Fluorine Bohr Model: The Simple Guide You NEED To See!
The Bohr model, a foundational concept in atomic physics, provides a simplified representation of atomic structure. Fluorine, an element studied extensively using this model, showcases the Bohr model's applicability. Specifically, the fluorine bohr model helps illustrate the quantization of electron energy levels, as described by Niels Bohr. Understanding the arrangement of electrons in a fluorine bohr model is critical for analyzing its chemical properties.
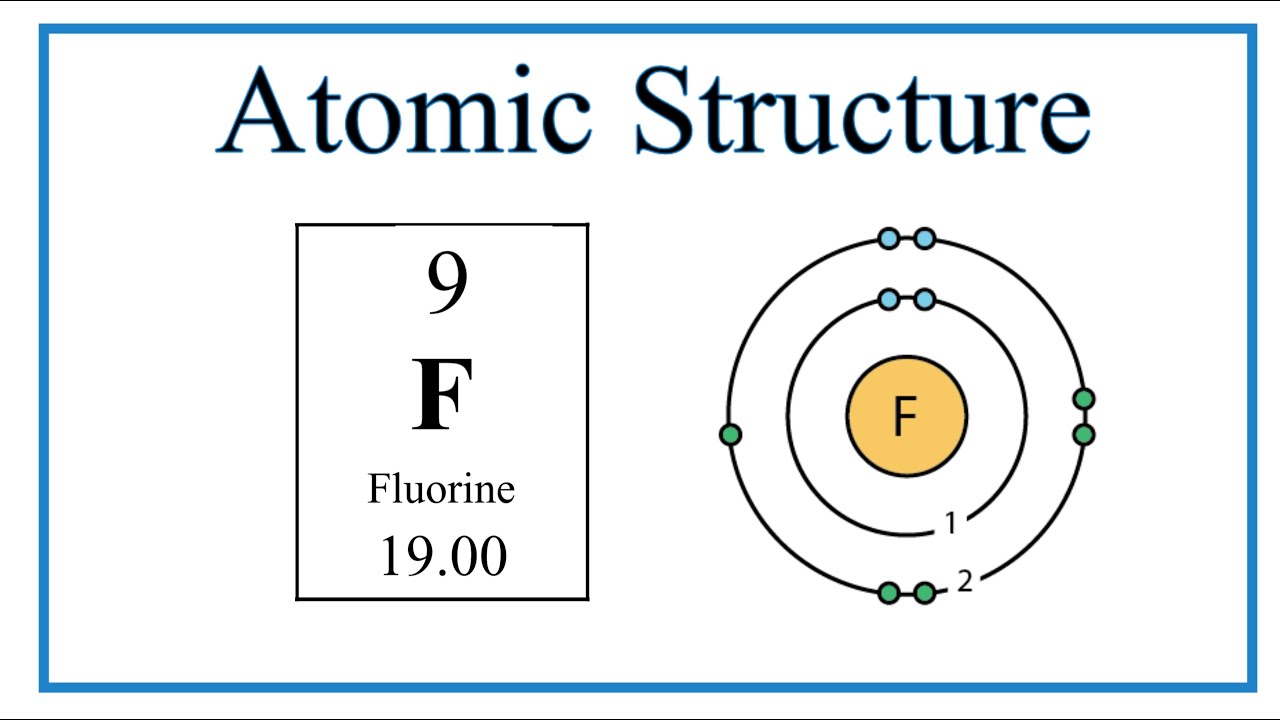
Image taken from the YouTube channel Wayne Breslyn (Dr. B.) , from the video titled Atomic Structure (Bohr Model) for Fluorine (F .
Imagine a world without toothpaste, non-stick cookware, or life-saving medications like certain antidepressants. It's a vastly different place, and it's a world without fluorine. This seemingly unassuming element plays a pivotal role in countless aspects of modern life, from promoting dental health to enabling advanced chemical processes.
But what exactly is fluorine, and how does it behave at the atomic level? To understand this, we turn to a foundational concept in chemistry: the Bohr model.
This article aims to provide a simplified, accessible explanation of the Fluorine Bohr model, a visual representation of the fluorine atom's structure based on Niels Bohr's groundbreaking atomic theory. By exploring this model, we can gain valuable insights into fluorine's unique properties and its importance in the world around us.
The Significance of the Fluorine Bohr Model
The Bohr model, while superseded by more complex quantum mechanical models, remains an incredibly useful tool for visualizing atomic structure, especially for elements like fluorine. It allows us to understand how electrons are arranged around the nucleus and how these arrangements influence an element's behavior.
The Bohr Model: A Foundational Atomic Model
At its core, the Bohr model, proposed by Niels Bohr in 1913, presents a simplified picture of the atom. In this model, electrons orbit the nucleus in specific, quantized energy levels or shells.
These shells are arranged in increasing order of energy, with the innermost shell (closest to the nucleus) having the lowest energy. The Bohr model postulates that electrons can only occupy these specific energy levels and can jump between them by absorbing or emitting energy in the form of photons.
While the Bohr model has limitations, it serves as an invaluable stepping stone for understanding more complex atomic theories and provides a clear, visual representation of atomic structure.
Imagine a world without toothpaste, non-stick cookware, or life-saving medications like certain antidepressants. It's a vastly different place, and it's a world without fluorine. This seemingly unassuming element plays a pivotal role in countless aspects of modern life, from promoting dental health to enabling advanced chemical processes.
But what exactly is fluorine, and how does it behave at the atomic level? To understand this, we turn to a foundational concept in chemistry: the Bohr model.
Now that we've introduced the importance of fluorine and the tool we'll use to understand it – the Bohr model – it's time to delve into the specifics. Before visualizing the atom, we need to establish fluorine's fundamental identity. What defines it as fluorine, and what properties are key to its unique behavior?
Fluorine's Atomic Identity: Basics and Properties
This section lays the groundwork for understanding fluorine's Bohr model by exploring its atomic characteristics. We'll examine its elemental nature, its place within the periodic table, and the significance of its electron configuration.
Fluorine: An Overview
Defining Fluorine
Fluorine, represented by the symbol F, is a chemical element. It exists as a pale yellow diatomic gas under standard conditions.
It is the lightest halogen and is renowned for being the most electronegative and reactive of all elements. This extreme reactivity is a defining characteristic.
Position in the Periodic Table
Fluorine resides in Group 17 (also known as Group VIIA) of the periodic table. These elements are collectively called the halogens.
Halogens are characterized by having seven valence electrons. This electron configuration makes them eager to gain an electron to achieve a stable octet.
Fluorine's position as the first element in the halogen group contributes to its exceptional reactivity compared to the heavier halogens like chlorine and bromine.
Key Properties
Fluorine's high reactivity is due to its strong desire to gain an electron. It readily forms compounds with almost all other elements.
Its exceptional electronegativity means it attracts electrons very strongly in chemical bonds. This leads to the formation of highly polar bonds.
Fluorine is also a powerful oxidizing agent. It readily accepts electrons from other substances, causing them to become oxidized.
The Atomic Number: A Key Identifier
Defining Atomic Number
The atomic number is a fundamental property of an element. It defines the element's identity.
The atomic number represents the number of protons found in the nucleus of an atom of that element. It is usually denoted by the symbol Z.
The number of protons dictates the element's chemical behavior. Each element has a unique atomic number, differentiating it from all others.
Fluorine's Atomic Number
Fluorine has an atomic number of 9. This means that every fluorine atom contains 9 protons in its nucleus.
This seemingly simple number has profound implications. It dictates the number of electrons present in a neutral fluorine atom.
The 9 protons are balanced by 9 electrons, arranged in specific energy levels around the nucleus. This arrangement shapes its chemical properties.
Electron Configuration Unveiled
Electron configuration describes how electrons are arranged within the energy levels and sublevels of an atom.
This arrangement follows specific rules and principles. The most important rule is that electrons fill the lowest energy levels first.
Understanding electron configuration is essential for predicting an element's bonding behavior and chemical reactivity.
Fluorine's Electron Configuration
Fluorine's electron configuration is 1s² 2s² 2p⁵. This notation reveals the distribution of its 9 electrons.
The '1s²' indicates that the first energy level (n=1) has two electrons in the s sublevel.
The '2s²' indicates that the second energy level (n=2) has two electrons in the s sublevel.
The '2p⁵' indicates that the second energy level (n=2) has five electrons in the p sublevel.
Significance of Valence Electrons
Valence electrons are the electrons in the outermost shell of an atom. They are crucial for chemical bonding.
Fluorine has seven valence electrons (2s² 2p⁵). It requires only one more electron to complete its octet (eight electrons) in the outer shell.
This electron configuration explains fluorine's extreme reactivity. Its strong tendency to gain one electron makes it a highly reactive nonmetal.
Fluorine's eagerness to react, as we've seen, stems directly from its electron configuration. But to truly grasp why it behaves this way, and to visualize the atom itself, we need a model. The Bohr model, while simplified, offers an excellent starting point for understanding how electrons are arranged around the nucleus and how this arrangement dictates fluorine's properties.
The Bohr Model and Fluorine: A Visual Representation
The Bohr model provides a simplified, yet insightful, way to visualize atomic structure. By applying this model to fluorine, we can gain a clearer understanding of its electronic configuration and reactivity. It's important to remember that while it's not a perfect representation, it's a helpful stepping stone.
Introducing the Bohr Model
The Bohr model, a cornerstone of early atomic theory, owes its existence to the brilliant Danish physicist Niels Bohr. His work revolutionized our understanding of the atom and its structure.
Niels Bohr: A Pioneer of Atomic Theory
Niels Bohr, a name synonymous with atomic structure, proposed his model in 1913. This model marked a significant departure from previous theories. Bohr's atomic model earned him the Nobel Prize in Physics in 1922, solidifying its place in scientific history.
Core Principles of the Bohr Model
The Bohr model rests on two fundamental principles: quantized energy levels and defined electron orbits.
Electrons are restricted to orbiting the nucleus only at specific distances with fixed energy values. These specific orbits are often referred to as energy levels or shells.
Electrons can jump from one energy level to another, emitting or absorbing energy in the process, but they cannot exist between these defined levels. This concept of quantized energy levels was revolutionary for its time.
Visualizing Fluorine's Bohr Model
With the basic principles of the Bohr model established, we can now apply it to visualize the structure of a fluorine atom.
Depiction of Electron Shells
In the Bohr model of fluorine, electrons reside in distinct shells around the nucleus. The first shell, closest to the nucleus, is known as the K shell, followed by the L shell, and so on. For Fluorine, we only need to consider the K and L shells.
Distribution of Electrons in Fluorine
Fluorine, with its atomic number of 9, possesses nine electrons. According to the Bohr model, the K shell can hold a maximum of two electrons. The remaining seven electrons then occupy the L shell. This 2,7 configuration is crucial to understanding fluorine's behavior.
Diagrams and Illustrations for Enhanced Understanding
Visual aids significantly enhance comprehension. A simple diagram of fluorine's Bohr model would show a central nucleus with the K shell containing two electrons and the L shell containing seven. These diagrams make the abstract concept of electron distribution more concrete and accessible.
Energy Levels within the Model
The concept of quantized energy levels is fundamental to the Bohr model. Each electron shell corresponds to a specific energy level.
Quantized Energy Levels Explained
Electrons cannot exist at just any energy level; they are limited to specific, quantized levels. The closer the electron is to the nucleus, the lower its energy level. When an electron gains energy, it jumps to a higher energy level.
Ground State vs. Excited State
An atom is in its ground state when all of its electrons occupy the lowest possible energy levels. If an electron absorbs energy, it can jump to a higher energy level, resulting in an excited state. This excited state is unstable, and the electron will eventually return to its ground state, releasing the absorbed energy in the form of light or heat. The movement between ground and excited states, and their associated quantized energy levels, drive many chemical processes.
Fluorine's eagerness to react, as we've seen, stems directly from its electron configuration. But to truly grasp why it behaves this way, and to visualize the atom itself, we need a model. The Bohr model, while simplified, offers an excellent starting point for understanding how electrons are arranged around the nucleus and how this arrangement dictates fluorine's properties.
Beyond Bohr: Modern Atomic Theory and Its Nuances
While the Bohr model provides a valuable foundation for understanding atomic structure, it's crucial to recognize its limitations. Science is a constantly evolving field, and our understanding of the atom has advanced significantly since Bohr's groundbreaking work. Stepping beyond the Bohr model allows us to appreciate the complexities of modern atomic theory and the nuances of electron behavior.
Limitations of the Bohr Model
The Bohr model, with its neat, circular orbits, paints an appealingly simple picture. However, this simplicity comes at a cost.
Inability to Explain Complex Atoms
The Bohr model works reasonably well for hydrogen, with its single electron, and provides a decent approximation for elements like fluorine.
However, it falls apart when applied to heavier, more complex atoms with multiple electrons. The interactions between these electrons, not accounted for in the Bohr model, significantly alter their behavior.
The Bohr model's failure to accurately describe complex atoms paved the way for the development of quantum mechanics.
Quantum mechanics offers a far more sophisticated and accurate model of the atom, replacing fixed orbits with probability distributions known as orbitals. This shift represents a fundamental change in how we understand electron behavior.
Ground State and Excited State Clarified
The concepts of ground state and excited state are crucial to understanding atomic behavior within both the Bohr model and more advanced theories.
The ground state represents the lowest energy configuration of an atom. In this state, electrons occupy the orbitals closest to the nucleus, resulting in the most stable arrangement.
Conversely, an excited state occurs when an electron absorbs energy (e.g., from heat or light) and jumps to a higher energy level, farther from the nucleus. This state is inherently unstable. The electron will quickly return to its ground state, releasing the absorbed energy as a photon of light. This transition is the basis for many phenomena, including atomic emission spectra.
Modern Atomic Theory: A Quick Overview
Modern atomic theory, rooted in quantum mechanics, presents a far more nuanced view of the atom than the Bohr model.
Quantum Mechanical Model
Instead of electrons orbiting the nucleus in fixed paths, the quantum mechanical model describes electrons existing in orbitals.
These orbitals are three-dimensional regions of space where an electron is most likely to be found. Unlike the defined orbits of the Bohr model, orbitals are described by probability distributions, reflecting the inherent uncertainty in an electron's position and momentum.
Acknowledging Complexity
Modern atomic theory embraces the complexity of the atom. It acknowledges the intricate interactions between electrons, the wave-particle duality of matter, and the probabilistic nature of electron behavior.
While this complexity may seem daunting, it provides a much more accurate and complete picture of the atom, allowing us to understand and predict its behavior with remarkable precision.
Video: Fluorine Bohr Model: The Simple Guide You NEED To See!
Frequently Asked Questions About the Fluorine Bohr Model
Here are some common questions about the fluorine Bohr model to help you understand its structure and properties better.
What does the fluorine Bohr model show?
The fluorine Bohr model is a simplified representation of the fluorine atom, showing its nucleus and electrons arranged in distinct energy levels or shells. It illustrates the basic structure and electron configuration of fluorine, highlighting how its electrons are distributed around the nucleus.
How many electrons does fluorine have in its outermost shell according to the Bohr model?
According to the fluorine Bohr model, fluorine has seven electrons in its outermost shell (also known as the valence shell). This makes fluorine highly reactive as it seeks to gain one more electron to achieve a stable octet configuration, like the noble gases.
Is the fluorine Bohr model a completely accurate depiction of the atom?
No, the fluorine Bohr model is a simplified representation. While useful for understanding basic electron arrangements, it doesn't fully capture the complexities of electron behavior as described by quantum mechanics. For instance, electrons don't orbit the nucleus in fixed paths like planets.
Why is understanding the fluorine Bohr model important?
Understanding the fluorine Bohr model provides a foundational understanding of fluorine's chemical properties and its ability to form compounds. It helps to visualize how fluorine's electron configuration dictates its reactivity and bonding behavior, which are essential concepts in chemistry.