Fungi: Unicellular vs Multicellular - Key Differences
Fungi, a diverse kingdom within Eukaryota, exhibit variations in cellular structure, influencing their biological functions and ecological roles. Saccharomyces cerevisiae, a type of yeast, represents a prominent example of unicellular fungi, which are widely used in industrial fermentation processes. Contrastingly, Agaricus bisporus, commonly known as the button mushroom, exemplifies multicellular fungi, forming complex structures with specialized tissues. Research at institutions like the Mycological Society of America has significantly contributed to understanding whether fungi are unicellular or multicellular, uncovering the genetic and environmental factors influencing their morphology. Methods such as microscopy enable scientists to observe and differentiate between these two forms, revealing the fundamental differences that dictate their life cycles and interactions within ecosystems.
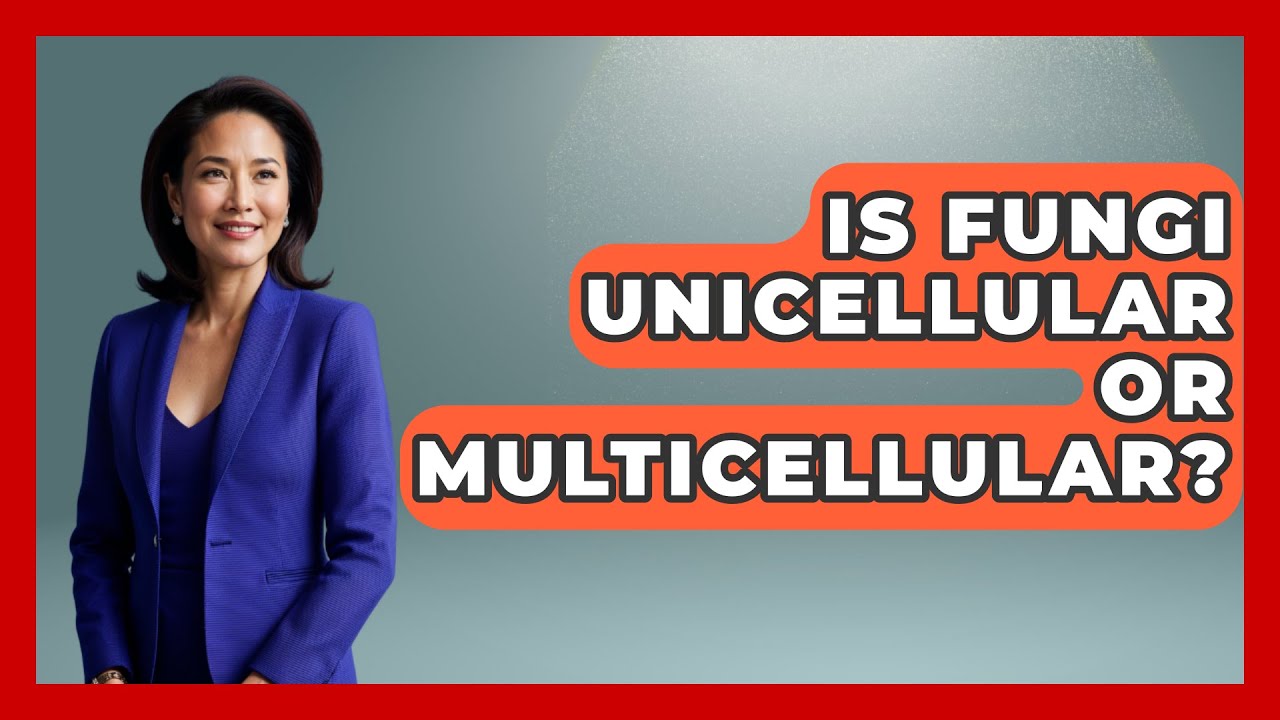
Image taken from the YouTube channel Biology for Everyone , from the video titled Is Fungi Unicellular Or Multicellular? - Biology For Everyone .
Unveiling the Kingdom of Fungi: A World Apart
Fungi, often overlooked, constitute a distinct and vital kingdom of life. They are neither plant nor animal, occupying their own unique branch on the evolutionary tree.
But what exactly are fungi, and what sets them apart?
Defining Fungi and Their Evolutionary Position
Fungi are eukaryotic organisms, meaning their cells possess a membrane-bound nucleus and other complex organelles.
Phylogenetically, they are more closely related to animals than plants, a surprising revelation underscored by molecular evidence. This closer kinship reflects similarities in cellular structure and biochemical processes, solidifying their placement within the Opisthokonta clade, which also includes animals.
Distinguishing Features: Fungi vs. Plants and Animals
Several key characteristics differentiate fungi from both plants and animals.
-
Cell Walls: Unlike plants with cellulose-based cell walls, fungi possess cell walls composed primarily of chitin, a tough, resilient polysaccharide also found in the exoskeletons of insects. This difference in cell wall composition is a fundamental distinction.
-
Nutrition: Fungi are heterotrophic, meaning they obtain nutrients from external sources. Unlike animals that ingest food, fungi secrete enzymes that break down organic matter externally, then absorb the resulting nutrients. This absorptive mode of nutrition is characteristic of fungi. Plants, in contrast, are autotrophic, synthesizing their own food through photosynthesis.
-
Structure: While some fungi are unicellular (e.g., yeasts), most are multicellular, composed of thread-like structures called hyphae. These hyphae intertwine to form a network known as a mycelium, which can permeate soil, wood, or other substrates. This unique structural organization distinguishes fungi from the more defined tissues and organ systems of plants and animals.
The Ecological Significance of Fungi
Fungi play diverse and crucial roles in virtually every ecosystem on Earth.
-
Decomposers: Perhaps their most well-known role is as decomposers. They break down dead organic matter, recycling essential nutrients back into the environment. Without fungi, decomposition rates would drastically slow, leading to the accumulation of organic debris and the disruption of nutrient cycles.
-
Symbionts: Many fungi form symbiotic relationships with other organisms. Mycorrhizae, for example, are associations between fungi and plant roots. The fungi enhance nutrient and water uptake by the plant, while the plant provides the fungi with carbohydrates. These symbiotic relationships are essential for the health and productivity of many plant communities.
-
Parasites: Some fungi are parasitic, obtaining nutrients from living organisms and causing harm. These parasitic fungi can infect plants, animals, and even other fungi, leading to diseases that can have significant ecological and economic consequences.
Fungi: Benefactors of Humanity
Beyond their ecological importance, fungi have profound implications for human society.
-
Medicine: Fungi are a source of many important medicines, most notably antibiotics. Penicillin, discovered from the fungus Penicillium, revolutionized the treatment of bacterial infections. Fungi continue to be investigated for novel therapeutic compounds.
-
Agriculture: Fungi, particularly mycorrhizal fungi, play a crucial role in agriculture. By enhancing nutrient uptake, they improve crop yields and reduce the need for synthetic fertilizers.
-
Industry: Fungi are used in a wide range of industrial processes. Yeasts, for instance, are essential for the production of bread, beer, and wine. Fungi are also used in the production of enzymes, organic acids, and other valuable products.
The kingdom of Fungi is a testament to the extraordinary diversity and adaptability of life. Understanding their unique characteristics and multifaceted roles is essential for appreciating their importance in the natural world and their potential to benefit humanity.
Unicellular vs. Multicellular Fungi: A Tale of Two Forms
Having established the unique position of fungi within the biological world, it is now pertinent to examine the diverse forms they assume. From the microscopic simplicity of single-celled yeasts to the complex, interwoven structures of molds, fungi exhibit a remarkable range of morphologies.
This section will delve into the fundamental differences between unicellular and multicellular fungi, providing a comparative analysis of their characteristics and attributes. Specific examples will be used to illustrate these differences, highlighting the adaptive strategies that underpin their ecological success.
Unicellular Fungi: The Microscopic Mavericks
Unicellular fungi, as the name suggests, exist as single, independent cells. This contrasts sharply with the multicellular organization found in plants and animals. The most familiar representatives of this group are the yeasts.
Defining Characteristics of Yeasts
Yeasts are characterized by their relatively simple morphology, typically appearing as oval or spherical cells. Their reproduction is primarily asexual, with budding being the most common method. This involves the formation of a small outgrowth or "bud" on the parent cell, which eventually detaches to become a new, independent yeast cell.
Metabolically, yeasts are capable of both aerobic and anaerobic respiration. The latter process, fermentation, is of immense importance in various industrial applications.
Saccharomyces cerevisiae: A Keystone Species
Saccharomyces cerevisiae, commonly known as baker's yeast, is arguably the most well-known and economically significant unicellular fungus. It plays a crucial role in the production of bread, beer, and wine.
The ability of S. cerevisiae to ferment sugars into ethanol and carbon dioxide is the basis for these processes. The carbon dioxide causes bread to rise, while the ethanol contributes to the alcoholic content of beer and wine.
Beyond its culinary applications, S. cerevisiae has also emerged as a powerful model organism in scientific research. Its relatively simple genome and ease of cultivation have made it invaluable for studying fundamental biological processes, including cell division, gene regulation, and protein synthesis.
Multicellular Fungi: The Architects of Mycelial Networks
Multicellular fungi, in contrast to their unicellular counterparts, are characterized by a complex, filamentous structure. Molds are a familiar example of this growth habit.
Defining Characteristics of Molds
Molds are composed of thread-like structures called hyphae. These hyphae intertwine to form a network known as the mycelium, which is the vegetative body of the fungus. The mycelium grows and spreads through the substrate, absorbing nutrients as it goes.
Reproduction in molds typically involves the formation of spores, which are dispersed into the environment to initiate new colonies. Spore formation can occur both asexually and sexually, depending on the species and environmental conditions.
Hyphae and Mycelium: The Building Blocks of Multicellularity
The structure of hyphae is crucial to understanding the growth and function of molds. Hyphae can be either septate or aseptate. Septate hyphae are divided into individual cells by cross-walls called septa, while aseptate hyphae lack these divisions and are essentially multinucleate cells.
The mycelium, formed by the collective growth of hyphae, allows the fungus to efficiently explore and colonize its environment. The extensive surface area of the mycelium facilitates nutrient absorption and decomposition.
Environmental Impact of Molds
Molds play a significant role in the environment as decomposers. They break down complex organic matter, such as dead plants and animals, releasing nutrients back into the ecosystem.
However, molds can also have negative impacts. Some species are pathogenic, causing diseases in plants, animals, and humans. Others can spoil food and contaminate indoor environments, leading to health problems.
Delving Deeper: Fungal Structures and Morphology
Having established the unique position of fungi within the biological world, it is now pertinent to examine the diverse forms they assume. From the microscopic simplicity of single-celled yeasts to the complex, interwoven structures of molds, fungi exhibit a remarkable range of morphologies.
Understanding the fundamental building blocks and organizational principles of these organisms is crucial to appreciating their ecological roles and diverse applications. We will now explore the key structural components of fungi, ranging from the cell wall to the mycelium, explaining their composition and functional significance.
The Fungal Cell Wall: A Fortress of Chitin
The fungal cell wall is a defining characteristic of these organisms, providing structural support, protection, and shape. Unlike plant cell walls, which are composed primarily of cellulose, the fungal cell wall is predominantly composed of chitin, a complex polysaccharide also found in the exoskeletons of insects and crustaceans.
Chitin's inherent strength and flexibility make it an ideal material for constructing a robust yet adaptable cell wall. The structural integrity of the cell wall is paramount, enabling fungi to withstand osmotic pressure and mechanical stress, particularly in diverse and often harsh environments.
Chitin: The Primary Structural Component
Chitin is a polymer of N-acetylglucosamine, linked together by β-1,4-glycosidic bonds. This arrangement forms long, linear chains that are highly crystalline and provide exceptional tensile strength.
These chains are further organized into microfibrils, which are embedded in a matrix of other polysaccharides and proteins, thus forming the cell wall. The crystalline nature of chitin lends the cell wall its rigidity, while the surrounding matrix provides flexibility and elasticity.
Other Cell Wall Components
While chitin is the primary component, fungal cell walls also contain other polysaccharides such as glucans (β-1,3-glucans and β-1,6-glucans) and proteins. Glucans contribute to the cell wall's structural integrity and provide a matrix for the chitin microfibrils.
Proteins embedded within the cell wall play various roles, including enzymatic activity, cell signaling, and adhesion. The specific composition of the cell wall can vary depending on the fungal species and environmental conditions, reflecting the adaptability of these organisms.
The Mycelium: An Interconnected Network
The mycelium is the vegetative part of a fungus, consisting of a network of thread-like filaments called hyphae. This intricate network is often hidden beneath the surface of the substrate, whether it be soil, decaying wood, or even living tissue.
The mycelium is responsible for nutrient absorption and transport, playing a critical role in the fungal life cycle. Its expansive reach allows fungi to efficiently colonize and exploit diverse environments.
Hyphae: The Building Blocks of the Mycelium
Hyphae are the fundamental building blocks of the mycelium. They are long, branching filaments that extend through the substrate, secreting enzymes to break down organic matter and absorbing the resulting nutrients.
Hyphae exhibit two primary forms: septate and aseptate (coenocytic). This distinction influences nutrient flow and compartmentalization within the mycelium.
Septate vs. Aseptate Hyphae
Septate hyphae are characterized by the presence of cross-walls, called septa, that divide the hyphae into individual cells. These septa typically contain pores that allow for the movement of cytoplasm, organelles, and nutrients between cells.
In contrast, aseptate hyphae (also known as coenocytic hyphae) lack these cross-walls and consist of a single, elongated cell containing numerous nuclei. This multinucleate condition allows for rapid nutrient distribution throughout the mycelium.
The presence or absence of septa reflects different strategies for nutrient management and cellular organization within fungal species. Septate hyphae offer greater compartmentalization and control, while aseptate hyphae facilitate rapid, coordinated growth.
Spores: Agents of Reproduction and Dispersal
Spores are the reproductive units of fungi, analogous to seeds in plants. These microscopic propagules are responsible for dispersal, colonization of new environments, and propagation of the fungal species.
Fungi produce vast quantities of spores, ensuring their survival and distribution across diverse habitats. Spore formation, dispersal mechanisms, and germination processes are critical aspects of the fungal life cycle.
Spore Formation and Dispersal
Spores can be formed through both asexual and sexual reproduction. Asexual spores, such as conidia, are produced through mitosis and are genetically identical to the parent organism. Sexual spores, such as ascospores and basidiospores, are produced through meiosis and genetic recombination, resulting in increased genetic diversity.
Fungal spores are dispersed through a variety of mechanisms, including wind, water, and animal vectors. Some fungi actively discharge their spores, while others rely on passive dispersal.
The small size and lightweight nature of spores facilitate their long-distance travel, enabling fungi to colonize new habitats far from their origin.
Spore Germination
Spore germination is the process by which a spore develops into a new fungal mycelium. Germination is triggered by favorable environmental conditions, such as adequate moisture, temperature, and nutrient availability.
Upon germination, the spore absorbs water, and its metabolic activity increases. The spore then develops one or more germ tubes, which elongate and branch to form the hyphae that constitute the mycelium.
Successful spore germination is essential for the establishment of new fungal colonies and the continuation of the fungal life cycle. The ability to germinate and thrive in diverse environments is a testament to the adaptability and resilience of fungi.
Fungal Physiology and Nutrition: Masters of Heterotrophic Acquisition
Having established a foundational understanding of fungal structures, our focus now shifts to the vital processes that sustain fungal life—physiology and nutrition. Fungi, unlike plants, cannot harness energy directly from sunlight. They are heterotrophic organisms, meaning they must obtain their nourishment from pre-existing organic matter.
This dependence has led to a remarkable array of nutritional strategies, shaping their ecological roles and interactions within diverse ecosystems. We will now delve into these strategies, highlighting their central role in decomposition and nutrient cycling.
Heterotrophic Lifestyles: Saprophytes, Parasites, and Symbionts
Fungi have evolved diverse methods to acquire nutrients. These are broadly classified into three primary lifestyles: saprophytic, parasitic, and symbiotic. Each of these strategies reflects a unique interaction with the surrounding environment and other organisms.
Saprophytes: Decomposers of the Dead
Saprophytic fungi are the quintessential decomposers of the natural world. They obtain their nutrients from non-living organic matter, playing a critical role in breaking down complex molecules into simpler compounds. Think of fallen logs, decaying leaves, and even animal carcasses; these are the feasts of saprophytes.
Enzymes secreted by these fungi digest the complex compounds, which are then absorbed directly through the fungal cell walls. Mushrooms, bracket fungi, and many molds are examples of saprophytes, diligently returning nutrients to the soil and air.
Parasites: Extracting Resources from Living Hosts
In stark contrast to saprophytes, parasitic fungi derive their sustenance from living organisms, known as hosts. This relationship is often detrimental to the host, as the fungus extracts nutrients, causing disease or even death.
Parasitic fungi have evolved specialized structures to penetrate host tissues and absorb nutrients. Athlete's foot fungus (Trichophyton) and corn smut (Ustilago maydis) represent the diverse range of parasitic fungi impacting both human health and agriculture.
Mutualistic Symbionts: Cooperative Partnerships
Not all fungal interactions are destructive. Mutualistic symbiotic fungi engage in mutually beneficial relationships with other organisms.
A prime example is mycorrhizae, the symbiotic association between fungi and plant roots. The fungus aids the plant in absorbing water and nutrients from the soil, while the plant provides the fungus with sugars produced through photosynthesis.
Another illustrative case is lichens, which consist of a symbiotic relationship between a fungus and an alga or cyanobacterium. The fungus provides structure and protection, while the alga or cyanobacterium photosynthesizes, providing the fungus with nutrients.
Fungi as Decomposers: Recyclers of Nature
The ability of fungi to decompose organic matter makes them indispensable recyclers in ecosystems. Their enzymatic arsenal allows them to break down complex substances that other organisms cannot digest, like cellulose and lignin in plant cell walls.
The decomposition process releases essential nutrients, such as nitrogen, phosphorus, and carbon, back into the environment, making them available for other organisms.
The Decomposition Process: A Step-by-Step Breakdown
The fungal decomposition process is a complex, multi-step affair, beginning with the secretion of extracellular enzymes.
These enzymes break down complex organic polymers into smaller, soluble molecules that the fungus can readily absorb.
- Enzyme Secretion: Fungi release a diverse array of enzymes tailored to break down specific organic compounds.
- Breakdown of Polymers: Enzymes cleave complex polymers like cellulose, lignin, and proteins into smaller monomers (e.g., sugars, amino acids).
- Absorption: The fungus absorbs the soluble monomers through its cell walls.
- Nutrient Release: Through respiration, fungi release carbon dioxide into the atmosphere. Simultaneously, inorganic nutrients are released into the soil, nourishing plants and other organisms.
Nutrient Cycling: The Cornerstone of Ecosystem Health
The role of fungi in nutrient cycling cannot be overstated. By decomposing organic matter, they release essential nutrients that would otherwise remain locked within dead organisms. This process sustains plant growth, supports food webs, and maintains the overall health and productivity of ecosystems. Without fungi, the world would be buried under an ever-accumulating pile of dead organic matter, eventually depleting available nutrients and disrupting ecological balance.
The Art of Reproduction: Asexual and Sexual Strategies in Fungi
Fungal Physiology and Nutrition: Masters of Heterotrophic Acquisition
Having established a foundational understanding of fungal structures, our focus now shifts to the vital processes that sustain fungal life—reproduction. Fungi, unlike plants and animals, possess a diverse range of reproductive strategies, employing both asexual and sexual mechanisms to ensure their survival and propagation. A detailed exploration of these methods reveals the adaptability and resilience of these organisms in various environments.
Asexual Reproduction: Cloning and Rapid Proliferation
Asexual reproduction in fungi is characterized by the production of genetically identical offspring, effectively cloning the parent organism. This strategy allows for rapid proliferation, especially in stable and favorable conditions where genetic diversity is not immediately necessary.
Spore Formation and Dispersal
The most common method of asexual reproduction involves the formation of spores, specialized reproductive cells capable of developing into new individuals. These spores are often produced in vast numbers and are adapted for dispersal via wind, water, or animal vectors. The efficiency of spore dispersal is crucial for the colonization of new habitats.
The conidia are the spores produced through asexual reproduction and the process of conidiogenesis. Conidia are non-motile spores that are adaptable to differing environmental conditions.
Budding: A Yeast-Specific Strategy
Budding is a distinctive form of asexual reproduction predominantly observed in yeasts, a group of unicellular fungi. During budding, a small outgrowth, or bud, develops on the parent cell. This bud gradually enlarges, eventually separating to form a new, independent yeast cell. This method allows for rapid population growth in nutrient-rich environments.
Fragmentation: A Less Common Alternative
Fragmentation represents another mode of asexual reproduction, albeit less prevalent. It consists of the separation of the mycelium or hyphae into fragments, with each fragment developing into a new, independent fungal colony. This method is particularly effective in environments where physical disturbance or breakage is common.
Sexual Reproduction: Generating Diversity and Adaptability
In contrast to asexual reproduction, sexual reproduction in fungi involves the fusion of genetic material from two different individuals, resulting in offspring with novel combinations of traits. This process enhances genetic diversity, increasing the adaptability of fungal populations to changing environmental conditions.
Fusion of Hyphae and Spore Formation
The process of sexual reproduction typically begins with the fusion of hyphae from compatible mating types. This fusion leads to the formation of a zygote, which then undergoes meiosis to produce sexual spores. These spores are genetically distinct from the parent organisms and possess the potential to colonize new environments and thrive under altered selective pressures.
Diversity in Sexual Spores
Fungi exhibit a range of sexual spore types, each characterized by unique morphology and developmental pathways. Ascospores, for example, are formed within a sac-like structure called an ascus, while basidiospores are produced on specialized cells called basidia. Understanding the diversity of sexual spores provides insights into the evolutionary history and ecological adaptations of different fungal groups.
In conclusion, fungi possess an impressive array of reproductive strategies, encompassing both asexual and sexual mechanisms. These strategies allow them to thrive in diverse environments and play crucial roles in ecological processes.
Fungal Dimorphism: Adapting to Different Environments
Having unveiled the intricacies of fungal reproduction, our exploration now turns to a remarkable adaptation exhibited by certain fungal species: dimorphism. This phenomenon highlights the inherent plasticity of fungi, enabling them to thrive in fluctuating and challenging environments. Dimorphism showcases how fungi dynamically adjust their morphology to suit prevailing conditions.
Defining and Understanding Fungal Dimorphism
Fungal dimorphism refers to the ability of some fungi to exist in two distinct morphological forms—either as a unicellular yeast or as a multicellular filamentous mold—depending on environmental cues. This capacity is not merely a cosmetic alteration; it represents a profound shift in cellular structure and function, driven by complex genetic and biochemical mechanisms.
The significance of dimorphism lies in its adaptive value. By switching between these forms, fungi can optimize their survival and propagation under varying conditions. This flexibility can be crucial for virulence in pathogenic fungi, where the yeast form may facilitate dissemination within a host.
Environmental Triggers of Morphological Shift
The transition between yeast and mold forms is often triggered by specific environmental factors. These factors act as signals, prompting the fungus to adopt the morphology best suited for the given situation.
-
Temperature: Temperature is perhaps the most well-known trigger. In many dimorphic fungi, the yeast form is favored at higher temperatures (e.g., body temperature of a host), while the mold form predominates at lower, environmental temperatures.
-
Nutrient Availability: Nutrient levels can also influence morphology. Certain nutrients may favor one form over the other, impacting growth and development.
-
pH: Changes in pH can also induce morphological transitions. Some fungi prefer the yeast form in acidic environments and the mold form in alkaline environments.
-
Carbon Dioxide Concentration: Elevated CO₂ levels, such as those found within the host, may promote yeast formation.
Candida albicans: A Prime Example of Fungal Dimorphism
Candida albicans is a classic example of a dimorphic fungus, and its dimorphism plays a critical role in its pathogenicity. This opportunistic pathogen is a common inhabitant of the human microbiome, but can cause infections when the host's immune system is compromised or when the balance of the microbiome is disrupted.
Pathogenicity and Human Health
Candida albicans can transition between a relatively harmless yeast form and a more invasive filamentous form. This morphological switch is integral to its ability to cause disease. The yeast form is primarily responsible for colonization and dissemination, whereas the filamentous form enhances tissue penetration and biofilm formation.
-
Yeast Form: Facilitates adherence to host cells and evasion of the immune system. It's crucial for the initial colonization of mucosal surfaces.
-
Filamentous Form: Enhances tissue invasion and biofilm formation. Hyphae can penetrate deeper into tissues, causing more severe infections.
The ability to switch between these forms allows Candida albicans to adapt to different niches within the host and to evade immune defenses, thereby promoting its survival and virulence. Understanding the mechanisms that govern dimorphism in Candida albicans is therefore crucial for developing effective strategies to combat candidiasis.
Meet the Fungi: Prominent Examples and Their Impact
Having unveiled the intricacies of fungal dimorphism, our exploration now takes a practical turn, spotlighting specific fungal species that significantly impact human society and the environment. By examining Saccharomyces cerevisiae, Candida albicans, and other notable fungi, we can more fully appreciate the diverse roles and consequences of these organisms.
Saccharomyces cerevisiae: The Unsung Hero of Fermentation
Saccharomyces cerevisiae, commonly known as baker's yeast, is arguably one of the most crucial fungi in human history. Its importance extends far beyond the simple act of leavening bread.
Food Production: A Cornerstone of Culinary Arts
The yeast's primary claim to fame is its pivotal role in food and beverage production. The fermentation process, driven by S. cerevisiae, is fundamental to the creation of:
- Bread: The yeast consumes sugars and releases carbon dioxide, causing the dough to rise.
- Beer: Similarly, fermentation converts sugars into alcohol and carbon dioxide, giving beer its characteristic fizz and alcoholic content.
- Wine: S. cerevisiae is responsible for the alcoholic fermentation of grape juice into wine.
The impact of S. cerevisiae on the culinary arts cannot be overstated. Its ability to transform raw ingredients into palatable and enjoyable products has shaped cuisines worldwide.
Scientific Research: A Model Eukaryotic Organism
Beyond its culinary applications, S. cerevisiae has emerged as a powerful model organism in scientific research. Its relatively simple genome, ease of cultivation, and well-characterized genetics make it an ideal system for studying:
- Cell biology: Many fundamental cellular processes were first elucidated in yeast.
- Genetics: Yeast has been instrumental in understanding gene function and regulation.
- Aging: Due to its relatively short lifespan, yeast is used to investigate the mechanisms of aging.
The insights gained from studying S. cerevisiae have had profound implications for our understanding of human biology and disease. It serves as a cornerstone in genetic and genomic studies.
Candida albicans: A Dual-Edged Sword
In stark contrast to the benign S. cerevisiae, Candida albicans represents the darker side of the fungal kingdom. While it can exist as a harmless commensal organism, it is also a significant human pathogen.
Dimorphism and Pathogenicity: A Strategy for Survival
Candida albicans exhibits dimorphism, the ability to switch between yeast and hyphal forms.
This adaptability plays a crucial role in its pathogenicity, allowing it to:
- Colonize different environments: The yeast form facilitates dissemination, while the hyphal form promotes tissue invasion.
- Evade immune responses: The morphological switch can help C. albicans evade host defenses.
Impact on Human Health: A Common Cause of Infections
As an opportunistic pathogen, C. albicans is a frequent cause of various infections, including:
- Oral thrush: A common infection of the mouth, particularly in infants and immunocompromised individuals.
- Vaginal yeast infections: A prevalent condition affecting women, characterized by itching, burning, and discharge.
- Invasive candidiasis: A severe systemic infection that can occur in immunocompromised patients, often with life-threatening consequences.
The ability of C. albicans to transition between different forms and its inherent resistance to certain antifungal drugs pose significant challenges to the treatment of Candida infections.
Other Prominent Fungal Examples
Beyond S. cerevisiae and C. albicans, many other fungi have significant impacts on human society.
- Penicillium: This genus is renowned for producing penicillin, a revolutionary antibiotic that has saved countless lives.
- Aspergillus: While some Aspergillus species are used in food production (e.g., Aspergillus oryzae in sake production), others are opportunistic pathogens that can cause aspergillosis, a serious lung infection.
- Mushrooms (Basidiomycetes): This group includes both edible and poisonous species, some of which are highly prized culinary delicacies, while others can cause severe illness or death.
The fungal kingdom presents a complex mosaic of beneficial and harmful organisms, each with its own unique characteristics and impact on our world.
Video: Fungi: Unicellular vs Multicellular - Key Differences
FAQs: Unicellular vs Multicellular Fungi
What is the biggest structural difference between unicellular and multicellular fungi?
Unicellular fungi, like yeast, are single-celled organisms. Multicellular fungi, such as mushrooms, are composed of many cells organized into structures called hyphae, forming a mycelium. This is the primary structural difference between these types of fungi. Therefore, when discussing fungi unicellular or multicellular, the cell number is the defining factor.
How does reproduction differ in unicellular and multicellular fungi?
Unicellular fungi primarily reproduce asexually through budding or fission, creating identical copies. Multicellular fungi reproduce both sexually (through spore formation after hyphal fusion) and asexually (through spore fragmentation or budding). Considering fungi unicellular or multicellular impacts the complexity of their reproductive strategies.
Which environments are more favorable for unicellular vs. multicellular fungi?
Unicellular fungi often thrive in moist, nutrient-rich environments where rapid reproduction is advantageous. Multicellular fungi can colonize more diverse and challenging environments due to their complex structures and ability to transport nutrients over longer distances. Thinking about fungi unicellular or multicellular is helpful in understanding their environmental niches.
Give a common example of each type of fungi and their uses.
A common example of unicellular fungi is Saccharomyces cerevisiae (baker's yeast), used for baking and brewing. A common example of multicellular fungi is Agaricus bisporus (button mushroom), a popular edible mushroom. This illustrates how fungi unicellular or multicellular have distinct applications in food and industry.
So, the next time you're enjoying some bread or find yourself marveling at a vibrant mushroom in the forest, remember the fascinating world of fungi, unicellular or multicellular. From the tiny yeasts to the sprawling networks underground, their differences highlight the incredible diversity and adaptability of these often-overlooked organisms.