we see isn't just a shade, but a specific range of wavelengths of light.
Wavelengths and the Rainbow: Setting the Stage
Think of each color as a distinct signature, defined by its wavelength. Wavelength, measured in nanometers (nm), determines how much energy the light wave carries.
Red light, for instance, has a longer wavelength and lower energy than violet light, which has a shorter wavelength and higher energy.
Understanding these wavelengths is crucial.
This blog post aims to decode this fascinating relationship.
We will be exploring the specific wavelengths associated with each color in Roy G. Biv and how they connect to the broader context of the visible light spectrum. This journey will take us from the basics of the electromagnetic spectrum to the real-world applications of understanding these color wavelengths.
Prepare to see the rainbow, and the world, in a whole new light.
The Electromagnetic Spectrum and Visible Light: A Primer
With Roy G. Biv as our guide, we can begin to appreciate the intricacies of the rainbow. But to truly understand the nature of these colors, we must place them within a broader context: the electromagnetic spectrum.
This spectrum is the totality of all electromagnetic radiation, a vast and continuous range of energy that travels in waves.
Unveiling the Electromagnetic Spectrum
The electromagnetic spectrum encompasses a wide array of radiation types, each defined by its frequency and wavelength. At one end, we find radio waves, characterized by their long wavelengths and low frequencies.
These waves are used in broadcasting, communications, and navigation.
Moving along the spectrum, we encounter microwaves, which are shorter than radio waves and are used in cooking, telecommunications, and radar systems.
Next comes infrared radiation, which is associated with heat. Infrared radiation is used in thermal imaging, remote controls, and various industrial applications.
After infrared radiation, we arrive at the visible light spectrum, the only portion of the electromagnetic spectrum that is visible to the human eye.
Beyond visible light lies ultraviolet (UV) radiation, which has shorter wavelengths and higher energy than visible light. UV radiation can cause sunburns and skin cancer but is also used in sterilization and medical treatments.
Further along the spectrum are X-rays, which have even shorter wavelengths and higher energy than UV radiation. X-rays are used in medical imaging and security scanning.
Finally, at the other extreme of the spectrum, we find gamma rays, which have the shortest wavelengths and highest energy. Gamma rays are produced by nuclear reactions and are used in cancer treatment and industrial radiography.
The Visible Light Spectrum
The visible light spectrum, as the name suggests, is the only portion of the electromagnetic spectrum that is detectable by the human eye. It is the narrow band of wavelengths that our eyes are sensitive to, enabling us to perceive the world in color.
Understanding Wavelength: The Key to Color
Wavelength is a fundamental property of electromagnetic radiation, including visible light. It refers to the distance between two successive crests or troughs of a wave.
Wavelength is typically measured in nanometers (nm), where one nanometer is equal to one billionth of a meter (1 x 10-9 meters).
Each color in the visible spectrum corresponds to a specific range of wavelengths. For example, red light has a longer wavelength than violet light.
Wavelength and Energy: An Inverse Relationship
An important concept to grasp is the inverse relationship between wavelength and energy.
Shorter wavelengths correspond to higher energy, while longer wavelengths correspond to lower energy. This means that violet light, with its shorter wavelength, carries more energy than red light, with its longer wavelength.
This relationship is described by the equation E = hc/λ, where:
- E is energy,
- h is Planck's constant,
- c is the speed of light, and
- λ is wavelength.
This equation highlights that energy is inversely proportional to wavelength, reaffirming that shorter wavelengths have higher energy and vice versa.
Decoding Roy G. Biv: Exploring Each Color's Wavelength
Having established the broader context of the electromagnetic spectrum and the nature of visible light, we can now turn our attention to the individual colors that comprise the rainbow. Each color, neatly represented by the mnemonic Roy G. Biv, corresponds to a specific range of wavelengths, a unique energy level, and a myriad of real-world manifestations.
Red Light: The Longest Wavelength
Red light occupies the longest wavelength end of the visible spectrum.
Its wavelengths stretch approximately from 625 to 740 nanometers (nm).
This extended wavelength translates to a relatively lower energy level compared to other colors in the spectrum.
Sources of Red Light
We encounter red light in various forms, both natural and artificial.
Red LEDs are commonplace in electronic displays and indicator lights.
Red lasers find use in everything from barcode scanners to laser pointers.
The captivating hues of sunsets often feature a dominant red, caused by the scattering of shorter wavelengths as sunlight passes through the atmosphere.
Orange Light: A Blend of Warmth
Moving towards shorter wavelengths, we arrive at orange light.
Orange light spans approximately 590 to 625 nm.
It carries a slightly higher energy than red light.
Manifestations of Orange
Orange light graces our lives in multiple ways.
Like red light, orange light can be efficiently produced by LEDs.
Sunsets also present vibrant orange tones.
Nature provides us with orange light through the colors of various fruits, such as oranges themselves, pumpkins, and apricots.
Yellow Light: A Sign of Energy
The next color in our spectrum is yellow.
Yellow light has wavelengths ranging from approximately 565 to 590 nm.
This represents a further increase in energy compared to orange light.
Yellow in Our Environment
Yellow light abounds in our world.
LEDs, again, can be tuned to emit yellow light.
Sunlight itself contains a significant component of yellow light.
Lemons and other yellow fruits and flowers provide examples of yellow coloration through selective reflection and absorption of light.
Green Light: The Color of Life
Green light occupies the central portion of the visible spectrum.
Its wavelengths fall within the approximate range of 500 to 565 nm.
Green light's energy level is intermediate compared to the colors already discussed.
Where We Find Green
Green is ubiquitous in nature.
Green LEDs are widely used in electronic devices.
Plants owe their green color to chlorophyll, a pigment that absorbs red and blue light while reflecting green.
Certain gemstones, such as emeralds, display their characteristic green color due to the presence of trace elements within their crystal structure.
Blue Light: Approaching Higher Energy
As we continue towards shorter wavelengths, we encounter blue light.
Blue light encompasses the approximate wavelength range of 450 to 500 nm.
Its energy level is notably higher than that of green light.
Blue in Our World
Blue light surrounds us constantly.
Blue LEDs are a common component of modern lighting and displays.
The sky appears blue due to Rayleigh scattering, a phenomenon where shorter wavelengths of light are scattered more effectively by atmospheric particles.
Blueberries and other blue-colored foods owe their hue to pigments that selectively absorb other wavelengths of light.
Indigo Light: A Transition Zone
Indigo light represents a transition between blue and violet.
Its wavelength range is approximately 420 to 450 nm.
Indigo possesses a higher energy level compared to blue light.
The Subtle Presence of Indigo
Indigo is less commonly encountered in pure form compared to other colors.
Some specialized LEDs can produce indigo light.
Indigo dye, historically derived from plants, is used to color fabrics, most notably denim.
Violet Light: The Shortest Visible Wavelength
Violet light occupies the shortest wavelength end of the visible spectrum.
Its wavelengths stretch approximately from 380 to 420 nm.
This corresponds to the highest energy level within the visible range.
Violet in Our Surroundings
Violet light manifests in various ways.
Violet LEDs are utilized in specialized lighting applications.
Violets and other violet-colored flowers owe their color to pigments that absorb longer wavelengths of light.
Having journeyed through the vibrant wavelengths of Roy G. Biv, understanding each color's place within the spectrum, it's compelling to reflect on the historical foundations that underpin this knowledge. The story of how we came to understand the composition of light itself is a fascinating one, deeply intertwined with the work of a scientific giant.
Newton's Prism: A Historical Perspective
Isaac Newton, a name synonymous with scientific revolution, profoundly shaped our understanding of light and color. His meticulous experimentation with prisms unveiled a truth that had remained hidden for centuries: white light is not a pure entity but a composite of all the colors we perceive.
The Experiment: A Beam of Sunlight and a Triangular Prism
In the late 1660s, Newton conducted a series of now-famous experiments. He darkened his room, allowing only a narrow beam of sunlight to enter through a hole in the window.
This beam was then directed through a triangular glass prism. The result was a dazzling display: the white light was separated into a band of colors, a spectrum arrayed across the opposite wall.
This spectrum, mirroring the hues of a rainbow, demonstrated that white light wasn't colorless, but rather a blend of all the colors of the visible spectrum.
Deconstructing White Light: Evidence of Composition
Newton's genius wasn't merely in observing the spectrum; it lay in his rigorous analysis of the phenomenon. He didn't simply accept that the prism was creating the colors.
To test this, he isolated a single color from the spectrum (say, red) and passed it through another prism. The red light remained red; it wasn't further separated into other colors.
This crucial step proved that the prism wasn't adding something to the light. Instead, it was separating what was already present within the white light.
He concluded that white light is composed of a mixture of different colors, each with a different degree of refrangibility (bending) when passing through the prism. This difference in bending is what separates the colors.
The Significance: A Foundation for Understanding Color
Newton's prism experiment stands as a cornerstone in the science of light and color.
Before Newton, the prevailing view was that color was a modification of white light, somehow altered by the objects it reflected from.
Newton's work overturned this notion. He established that color is an intrinsic property of light itself, determined by its degree of refrangibility and, as we now know, its wavelength.
His findings paved the way for future scientists to explore the properties of light, ultimately leading to our understanding of the electromagnetic spectrum, the wave nature of light, and the complex mechanisms of human color perception. Newton's prism experiment remains a powerful demonstration of scientific inquiry and a pivotal moment in the history of optics.
Having journeyed through the vibrant wavelengths of Roy G. Biv, understanding each color's place within the spectrum, it's compelling to reflect on the historical foundations that underpin this knowledge. The story of how we came to understand the composition of light itself is a fascinating one, deeply intertwined with the work of a scientific giant. With that understanding of the building blocks of light, it's time to consider how these wavelengths translate into the rich tapestry of color we experience every day. The interplay between wavelength, frequency, and our own biology is crucial in unlocking the full story of color perception.
Wavelength, Frequency, and the Science of Color Perception
Light, as we've established, travels in waves. These waves possess two fundamental characteristics: wavelength and frequency.
These properties are inextricably linked, and understanding their relationship is crucial to comprehending how we perceive color.
The Inverse Dance of Wavelength and Frequency
Wavelength and frequency share an inverse relationship. This means that as the wavelength of light decreases, its frequency increases, and vice versa.
Imagine a skipping rope: if you shake it slowly, the waves are long and spread out (long wavelength, low frequency). Shake it faster, and the waves become shorter and more compressed (short wavelength, high frequency).
The same principle applies to light waves. Shorter wavelengths, like those of violet light, have a higher frequency than longer wavelengths, like those of red light.
This difference in frequency translates directly to differences in energy, with higher frequencies carrying more energy.
The Eye's Symphony: How Wavelengths Become Color
The human eye is a remarkable instrument, capable of discerning millions of different colors. This ability stems from specialized cells in the retina called cone cells.
There are three types of cone cells, each most sensitive to a particular range of wavelengths: short (blue), medium (green), and long (red).
When light enters the eye, it stimulates these cone cells to varying degrees, depending on its wavelength composition. For instance, light with a dominant wavelength of 530 nm will primarily stimulate the green cone cells.
The brain then interprets the relative activation levels of these three cone types, translating this information into the sensation of color. This is a remarkable feat of neural processing.
For example, if all three cone types are stimulated equally, we perceive white light. If only the red cone cells are stimulated, we perceive red.
This trichromatic theory of color vision, while simplified, provides a solid foundation for understanding how we perceive color.
When the Symphony Falters: Color Blindness and Deficiency
Sometimes, the delicate machinery of color vision can be disrupted. This can lead to color blindness, more accurately termed color deficiency.
Color deficiency arises when one or more of the cone cell types are either absent or malfunctioning. The most common form is red-green color deficiency, where individuals have difficulty distinguishing between red and green hues.
This occurs because the red or green cone cells are either missing or respond abnormally to light.
Another, less common form is blue-yellow color deficiency.
In rare cases, individuals may have complete color blindness (monochromacy), where they can only see shades of gray due to the absence or non-functionality of all cone cells.
While color deficiency can present challenges, it's important to note that most individuals with the condition adapt well and lead full lives. Their perception of the world is simply different, not inferior.
Having journeyed through the vibrant wavelengths of Roy G. Biv, understanding each color's place within the spectrum, it's compelling to reflect on the historical foundations that underpin this knowledge. The story of how we came to understand the composition of light itself is a fascinating one, deeply intertwined with the work of a scientific giant. With that understanding of the building blocks of light, it's time to consider how these wavelengths translate into the rich tapestry of color we experience every day. The interplay between wavelength, frequency, and our own biology is crucial in unlocking the full story of color perception.
The Impact of Roy G. Biv Wavelengths: Real-World Applications
The seemingly abstract concept of light wavelengths manifests in tangible ways across various disciplines. From the creation of breathtaking art to the analysis of distant stars, the principles underlying Roy G. Biv are not confined to textbooks or laboratories. Understanding these wavelengths unlocks innovative applications in art, physics, technology, and medicine.
Art and Design: Wavelengths as a Palette
Color theory, a cornerstone of art and design, is fundamentally rooted in the physics of light. Artists manipulate pigments and light sources to evoke specific emotions and create visual effects, all based on the wavelengths reflected or emitted.
The strategic use of complementary colors (those opposite each other on the color wheel) intensifies vibrancy because of the way the human eye perceives contrasting wavelengths. A painter might use a dab of red next to green to make both colors appear more vivid.
Furthermore, the perceived temperature of colors influences mood. Warmer colors, with longer wavelengths, like red and orange, often convey energy and excitement. Cooler colors, with shorter wavelengths, like blue and violet, evoke calmness and serenity.
By understanding the nuances of wavelength, artists and designers can craft experiences that resonate deeply with their audience.
Physics and Astronomy: Spectroscopy and Stellar Composition
Spectroscopy, a powerful analytical tool, hinges on analyzing the wavelengths of light emitted or absorbed by matter. In astronomy, this technique reveals the composition, temperature, and motion of celestial objects.
When light from a star passes through a prism (or a spectroscope), it separates into a spectrum of colors, similar to Roy G. Biv. However, this spectrum isn't continuous.
Instead, it contains dark lines, called absorption lines, at specific wavelengths. These lines correspond to elements in the star's atmosphere that absorb light at those particular wavelengths.
By matching these absorption lines to known elemental spectra, scientists can determine which elements are present in the star and their relative abundance. This provides insights into the star's life cycle, its formation, and even the potential for harboring planets.
Technology: Displays, Lighting, and Communication
Wavelengths play a critical role in numerous technologies that permeate our modern lives. From the screens we stare at daily to the light that illuminates our homes, the manipulation of light waves is central.
LED (Light Emitting Diode) displays, for instance, use semiconductors to emit light at specific wavelengths, creating the colors we see on our phones, televisions, and computer monitors. The precision with which these wavelengths are controlled dictates the color accuracy and vibrancy of the display.
In lighting, different wavelengths can impact our well-being. Blue light, emitted by many electronic devices, can suppress melatonin production, disrupting sleep patterns. Conversely, warmer-toned light bulbs, emitting longer wavelengths, are often preferred for creating a relaxing ambiance.
Moreover, fiber optic communication relies on transmitting data as light pulses through glass fibers. The wavelengths used in these systems are carefully chosen to minimize signal loss and maximize data transmission speed.
Medicine: Light Therapy and Imaging
The medical field leverages the power of specific wavelengths for both therapeutic and diagnostic purposes.
Light therapy, also known as phototherapy, uses specific wavelengths of light to treat various conditions. Blue light therapy is commonly used to treat acne by killing bacteria on the skin. Red light therapy is employed to stimulate collagen production and reduce inflammation.
Medical imaging techniques, such as MRI (Magnetic Resonance Imaging) and PET (Positron Emission Tomography), also utilize electromagnetic radiation (albeit outside the visible spectrum) to visualize internal structures and processes within the body. These technologies provide invaluable insights for diagnosing and treating a wide range of illnesses.
Video: Unlock the Rainbow: Roy G. Biv Wavelengths Explained!
FAQs: Roy G. Biv Wavelengths
Have more questions about Roy G. Biv and the science behind the rainbow? Here are some common questions and answers to help you understand the spectrum of visible light.
What exactly are Roy G. Biv wavelengths?
Roy G. Biv is a mnemonic for the colors of the visible light spectrum: Red, Orange, Yellow, Green, Blue, Indigo, and Violet. Each of these colors corresponds to a specific range of roy g biv wavelengths. Red has the longest wavelengths, while violet has the shortest.
Are Roy G. Biv wavelengths the only wavelengths of light that exist?
No, the roy g biv wavelengths only represent the portion of the electromagnetic spectrum that humans can see. There are many other wavelengths, including infrared, ultraviolet, radio waves, and X-rays, that are invisible to the naked eye.
Why do different Roy G. Biv wavelengths appear as different colors?
The different colors we perceive are due to the different frequencies and energies associated with each roy g biv wavelength. Our eyes have specialized cells that are sensitive to these different wavelengths, and our brains interpret these signals as different colors.
Can objects absorb or reflect Roy G. Biv wavelengths, and how does this affect their color?
Yes, objects absorb some roy g biv wavelengths and reflect others. The colors we see are the wavelengths that are reflected back to our eyes. For example, a red apple absorbs most wavelengths but reflects red roy g biv wavelengths.