Solar Granules: What Are They and Why Do They Matter?
The Sun, our star, exhibits fascinating features, and understanding what are solar granules? is crucial for comprehending its dynamics. The visible surface of the Sun, known as the Photosphere, displays these granular structures. NASA's solar observatories provide invaluable data that allow scientists to analyze solar granules. Specifically, the study of convection, a key process studied by solar physicists, directly relates to the formation and behavior of these granules. Studying what are solar granules? gives vital insights to scientists and experts, regarding heat production and radiation output within our solar system.
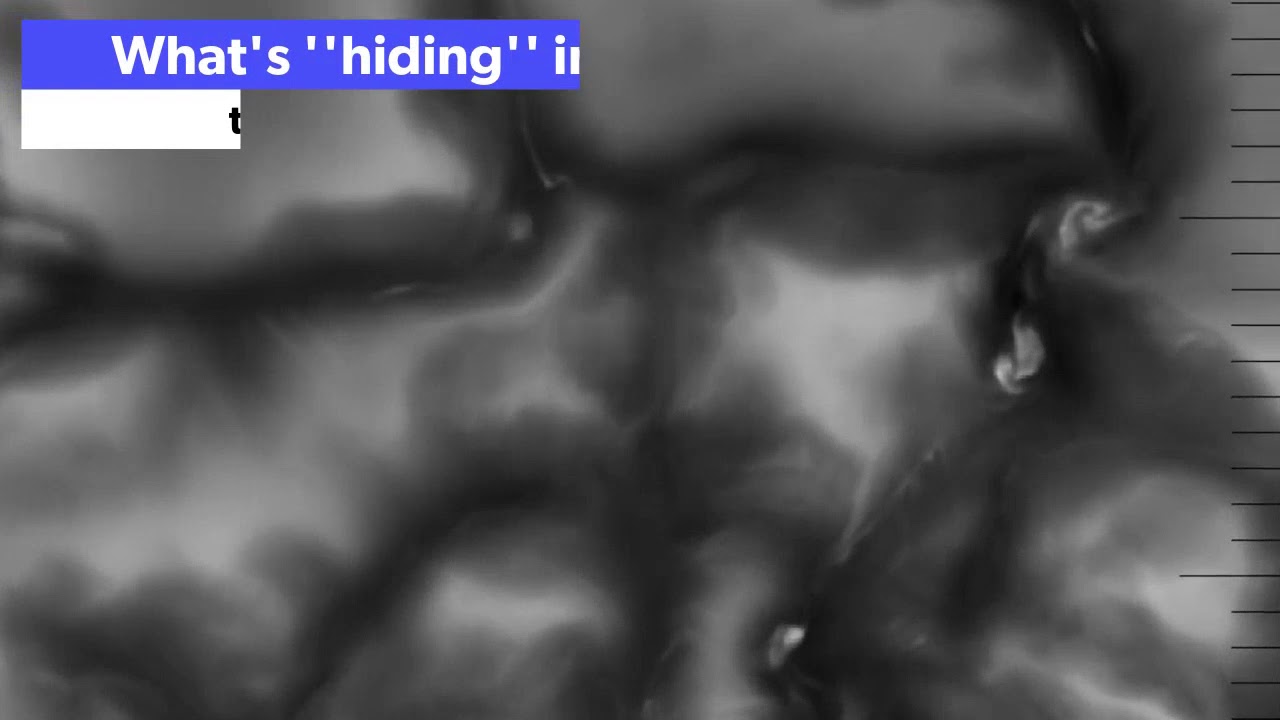
Image taken from the YouTube channel National Solar Observatory , from the video titled Inouye Solar Telescope and Solar Granulation .
The Sun, our solar system's powerhouse, is far from a static, featureless ball of light. It is a seething, dynamic furnace, a colossal sphere of plasma undergoing constant change and activity. To truly understand its influence on our planet and the broader cosmos, we must delve into the intricate details of its surface.
One of the most striking features of the Sun's visible surface, the photosphere, is its granular appearance. These granules, like grains of rice scattered across the solar disk, are not merely cosmetic blemishes. They are the visible signatures of powerful convective processes churning beneath the surface, offering a window into the Sun's inner workings.
The Importance of Granule Study
Understanding these solar features, especially granules, is paramount for several reasons. They provide crucial insights into:
-
Solar Energy Transport: Granules are direct evidence of convection, the primary mechanism by which energy generated in the Sun's core is transported outwards.
-
Solar Magnetic Fields: The movement and evolution of granules are intimately linked to the Sun's complex magnetic field, which drives solar activity like flares and coronal mass ejections.
-
Space Weather Prediction: By studying granules, we can gain a better understanding of the Sun's variability and its impact on space weather, which can affect our technological infrastructure and even our climate.
Article Purpose and Overview
This article aims to illuminate the nature of solar granules, demystifying their formation and highlighting their significance. We will explore:
- The Definition of Solar Granules: What they are, their appearance, and their typical size and lifespan.
- The Convection Process: How the rising and falling of hot plasma gives rise to the granular structure.
- Granule Interactions: Their interactions within the photosphere and with the Sun's magnetic field.
By understanding these fundamental aspects of solar granules, we can begin to appreciate their vital role in the Sun's overall structure and dynamics, and ultimately, their influence on our own world.
The importance of granule study cannot be overstated. They are direct probes into the Sun's convection processes and are thus deeply connected to its magnetic field generation. Let's turn our focus now to defining exactly what these granules are and characterizing their most important features.
Defining Solar Granules: Grains on the Photosphere
Solar granules are essentially the visible signatures of convection occurring just beneath the Sun's photosphere.
Think of them as the "grains" that make up the textured surface of our star, much like grains of sand on a beach.
The Granular Pattern
When viewed through a telescope equipped with proper filters, the photosphere doesn't appear smooth.
Instead, it exhibits a mottled, granular pattern.
This pattern is formed by the tops of convection cells, where hot plasma rises from the Sun's interior, radiates its energy, and then cools and sinks back down.
Anatomy of a Granule
Each granule has a characteristic appearance: a bright center surrounded by darker lanes.
The bright center represents the upwelling of hotter plasma.
As this plasma reaches the surface, it cools and spreads out, eventually sinking back down along the darker lanes that outline the granule.
These dark lanes are cooler regions where the plasma is descending.
Size and Lifespan
Solar granules are surprisingly small, especially when compared to the overall size of the Sun.
A typical granule is around 1000 kilometers in diameter, roughly the size of the state of Texas.
Despite their constant presence, granules are short-lived phenomena.
The typical lifespan of a granule is only about 5 to 10 minutes.
This dynamic turnover indicates a highly active and turbulent convective process happening constantly beneath the Sun's surface.
The continuous formation and dissipation of granules give the photosphere its ever-changing, dynamic appearance.
The typical lifespan of a granule, as we’ve seen, is relatively short – on the order of minutes. But what gives rise to these ephemeral features, constantly bubbling up and fading away across the Sun's face? The answer lies in the fundamental process of convection, the engine that drives not only granule formation but also much of the Sun’s dynamic behavior.
The Convection Process: How Granules are Born
The birth of a solar granule is a dramatic illustration of convection, a process akin to boiling water but on a vastly grander scale. Within the Sun, energy generated by nuclear fusion in the core must be transported outwards.
Radiation is effective in the Sun's deep interior, but as we move closer to the surface, the plasma becomes denser and more opaque.
This impedes radiative transport, making convection the more efficient mechanism for transferring heat.
The Upwelling of Hot Plasma
Imagine plumes of intensely hot plasma rising from deep within the Sun's interior. These plumes, driven by buoyancy, are hotter and less dense than their surroundings.
As they ascend, they carry vast amounts of energy towards the photosphere.
This upward movement of hot plasma is the initial stage in the formation of a granule.
Think of it as the initial spark that ignites the granular pattern we observe.
Cooling and Descent: The Formation of Dark Lanes
Upon reaching the photosphere, the hot plasma releases its energy into space, primarily in the form of light and heat. This radiation causes the plasma to cool.
As it cools, it becomes denser and begins to sink back down into the Sun's interior.
This descending plasma forms the dark lanes that surround each granule.
These lanes are cooler regions compared to the bright centers, creating the characteristic granular contrast. The darker appearance is a direct result of the temperature difference.
The cycle of rising hot plasma and sinking cooler plasma forms a closed loop, continually churning and reshaping the solar surface.
Convection: The Sun's Heat Transfer Mechanism
Convection isn't merely a process that creates granules; it's a fundamental mechanism for energy transport within the Sun. It efficiently moves energy from the Sun's interior to its surface.
This energy is then radiated into space, sustaining life on Earth and driving countless processes throughout the solar system.
Without convection, the Sun's energy balance would be drastically altered, leading to profound changes in its behavior and its influence on its surrounding environment.
The granules we observe are, therefore, the visible manifestation of a crucial heat transfer process. Each granule is a window into the Sun's internal dynamics, revealing the constant flow of energy that sustains our star.
The constant ballet of rising and falling plasma paints a vivid picture of convection at work. But where exactly does this granular drama unfold on the Sun?
Granules Within the Photosphere: A Landscape of Constant Change
The photosphere, often referred to as the Sun's visible surface, is where solar granules reside. It's important to note that the Sun doesn't have a solid surface like Earth. Instead, the photosphere is a layer of plasma from which the majority of the Sun's light is emitted.
Therefore, when we observe solar granules, we are essentially witnessing the top layer of the Sun's convective zone in action. Granules are not surface features per se, but rather manifestations of the convection process occurring just below the photosphere.
The Photosphere: A Sea of Granules
Imagine looking down at a vast, bubbling sea. This is analogous to the appearance of the photosphere when viewed at high resolution. The entire solar surface is covered with these granules, each one representing the top of a convection cell.
The bright centers of the granules are where hot plasma is rising, and the dark lanes are where cooler plasma is sinking back down.
This constant upwelling and downwelling creates a dynamic and ever-changing granular pattern across the entire photosphere.
Granular Appearance and Its Impact
The granular appearance of the photosphere has a significant impact on the Sun's overall appearance. It contributes to the mottled texture we observe.
Without the presence of granules, the Sun would likely appear much smoother and less dynamic.
The constant movement and turnover of granular structures also play a crucial role in transporting energy from the Sun's interior to its outer layers, which ultimately affects the amount of energy radiated into space.
Furthermore, the granular pattern contributes to the observed variations in solar irradiance. It highlights the dynamic and complex nature of our nearest star.
The granular appearance of the photosphere has a significant impact on the Sun's overall appearance, contributing to the mottled texture we observe. Without the presence of granules, the Sun would likely appear much smoother and less dynamic. But these aren't the only convective features at play. The Sun exhibits convection at multiple scales, and understanding these different scales is crucial to grasping the full picture of solar dynamics.
Granules vs. Supergranules: Scales of Convection
While granules represent a fundamental level of solar convection, they are not the only manifestation of this process. The Sun exhibits convective activity on a much larger scale as well, giving rise to features known as supergranules.
Comparing granules and supergranules reveals a hierarchy of convective processes at work within the Sun.
Size and Lifespan: A Matter of Scale
The most immediately apparent difference between granules and supergranules lies in their size.
Typical granules measure around 1,000 kilometers in diameter.
Supergranules, on the other hand, are vastly larger, often reaching diameters of up to 30,000 kilometers.
This makes supergranules significantly larger than the Earth itself!
Lifespan also differs dramatically.
Granules are ephemeral features, typically lasting only 5 to 10 minutes before dissipating.
Supergranules are much more persistent, with lifetimes that can extend for 24 hours or even longer.
The Dynamics of Supergranules
Supergranules are not simply larger versions of granules. Their formation and dynamics are thought to involve different processes occurring deeper within the Sun's convective zone.
While granules are driven by convection near the surface, supergranules are believed to be influenced by larger-scale convective currents originating at greater depths.
These deeper currents create broader patterns of plasma flow, resulting in the larger size and longer lifespan of supergranules.
The Relationship Between Granules and Supergranules
Granules and supergranules are not independent entities; they are interconnected aspects of the Sun's convective system.
Supergranules can be visualized as vast cellular patterns on the photosphere, within which numerous granules constantly form and dissipate.
The boundaries of supergranules are often marked by concentrations of magnetic field, which are swept along by the converging plasma flows.
These magnetic concentrations can influence the formation and behavior of granules within the supergranule cell.
In essence, granules can be seen as embedded within the larger framework of supergranules.
Understanding Solar Convection
By studying both granules and supergranules, scientists gain a more complete understanding of the Sun's convective dynamics.
The differences in size, lifespan, and formation mechanisms provide valuable insights into the complex processes that drive energy transport within the Sun.
These insights are crucial for understanding solar activity, magnetic field generation, and the Sun's overall influence on the solar system.
The stark difference in scale between granules and supergranules underscores the complexity of solar convection. But convection isn't the only process shaping the photosphere. The Sun's magnetic field, a powerful force generated by the movement of electrically conductive plasma, also plays a vital role, and its interaction with granules is far from a passive one.
Granules and the Solar Magnetic Field: An Intertwined Relationship
The relationship between solar granules and the Sun's magnetic field is a dynamic and intricate dance. These seemingly disparate elements are, in fact, deeply intertwined, each influencing the other in a constant feedback loop. Understanding this interaction is paramount to unraveling the mysteries of solar activity and the Sun's overall behavior.
Granule Movement: A Driver of Magnetic Field Dynamics
The movement of granules, driven by convection, directly influences the structure and behavior of the solar magnetic field. As hot plasma rises and spreads across the photosphere, it carries magnetic field lines along with it.
This motion, known as advection, effectively shuffles and concentrates magnetic flux.
The convergence of granules at their boundaries leads to the accumulation of magnetic field lines in these intergranular lanes.
This process can intensify the magnetic field in these regions, potentially leading to the formation of small-scale magnetic features known as magnetic bright points.
These bright points are often associated with enhanced heating in the upper solar atmosphere.
Magnetic Fields: Shaping Granule Behavior
The influence isn't a one-way street. The magnetic field, in turn, can significantly impact the behavior of granules. Strong magnetic fields can suppress convection, inhibiting the formation or altering the shape of granules.
In regions of intense magnetic activity, such as sunspots, the magnetic field is so strong that it completely inhibits convection, preventing the formation of granules altogether.
Even in regions with weaker magnetic fields, the presence of magnetic flux can influence the size, shape, and lifespan of granules.
For instance, granules in active regions tend to be smaller and shorter-lived than those in quieter areas of the Sun.
Unveiling Solar Dynamics: A Crucial Interaction
The interplay between granules and the magnetic field is a key element in understanding the Sun's complex dynamics. By studying how granules interact with magnetic fields, we can gain insights into the processes that drive solar flares, coronal mass ejections, and other forms of solar activity.
These events have a direct impact on space weather, which can affect satellites, communication systems, and even power grids on Earth.
Furthermore, analyzing the magnetic properties of granules provides clues about the internal structure and dynamics of the Sun's convective zone.
The distribution and movement of magnetic fields within granules can reveal information about the strength and organization of the magnetic dynamo, the mechanism responsible for generating the Sun's magnetic field.
Ultimately, unraveling the intricate relationship between granules and the solar magnetic field is essential for building a comprehensive picture of the Sun and its influence on our solar system.
The convergence of granular flows concentrates magnetic fields, and these concentrated fields, in turn, can influence the very dynamics of the granules themselves. But the story doesn't end with the intricate push and pull within the photosphere. These small-scale phenomena have far-reaching consequences, impacting the Sun's overall activity and even influencing the environment of our planet.
The Broader Significance: Implications for Solar Activity and Space Weather
The study of solar granules, seemingly a niche area of solar physics, unlocks crucial insights into the Sun's complex behavior and its influence on the solar system, especially Earth. Understanding these photospheric grains provides a foundation for comprehending larger solar phenomena. These include solar flares, coronal mass ejections (CMEs), and the overall solar cycle. Ultimately, this knowledge is critical for predicting and mitigating the effects of space weather.
Granules as Windows into Solar Variability
Solar granules are not static entities. Their number, size, and distribution vary over time, reflecting changes in the Sun's internal dynamics. These variations, though subtle, offer valuable clues about the underlying processes driving solar variability.
By meticulously tracking and analyzing these changes, scientists can gain a better understanding of the solar dynamo. This dynamo is responsible for generating the Sun's magnetic field. This understanding is a cornerstone of predicting the solar cycle and the associated changes in solar activity.
The Link to Space Weather
The Sun's activity has a direct impact on space weather, which refers to the conditions in space influenced by solar emissions. Solar flares and CMEs, powerful eruptions of energy and matter from the Sun, can disrupt the Earth's magnetosphere. They can also interfere with radio communications, damage satellites, and even cause power grid failures.
While granules themselves are not the direct cause of these major events, their dynamics are intricately linked to the magnetic field configurations that give rise to them. The granular motions help shape and concentrate magnetic flux, creating the conditions ripe for flares and CMEs.
Forecasting Solar Events
A deeper understanding of the interplay between granules and the magnetic field is crucial for improving space weather forecasting. By monitoring the evolution of granular patterns and their associated magnetic fields, scientists can potentially identify regions of high magnetic stress.
These are the regions where flares and CMEs are more likely to occur. Early warnings can allow satellite operators and power grid managers to take protective measures. This includes shutting down sensitive equipment or rerouting power flow to minimize the impact of these events.
A Continuous Pursuit
The study of solar granules is an ongoing endeavor, with new observations and advanced computer simulations constantly refining our understanding. As we continue to probe the secrets of these photospheric grains, we move closer to unraveling the mysteries of the Sun.
The goal is to predict its behavior and mitigate the potential risks posed by solar activity. The knowledge has become increasingly important in our technologically dependent world.
Video: Solar Granules: What Are They and Why Do They Matter?
Solar Granules: Frequently Asked Questions
This FAQ aims to answer common questions about solar granules and their importance in understanding the Sun.
What exactly are solar granules?
Solar granules are convection cells found on the Sun's photosphere – its visible surface. These granules are essentially the tops of rising plumes of hot plasma. The bright center is where hot plasma rises, cools, and then sinks back down along the darker edges.
How big are these solar granules?
A typical solar granule is about 1,000 kilometers (620 miles) in diameter. To put that in perspective, that's roughly the size of the state of Texas! They're much smaller than sunspots, but cover the entire photosphere.
Why are solar granules important to study?
Studying solar granules helps scientists understand the Sun's internal dynamics and energy transport. By observing the behavior and movement of these convection cells, researchers can learn more about the processes that drive solar activity and how the Sun impacts Earth. Changes in solar granules can indicate changes in the Sun's overall behavior.
How long do solar granules typically last?
Solar granules are transient features, meaning they don't last very long. A typical granule lasts for only about 5 to 20 minutes before dissipating and being replaced by new granules. This constant churning and change is a key characteristic of the Sun's surface.