Heart Anatomy: The Ultimate Guide You Need To Read
A comprehensive understanding of heart anatomy is fundamental for healthcare professionals and anyone interested in cardiovascular health. The American Heart Association emphasizes the importance of knowing the intricate structure of the heart, as it directly impacts function. Cardiac surgeons rely heavily on their detailed anatomical knowledge to perform successful interventions. Furthermore, advancements in echocardiography allow for non-invasive visualization of heart anatomy, providing critical diagnostic information. This guide provides an in-depth exploration of these vital structures, focusing on how each component contributes to the overall function of this remarkable organ.
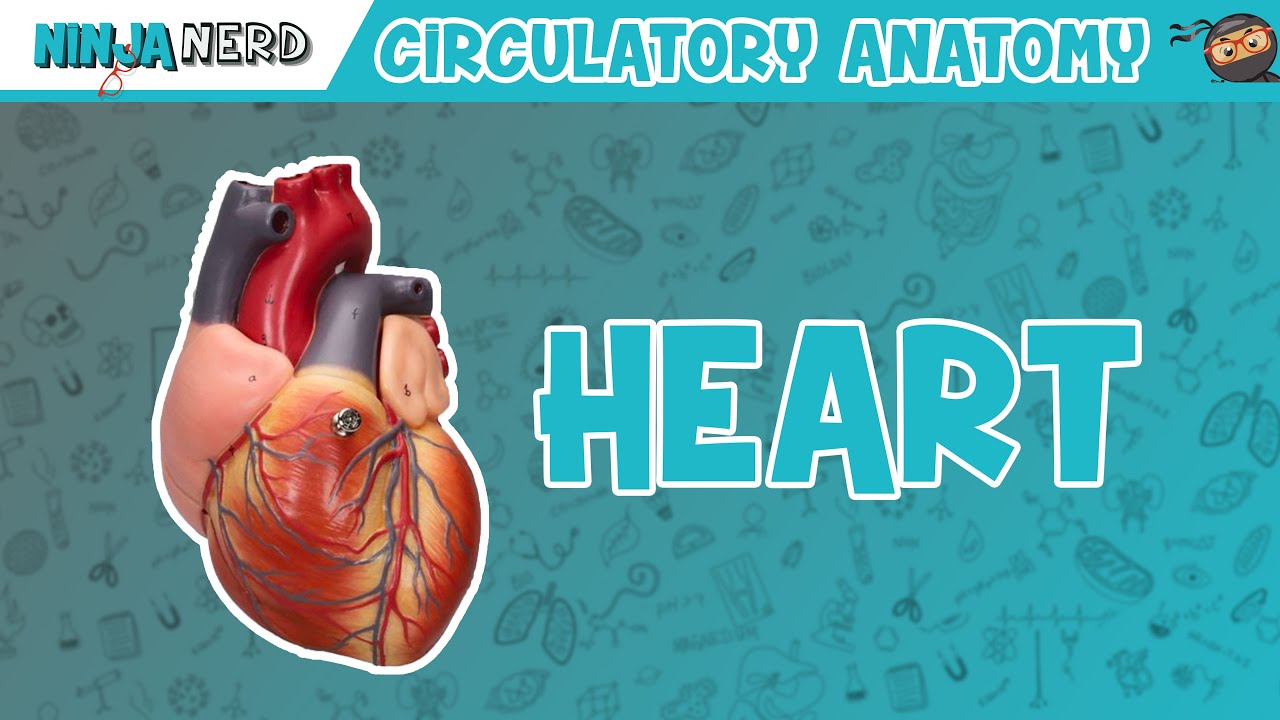
Image taken from the YouTube channel Ninja Nerd , from the video titled Cardiovascular | Anatomy of the Heart | Heart Model .
The human heart, a fist-sized powerhouse nestled within our chests, is far more than just a biological pump. It is the very engine of life, tirelessly working to sustain every cell in our bodies. Understanding its intricate structure, its delicate dance of chambers and valves, is not merely an academic exercise but a crucial step toward appreciating its function and safeguarding our well-being.
Without a functioning heart, the vibrant tapestry of our existence unravels.
The Heart's Central Role: A Circulatory System Overview
At the core of our being lies the circulatory system, and the heart is its undisputed conductor. This intricate network, a vast and complex highway, is responsible for delivering oxygen, nutrients, hormones, and immune cells to every corner of our bodies, while simultaneously whisking away waste products like carbon dioxide.
The heart, as the central pump, propels this life-sustaining fluid throughout the body. With each beat, it orchestrates a symphony of pressure changes, ensuring that blood flows in the correct direction and at the right velocity. The blood travels through arteries, capillaries, and veins, allowing for essential exchange processes to occur.
Without the heart's continuous and rhythmic contractions, this vital transportation system would grind to a halt.
Why Heart Anatomy Matters: Health and Disease Prevention
A foundational understanding of heart anatomy provides the necessary knowledge to comprehend the onset and progression of various cardiovascular diseases. By gaining insights into the structure and function of the heart's chambers, valves, and major vessels, we can better appreciate the mechanisms by which things can go wrong.
For instance, understanding the role of the coronary arteries in supplying oxygen-rich blood to the heart muscle is crucial for grasping the devastating effects of coronary artery disease. Similarly, knowledge of the heart valves and their role in preventing backflow is vital for understanding valve disorders such as stenosis or regurgitation.
In addition to understanding disease, the study of heart anatomy empowers us to make informed lifestyle choices that promote heart health. We are better equipped to adopt preventive measures, such as maintaining a healthy diet, engaging in regular exercise, and avoiding tobacco use.
A Roadmap to Heart Health: Unveiling the Mysteries Within
This guide serves as a comprehensive exploration of the heart's anatomy, designed to illuminate its inner workings and empower you to make informed decisions about your health. We will embark on a detailed journey through the heart's chambers, valves, and major blood vessels, examining their individual roles and how they work together in perfect harmony.
We'll delve into the microscopic level, exploring the structure and function of cardiomyocytes, the heart's remarkable muscle cells. We will also investigate the heart's electrical conduction system, the intricate network that coordinates each heartbeat with remarkable precision.
By the end of this guide, you will have gained a profound appreciation for the heart's intricate design and its central role in sustaining life. This knowledge will not only enhance your understanding of cardiovascular health but also empower you to take proactive steps toward protecting your heart and overall well-being.
The Four Chambers: A Detailed Tour Inside
The heart's remarkable efficiency stems from its division into four distinct chambers, each meticulously designed to perform a specific role in the circulatory process. These chambers – the right atrium, left atrium, right ventricle, and left ventricle – work in perfect synchrony to ensure a continuous and unidirectional flow of blood. Understanding the individual characteristics and functions of these chambers is fundamental to grasping the overall mechanics of the heart.
Orchestrating Blood Flow: The Chamber's Roles
Each chamber plays a crucial role in the intricate dance of blood flow through the heart. The atria act as receiving chambers, while the ventricles serve as powerful pumps. Understanding their sequential actions is key to appreciating the elegance of cardiac physiology.
Right Atrium: The Gateway for Deoxygenated Blood
The right atrium stands as the entry point for deoxygenated blood returning from the body. This chamber receives blood via two major veins: the superior vena cava, which drains blood from the upper body, and the inferior vena cava, which carries blood from the lower body. The coronary sinus, which drains blood from the heart muscle itself, also empties into the right atrium.
The right atrium's primary function is to collect this deoxygenated blood and then propel it into the right ventricle. The pressure generated by the atrial contraction assists in completely filling the ventricle.
Left Atrium: Receiving Oxygenated Blood from the Lungs
In stark contrast to its right-sided counterpart, the left atrium receives oxygenated blood returning from the lungs. This oxygen-rich blood arrives via the pulmonary veins, typically four in number, which connect the lungs to the left atrium.
The left atrium, much like the right atrium, acts as a holding chamber and pump, directing the oxygenated blood into the left ventricle. This process ensures that the left ventricle is fully primed to deliver oxygenated blood to the rest of the body.
Right Ventricle: Delivering Blood to the Lungs
The right ventricle is responsible for pumping deoxygenated blood received from the right atrium to the lungs for oxygenation. This crucial step in the circulatory process is achieved via the pulmonary artery, which branches into the left and right pulmonary arteries, leading to each lung respectively.
Notably, the walls of the right ventricle are thinner compared to the left ventricle. This difference in thickness reflects the lower pressure required to pump blood to the nearby lungs, as opposed to the systemic circulation.
Left Ventricle: Powering Systemic Circulation
The left ventricle is the heart's most powerful chamber, responsible for pumping oxygenated blood to the entire body. This is achieved through the aorta, the largest artery in the body, which branches out to supply blood to all organ systems.
Reflecting its demanding task, the left ventricle possesses the thickest walls of all four chambers. This robust musculature enables the left ventricle to generate the high pressures required to overcome systemic resistance and ensure adequate blood flow to all tissues.
The Septa: Dividing Walls for Efficient Flow
The interatrial septum separates the right and left atria, while the interventricular septum divides the right and left ventricles. These septa are essential for preventing the mixing of oxygenated and deoxygenated blood, thereby ensuring that the body receives fully oxygenated blood.
These septa are composed of cardiac muscle tissue and are vital for maintaining the pressure differences between the chambers, which are critical for proper blood flow direction. Any defects in these septa can lead to congenital heart conditions, where oxygen-rich and oxygen-poor blood mix, compromising the efficiency of the circulatory system.
The Heart Valves: Gatekeepers of Blood Flow
With the heart chambers now clearly defined, the next critical component in the heart's architecture are the valves. These ingenious structures ensure that blood flows in only one direction, preventing backflow and maintaining the circulatory system's efficiency. The four valves – tricuspid, mitral (bicuspid), pulmonary, and aortic – act as precisely timed gates, opening and closing in perfect coordination with the heart's contractions.
Preventing Backflow: The Valve's Primary Role
The primary function of the heart valves is to maintain unidirectional blood flow. This prevents the retrograde movement of blood, ensuring that oxygenated blood reaches the tissues and organs that need it.
Valves achieve this through their unique structure and synchronized action. Each valve consists of leaflets (or cusps) that open to allow blood to flow forward and then snap shut to prevent backflow. The pressure gradient created by the heart's contractions governs the opening and closing of these leaflets.
When the pressure behind the valve is greater, it opens. Conversely, when the pressure in front of the valve is greater, it closes, preventing backflow. This seemingly simple mechanism is vital for efficient circulation.
The Four Valves: Location and Function
Each of the four heart valves is strategically located to control blood flow between specific chambers or vessels. Understanding their individual roles provides a deeper appreciation of their critical function.
Tricuspid Valve: Guarding the Right Atrium and Ventricle
The tricuspid valve is located between the right atrium and the right ventricle. As the name implies, it has three leaflets, or cusps.
This valve opens to allow deoxygenated blood to flow from the right atrium into the right ventricle during diastole (the heart's relaxation phase). During systole (contraction), the tricuspid valve closes, preventing backflow of blood into the right atrium as the right ventricle pumps blood to the lungs.
Mitral (Bicuspid) Valve: The Left Side's Guardian
The mitral valve, also known as the bicuspid valve, is situated between the left atrium and the left ventricle. Unlike the tricuspid valve, it has only two leaflets.
It opens during diastole to allow oxygenated blood to flow from the left atrium into the left ventricle. During systole, the mitral valve closes tightly to prevent backflow as the left ventricle forcefully pumps oxygenated blood into the aorta for distribution throughout the body. Proper mitral valve function is crucial, given the high pressures generated by the left ventricle.
Pulmonary Valve: Directing Flow to the Lungs
The pulmonary valve is located between the right ventricle and the pulmonary artery. This valve has three leaflets arranged in a semi-lunar fashion.
It opens during systole, allowing deoxygenated blood to flow from the right ventricle into the pulmonary artery, which then carries the blood to the lungs for oxygenation. During diastole, the pulmonary valve closes to prevent backflow of blood from the pulmonary artery back into the right ventricle.
Aortic Valve: The Gateway to Systemic Circulation
The aortic valve sits between the left ventricle and the aorta, the body's largest artery. Similar to the pulmonary valve, it also has three semi-lunar cusps.
During systole, the aortic valve opens, allowing oxygenated blood to surge from the left ventricle into the aorta for distribution to the systemic circulation. In diastole, the aortic valve closes to prevent backflow of blood from the aorta back into the left ventricle, ensuring that the systemic circulation receives an adequate supply of oxygenated blood.
Chordae Tendineae and Papillary Muscles: Supporting Valve Function
While the leaflets themselves are crucial to valve function, their integrity depends on the support provided by the chordae tendineae and papillary muscles. These structures work together to prevent the valves from prolapsing, or bulging backward, into the atria during ventricular contraction.
Chordae tendineae are strong, fibrous cords that connect the valve leaflets to the papillary muscles, which are cone-shaped muscular projections arising from the ventricular walls.
When the ventricles contract, the papillary muscles also contract, pulling on the chordae tendineae. This tension prevents the valve leaflets from inverting into the atria due to the high pressure generated during ventricular systole. Without the support of the chordae tendineae and papillary muscles, the valves would become incompetent, leading to backflow and circulatory compromise.
The precise orchestration of the heart valves ensures blood moves efficiently through the chambers, but the journey doesn't end there. The heart's functionality also hinges on a network of major blood vessels that act as conduits, delivering life-sustaining oxygen and nutrients, and removing waste products. Understanding these vessels is paramount to grasping the complete picture of cardiovascular function.
Major Blood Vessels: The Heart's Lifelines
The heart is not a closed system. It relies on a complex network of blood vessels to receive blood, oxygenate it, and distribute it throughout the body. These major vessels are the highways of the circulatory system, ensuring continuous and efficient blood flow.
The Aorta: The Body's Oxygen Supply Line
The aorta, the largest artery in the body, originates directly from the left ventricle. It is the primary conduit for oxygenated blood heading out to systemic circulation.
Journey of Oxygenated Blood
The aorta arches upwards (the aortic arch) and then descends through the chest and abdomen. Along its course, major arteries branch off, delivering oxygen-rich blood to all organs and tissues.
The Pulmonary Artery: Deoxygenated Blood to the Lungs
In stark contrast to the aorta, the pulmonary artery carries deoxygenated blood. It originates from the right ventricle and is the sole artery in the body to carry oxygen-poor blood.
Pathway to Oxygenation
The pulmonary artery bifurcates into the left and right pulmonary arteries. These arteries then travel to the respective lungs, where the blood releases carbon dioxide and picks up oxygen.
Pulmonary Veins: Oxygenated Blood Returns
The pulmonary veins are responsible for returning oxygenated blood from the lungs to the heart. Uniquely, these are the only veins in the body carrying oxygen-rich blood.
Delivering to the Left Atrium
Typically, there are four pulmonary veins: two from the left lung and two from the right lung. These veins empty into the left atrium, completing the pulmonary circulation loop.
Vena Cavae: Returning Deoxygenated Blood
The superior vena cava (SVC) and the inferior vena cava (IVC) are the two major veins that return deoxygenated blood from the body to the right atrium.
Superior Vena Cava
The SVC drains blood from the upper body, including the head, neck, and upper limbs.
Inferior Vena Cava
The IVC drains blood from the lower body, including the abdomen, pelvis, and lower limbs. These vessels are crucial for bringing blood back to the heart for re-oxygenation.
Coronary Arteries: Nourishing the Heart Muscle
While the heart pumps blood to the entire body, it also requires its own dedicated blood supply. This crucial task is performed by the coronary arteries. These arteries branch off from the aorta near its origin.
Supplying the Myocardium
The left and right coronary arteries, along with their branches, provide oxygen-rich blood to the heart muscle (myocardium), ensuring its continuous function.
Coronary Veins: Draining the Heart Muscle
Just as the heart needs a supply of oxygenated blood, it also requires a way to remove deoxygenated blood and metabolic waste products. This is the role of the coronary veins.
Emptying into the Right Atrium
These veins collect the deoxygenated blood from the heart muscle and eventually drain into the right atrium via the coronary sinus.
Facilitating Overall Blood Flow
These major blood vessels work in concert, forming an intricate network that sustains life. The coordinated action of these vessels ensures that oxygenated blood reaches every cell in the body, while deoxygenated blood is efficiently transported to the lungs for replenishment. Disruptions in the function of any of these vessels can have severe consequences on overall health.
Layers of the Heart Wall: Protection and Power
With the heart's chambers and vital vessels now brought to light, it's time to consider the very structure that houses and protects them: the heart wall itself. This wall is not a single entity, but a sophisticated composite of three distinct layers, each contributing uniquely to the organ's resilience and functionality. From the outermost protective sac to the innermost lining, these layers work in concert to ensure the heart's ceaseless operation.
The Pericardium: The Heart's Protective Shield
The pericardium, the outermost layer, serves as the heart's primary defense against friction and infection. It is a double-layered sac that not only encases the heart but also provides crucial physical support.
Parietal and Visceral Layers
The pericardium consists of two main layers: the parietal pericardium and the visceral pericardium. The parietal pericardium is the outer layer, forming a sac that is attached to the mediastinum.
The visceral pericardium, also known as the epicardium, is directly adhered to the heart's surface.
The Pericardial Space
Between the parietal and visceral layers lies the pericardial space, a cavity filled with a small amount of serous fluid. This fluid acts as a lubricant, minimizing friction as the heart beats within the pericardial sac. This lubrication is critical to prevent inflammation and damage during the heart's constant motion.
The Myocardium: The Engine of Contraction
Beneath the pericardium lies the myocardium, the thickest and most muscular layer of the heart wall. This is the heart's workhorse, responsible for the powerful contractions that pump blood throughout the body.
Cardiomyocytes: The Muscle Cells
The myocardium is primarily composed of cardiomyocytes, specialized cardiac muscle cells. These cells are uniquely structured with interlocking fibers that allow for efficient and coordinated contractions.
Cardiomyocytes are rich in mitochondria, providing the energy needed for continuous activity. Intercalated discs connect adjacent cardiomyocytes, enabling rapid electrical impulse transmission for synchronized contractions.
The Endocardium: The Inner Sanctum
The endocardium, the innermost layer of the heart wall, lines the heart chambers and covers the heart valves. This thin, smooth layer is in direct contact with the blood flowing through the heart.
Structure and Function
The endocardium is composed of endothelial cells, similar to those lining blood vessels. This continuity helps minimize friction and prevents blood clot formation within the heart.
The endocardium also plays a role in regulating heart function by releasing substances that can influence myocardial contractility and vascular tone. Its smooth surface ensures seamless blood flow, preventing damage to blood cells and maintaining efficient cardiac function.
Beneath the muscular layers and protective sheaths lies a different kind of network, one that doesn't rely on physical strength but on precisely timed electrical signals. This network, the cardiac conduction system, is the heart's intrinsic control system, the conductor of its rhythmic symphony. It’s what allows the heart to beat in a coordinated fashion, ensuring efficient blood delivery to the body.
The Cardiac Conduction System: The Heart's Electrical Network
The cardiac conduction system is a specialized network of cells that generate and transmit electrical impulses throughout the heart. This intricate system is responsible for coordinating the contraction of the heart's chambers, ensuring that blood is pumped efficiently throughout the body. Without this system, the heart's contractions would be disorganized and ineffective.
The Role of Electrical Signals in Heart Coordination
The heart's ability to function as a pump depends on the precise timing and coordination of its contractions. The atria must contract before the ventricles, and the ventricles must contract in a synchronized manner to effectively eject blood into the pulmonary artery and aorta.
This coordination is achieved through the cardiac conduction system, which generates electrical impulses and transmits them rapidly throughout the heart. These electrical impulses trigger the contraction of cardiomyocytes, the heart muscle cells.
Components of the Conduction System
The cardiac conduction system consists of several key components, each playing a crucial role in the generation and transmission of electrical signals:
- Sinoatrial (SA) Node: The heart's natural pacemaker, initiating the heartbeat.
- Atrioventricular (AV) Node: Delays the electrical signal, allowing the atria to contract before the ventricles.
- Bundle of His: Transmits the electrical signal from the AV node to the ventricles.
- Purkinje Fibers: Distribute the electrical signal throughout the ventricles, ensuring coordinated contraction.
Sinoatrial (SA) Node: The Heart's Natural Pacemaker
Located in the right atrium, the sinoatrial (SA) node is the heart's primary pacemaker. It spontaneously generates electrical impulses at a rate of 60 to 100 beats per minute under normal conditions.
These impulses spread throughout the atria, causing them to contract. The SA node's ability to generate regular electrical impulses is essential for maintaining a consistent heart rhythm.
Atrioventricular (AV) Node: The Gatekeeper
The electrical impulses generated by the SA node travel to the atrioventricular (AV) node, located in the septum between the atria and ventricles. The AV node acts as a gatekeeper, delaying the electrical signal before it is transmitted to the ventricles.
This delay is crucial because it allows the atria to finish contracting and empty their contents into the ventricles before ventricular contraction begins.
Bundle of His: Bridging the Gap
From the AV node, the electrical signal travels down the bundle of His, a bundle of specialized fibers that runs through the interventricular septum.
The bundle of His divides into left and right bundle branches, which carry the electrical signal to the left and right ventricles, respectively.
Purkinje Fibers: Spreading the Word
The bundle branches further divide into a network of Purkinje fibers, which spread throughout the ventricular myocardium. These fibers rapidly distribute the electrical signal, ensuring that the ventricles contract in a coordinated and synchronized manner. This coordinated contraction is essential for efficient ejection of blood from the ventricles.
In summary, the cardiac conduction system functions as a precisely orchestrated electrical network, ensuring that the heart beats in a coordinated and efficient manner. Understanding this system is essential for appreciating the heart's remarkable ability to sustain life.
Beneath the muscular layers and protective sheaths lies a different kind of network, one that doesn't rely on physical strength but on precisely timed electrical signals. This network, the cardiac conduction system, is the heart's intrinsic control system, the conductor of its rhythmic symphony. It’s what allows the heart to beat in a coordinated fashion, ensuring efficient blood delivery to the body.
Now, let's zoom out from the intricate electrical circuits and consider the bigger picture. The coordinated sequence of events that constitute a single heartbeat – the cardiac cycle – is a marvel of physiological engineering.
The Cardiac Cycle: A Rhythmic Dance of Contraction and Relaxation
The cardiac cycle is the sequence of events that occurs during one complete heartbeat. It encompasses all the changes within the heart, including pressure changes, valve opening and closing, and blood flow.
Understanding this cycle is crucial to appreciating how the heart functions as a pump. The cardiac cycle is comprised of two primary phases: systole (contraction) and diastole (relaxation).
Systole: The Contraction Phase
Systole refers to the phase of the cardiac cycle when the heart muscle contracts. This contraction generates pressure, which propels blood out of the heart and into the circulation.
Systole can be further divided into two sub-phases: atrial systole and ventricular systole.
Atrial Systole
Atrial systole is the contraction of the atria. This contraction forces the remaining blood from the atria into the ventricles, completing the ventricular filling process.
While atrial systole contributes a relatively small amount to overall ventricular filling, it's crucial in individuals with certain heart conditions where ventricular filling may be compromised.
Ventricular Systole
Ventricular systole is the powerful contraction of the ventricles. This phase is responsible for ejecting the majority of the blood volume into the pulmonary artery (from the right ventricle) and the aorta (from the left ventricle).
As the ventricles contract, the pressure inside them rises sharply. When this pressure exceeds the pressure in the pulmonary artery and aorta, the pulmonary and aortic valves open. Blood rushes out of the ventricles.
Diastole: The Relaxation Phase
Diastole is the phase of the cardiac cycle when the heart muscle relaxes. During diastole, the heart chambers fill with blood in preparation for the next contraction.
Like systole, diastole can also be divided into sub-phases: ventricular diastole and atrial diastole.
Ventricular Diastole
Ventricular diastole begins with the relaxation of the ventricles. As the ventricles relax, the pressure inside them decreases.
When ventricular pressure falls below the pressure in the pulmonary artery and aorta, the pulmonary and aortic valves close. This prevents backflow of blood into the ventricles.
As ventricular pressure continues to decrease, it eventually falls below the pressure in the atria. This causes the tricuspid and mitral valves to open, allowing blood to flow from the atria into the ventricles (ventricular filling).
Atrial Diastole
Atrial diastole occurs concurrently with ventricular filling. The atria are relaxed, allowing them to fill with blood returning from the body (right atrium) and the lungs (left atrium).
This continuous filling of the atria creates a pressure gradient that facilitates the passive flow of blood into the ventricles once the tricuspid and mitral valves open.
The Role of Pressure Changes
The cyclical changes in pressure within the heart chambers and major blood vessels are the driving force behind the cardiac cycle. These pressure changes dictate the opening and closing of the heart valves.
They also control the direction of blood flow.
Pressure Gradients and Valve Function
The heart valves open and close in response to pressure gradients. Blood flows from an area of higher pressure to an area of lower pressure.
When the pressure in the atrium is higher than the pressure in the ventricle, the atrioventricular valve (tricuspid or mitral) opens, allowing blood to flow from the atrium to the ventricle.
Conversely, when the pressure in the ventricle is higher than the pressure in the atrium, the atrioventricular valve closes, preventing backflow of blood into the atrium. A similar principle governs the opening and closing of the semilunar valves (pulmonary and aortic).
Pressure and Blood Flow
The pressure generated during systole is essential for propelling blood throughout the circulatory system. The left ventricle, with its thicker walls, generates a significantly higher pressure than the right ventricle.
This higher pressure is necessary to overcome the resistance of the systemic circulation and deliver oxygenated blood to all the tissues of the body. The right ventricle only needs to pump blood to the lungs, which have a much lower resistance.
By understanding the cardiac cycle and how pressure changes orchestrate its events, we gain a deeper appreciation for the elegance and efficiency of the heart as a pump. It's a delicate and precisely timed sequence that ensures the continuous delivery of life-sustaining blood to every cell in the body.
Understanding the Electrocardiogram (ECG/EKG): A Window into Heart Function
The coordinated dance of contraction and relaxation within the cardiac cycle creates a rhythm that can be visualized and interpreted. This is where the electrocardiogram (ECG), also known as an EKG, becomes an invaluable tool. It's not an invasive procedure, but rather a sophisticated method of eavesdropping on the heart's electrical activity from the surface of the body.
An ECG provides a graphical representation of the heart's electrical impulses as they travel through the myocardium. It offers a non-invasive snapshot of cardiac function, illuminating the sequence of atrial and ventricular depolarization and repolarization. It allows clinicians to identify abnormalities in heart rate, rhythm, and conduction, offering crucial insights into a patient's cardiovascular health.
The Basics of ECG/EKG: Deciphering the Waveforms
An ECG tracing isn't just a random squiggle; it's a carefully orchestrated sequence of waveforms, each corresponding to a specific event in the cardiac cycle. These deflections, both upward and downward, represent the electrical activity of the heart, captured by electrodes placed on the skin.
The key components of a standard ECG waveform include the P wave, QRS complex, and T wave. Each of these waves represents a different part of the cardiac cycle:
- The P wave represents atrial depolarization, the electrical activation of the atria leading to their contraction.
- The QRS complex signifies ventricular depolarization, the electrical activation of the ventricles which triggers their contraction. Its shape and duration are carefully scrutinized.
- The T wave reflects ventricular repolarization, the return of the ventricles to their resting electrical state.
The intervals between these waves, such as the PR interval and the QT interval, are also critically important. They represent the time taken for electrical impulses to travel between different parts of the heart.
ECG/EKG in Diagnosis: A Powerful Diagnostic Tool
The ECG is a cornerstone in the diagnosis and management of a wide range of cardiac conditions. It offers a wealth of information that can help clinicians identify problems early and guide treatment decisions. From detecting subtle arrhythmias to recognizing the telltale signs of a heart attack, the ECG is an indispensable tool.
- Arrhythmias: An ECG can detect irregularities in heart rhythm, such as atrial fibrillation, ventricular tachycardia, and bradycardia. These rhythm disturbances can significantly impact cardiac output and overall health.
- Myocardial Ischemia and Infarction: Changes in the ST segment and T wave can indicate myocardial ischemia (reduced blood flow to the heart muscle) or infarction (heart attack). The ECG can help pinpoint the location and extent of damage.
- Conduction Abnormalities: Problems with the conduction system, such as heart blocks, can be identified by prolonged intervals or abnormal waveforms on the ECG.
- Electrolyte Imbalances: Severe imbalances of electrolytes like potassium, calcium, and magnesium can have notable changes on ECG wave morphology.
- Hypertrophy: Enlargement of the atria or ventricles can manifest as increased amplitudes in the respective waves.
The interpretation of an ECG requires expertise and careful consideration of the patient's clinical context. However, its ability to provide real-time information about the heart's electrical activity makes it an essential tool for cardiologists and other healthcare professionals. It provides an immediate understanding of heart function, enabling a rapid diagnosis and treatment plan.
Cardiomyocytes: The Heart's Workhorses
The intricate dance of electrical signals across the heart, so clearly visualized on an ECG, ultimately translates into a mechanical action: the powerful contraction that pumps life-sustaining blood throughout the body. This critical function is performed by specialized cells known as cardiomyocytes, the heart's tireless workhorses. Understanding their unique structure and function is crucial to appreciating the heart's overall capability.
The Unique Cell Structure of Cardiomyocytes
Cardiomyocytes, or cardiac muscle cells, are highly specialized cells designed for efficient and continuous contraction. Unlike skeletal muscle cells, cardiomyocytes are mononucleated, meaning they possess only one nucleus.
This is a key distinction related to their energy demands and resistance to fatigue.
They are also much shorter and wider than skeletal muscle fibers.
Intercalated Discs: The Key to Coordinated Contraction
One of the most distinctive features of cardiomyocytes is the presence of intercalated discs. These specialized junctions connect adjacent cells, forming a functional syncytium.
Intercalated discs are complex structures comprising desmosomes, which provide strong physical adhesion, and gap junctions.
Gap junctions are particularly important, as they allow for the rapid passage of ions and electrical signals between cells.
This enables the near-instantaneous spread of depolarization, ensuring that the heart muscle contracts in a coordinated and efficient manner.
Without these specialized connections, the heart would not be able to pump blood effectively.
Abundant Mitochondria: Powering the Heart's Relentless Beat
Cardiomyocytes are exceptionally rich in mitochondria, the cellular powerhouses responsible for generating ATP, the primary energy currency of the cell.
This high mitochondrial content reflects the heart's constant and enormous energy demands.
Unlike other muscles, the heart cannot function for long without sufficient oxygen.
Cardiomyocytes rely almost exclusively on aerobic metabolism to produce ATP, using fatty acids and glucose as fuel.
This constant energy supply is crucial for maintaining the continuous cycle of contraction and relaxation that characterizes the heartbeat.
How Cardiomyocytes Function to Enable Heart Contractions
The function of cardiomyocytes is intricately linked to their structure. They are highly organized to efficiently convert electrical signals into mechanical force.
The Role of Sarcomeres and Myofilaments
Like skeletal muscle cells, cardiomyocytes contain sarcomeres, the basic contractile units of muscle tissue. Sarcomeres are composed of organized arrangements of actin and myosin filaments, the proteins responsible for muscle contraction.
The sliding filament mechanism, where actin and myosin filaments slide past each other, shortening the sarcomere, drives cardiomyocyte contraction.
This process is triggered by the influx of calcium ions into the cytoplasm of the cell, initiated by the electrical impulse traveling through the heart.
The coordinated shortening of countless sarcomeres within each cardiomyocyte generates the force necessary for the heart to contract and pump blood.
Excitation-Contraction Coupling: From Electrical Signal to Mechanical Action
The process by which an electrical signal initiates muscle contraction is known as excitation-contraction coupling.
In cardiomyocytes, this process involves a complex interplay of ion channels, calcium release, and protein interactions.
When an electrical impulse, generated by the cardiac conduction system, reaches a cardiomyocyte, it causes the cell membrane to depolarize.
This depolarization triggers the opening of voltage-gated calcium channels, allowing calcium ions to enter the cell from the extracellular space.
This influx of calcium further stimulates the release of calcium from the sarcoplasmic reticulum, an intracellular calcium store.
The increased intracellular calcium concentration then binds to troponin, a protein associated with actin filaments, initiating the sliding filament mechanism and ultimately leading to contraction.
Relaxation: Returning to the Resting State
Following contraction, calcium ions are actively pumped back into the sarcoplasmic reticulum or out of the cell, decreasing the intracellular calcium concentration.
This allows troponin to return to its original position, blocking the interaction between actin and myosin, and causing the sarcomere to lengthen, leading to relaxation.
The efficient removal of calcium is crucial for allowing the heart muscle to relax completely between beats, ensuring proper filling of the heart chambers with blood.
The delicate balance between calcium influx and efflux is essential for regulating the strength and duration of each heartbeat.
The coordinated function of these specialized cells is what sustains life, tirelessly pumping blood to every corner of the body.
Cardiomyocytes are exquisitely designed for their tireless task, their structure optimized for efficient energy production and coordinated contraction. But even the most robust systems are susceptible to failure.
Common Heart Conditions and Anatomical Abnormalities: When Things Go Wrong
The heart, despite its remarkable resilience, is vulnerable to a range of conditions that can compromise its function. A foundational understanding of cardiac anatomy is crucial not only for appreciating the heart's normal operation but also for comprehending the mechanisms underlying various heart diseases. Let's explore some common cardiac conditions and how anatomical knowledge illuminates their pathology.
Coronary Artery Disease (CAD): A Plumbing Problem
Coronary Artery Disease (CAD) is one of the most prevalent heart conditions, often stemming from atherosclerosis, the buildup of plaque inside the coronary arteries. These arteries, as we've discussed, are responsible for supplying the heart muscle itself with oxygen-rich blood.
When plaque accumulates, it narrows the arteries, restricting blood flow to the myocardium. This ischemia (oxygen deprivation) can lead to chest pain (angina) or, in severe cases, a heart attack (myocardial infarction), where a portion of the heart muscle dies due to prolonged lack of oxygen.
Understanding the location and distribution of the coronary arteries is vital in diagnosing and treating CAD. An obstruction in the left anterior descending artery (LAD), for example, can have a more significant impact than a blockage in a smaller branch, due to the larger area of myocardium it supplies.
Heart Failure: When the Pump Weakens
Heart failure doesn't mean the heart has stopped working; rather, it signifies that the heart isn't pumping blood as efficiently as it should to meet the body's needs. This can result from various underlying conditions, including CAD, high blood pressure, valve disorders, and cardiomyopathy (disease of the heart muscle).
Heart failure can affect either the left or right side of the heart, or both. Left-sided heart failure often leads to fluid buildup in the lungs (pulmonary edema), causing shortness of breath. Right-sided heart failure can cause swelling in the legs and ankles (peripheral edema) and fluid accumulation in the abdomen (ascites).
Knowledge of the heart's chambers and their respective functions is essential for understanding the different types and consequences of heart failure. For instance, left ventricular hypertrophy (thickening of the left ventricle wall) can impair its ability to relax and fill properly, leading to diastolic heart failure.
Arrhythmias: Disruptions in the Electrical Symphony
Arrhythmias are irregularities in the heart's rhythm, caused by problems with the heart's electrical conduction system. These can manifest as heartbeats that are too fast (tachycardia), too slow (bradycardia), or irregular.
Understanding the location and function of the sinoatrial (SA) node, atrioventricular (AV) node, Bundle of His, and Purkinje fibers is critical for diagnosing and treating arrhythmias. For example, atrial fibrillation, a common arrhythmia, involves rapid, chaotic electrical activity in the atria, disrupting the coordinated contraction of these chambers.
An ECG (electrocardiogram), which records the heart's electrical activity, is a key diagnostic tool for identifying arrhythmias. Knowledge of the normal ECG waveform and its relationship to the cardiac cycle allows clinicians to pinpoint the source and type of arrhythmia.
Valve Disorders: Leaky or Stiff Gatekeepers
The heart valves, as discussed, ensure unidirectional blood flow through the heart. Valve disorders occur when these valves don't open or close properly, disrupting the smooth flow of blood.
Stenosis refers to a valve that is narrowed and doesn't open fully, restricting blood flow. Regurgitation (or insufficiency) occurs when a valve doesn't close tightly, allowing blood to leak backward.
Valve disorders can affect any of the four heart valves: the tricuspid, mitral, pulmonary, and aortic valves. Mitral valve prolapse, for example, is a condition in which the mitral valve leaflets bulge back into the left atrium during ventricular contraction. Severe valve disorders may require surgical repair or replacement.
Understanding the anatomical location and function of each valve, along with the chordae tendineae and papillary muscles that support them, is essential for diagnosing and treating valve disorders.
Congenital Heart Defects: When Development Goes Astray
Congenital heart defects are structural abnormalities of the heart that are present at birth. These defects can range from minor to life-threatening and can involve the heart's chambers, valves, or major blood vessels.
Examples of congenital heart defects include:
- Ventricular Septal Defect (VSD): A hole in the wall separating the ventricles.
- Atrial Septal Defect (ASD): A hole in the wall separating the atria.
- Tetralogy of Fallot: A complex defect involving four abnormalities, including a VSD, pulmonary stenosis, and an overriding aorta.
Detailed knowledge of normal heart development and anatomy is paramount for understanding and managing congenital heart defects. Advances in surgical techniques have significantly improved the outcomes for children born with these conditions.
Anatomical Knowledge: The Cornerstone of Understanding
In conclusion, understanding the intricacies of heart anatomy is not merely an academic exercise; it is the foundation upon which we comprehend the mechanisms of heart disease. From the plumbing problems of coronary artery disease to the electrical disruptions of arrhythmias and the structural abnormalities of congenital heart defects, a solid grasp of cardiac anatomy provides invaluable insights into the causes, consequences, and treatment of these conditions.
Video: Heart Anatomy: The Ultimate Guide You Need To Read
FAQs: Understanding Heart Anatomy
Hopefully, this FAQ section will clear up any lingering questions you might have after reading our ultimate guide to heart anatomy.
What are the four chambers of the heart?
The heart has four chambers: the right atrium, the right ventricle, the left atrium, and the left ventricle. These chambers work together to pump blood throughout the body. A good understanding of these chambers is essential to grasping heart anatomy.
What's the function of heart valves?
Heart valves act as one-way doors, ensuring blood flows in the correct direction through the heart. They open and close in coordination with the heart's contractions. Proper function of these valves is critical for efficient blood circulation, and understanding them is a key element of heart anatomy.
What's the difference between arteries and veins related to the heart?
Arteries carry oxygenated blood away from the heart to the rest of the body, while veins carry deoxygenated blood back to the heart. The pulmonary artery is the exception, carrying deoxygenated blood to the lungs. Learning how arteries and veins are connected is an essential part of learning heart anatomy.
What is the pericardium, and why is it important?
The pericardium is a double-layered sac surrounding the heart. It provides protection, reduces friction as the heart beats, and helps to anchor the heart within the chest cavity. Many heart conditions, such as pericarditis, involve issues with this structure of heart anatomy.
Hopefully, this deep dive into heart anatomy has been helpful! It's a complex but fascinating subject, and understanding the basics can really empower you to take care of your cardiovascular health. Keep exploring the amazing world of heart anatomy, and don't hesitate to ask your doctor any questions you might have!