Energy Coupling: Fueling Your Body Naturally
Unlocking the secrets of vitality starts with understanding how your body converts fuel into power, a process deeply rooted in energy coupling. Think of your cells as tiny mitochondria power plants, masterfully orchestrating reactions to keep you energized; Adenosine Triphosphate, ATP, a key molecule, acts as the universal energy currency of the cell, capturing and transferring energy produced from nutrients in your diet and enabling the vast majority of cellular functions; Exercise, a purposeful activity that promotes metabolic health, improves energy coupling by enhancing mitochondrial function and energy transfer, leading to efficient energy expenditure and improved physical performance. The Krebs cycle, also known as the citric acid cycle, is a pivotal sequence of chemical reactions that occur in the mitochondria, playing a crucial role in energy coupling.
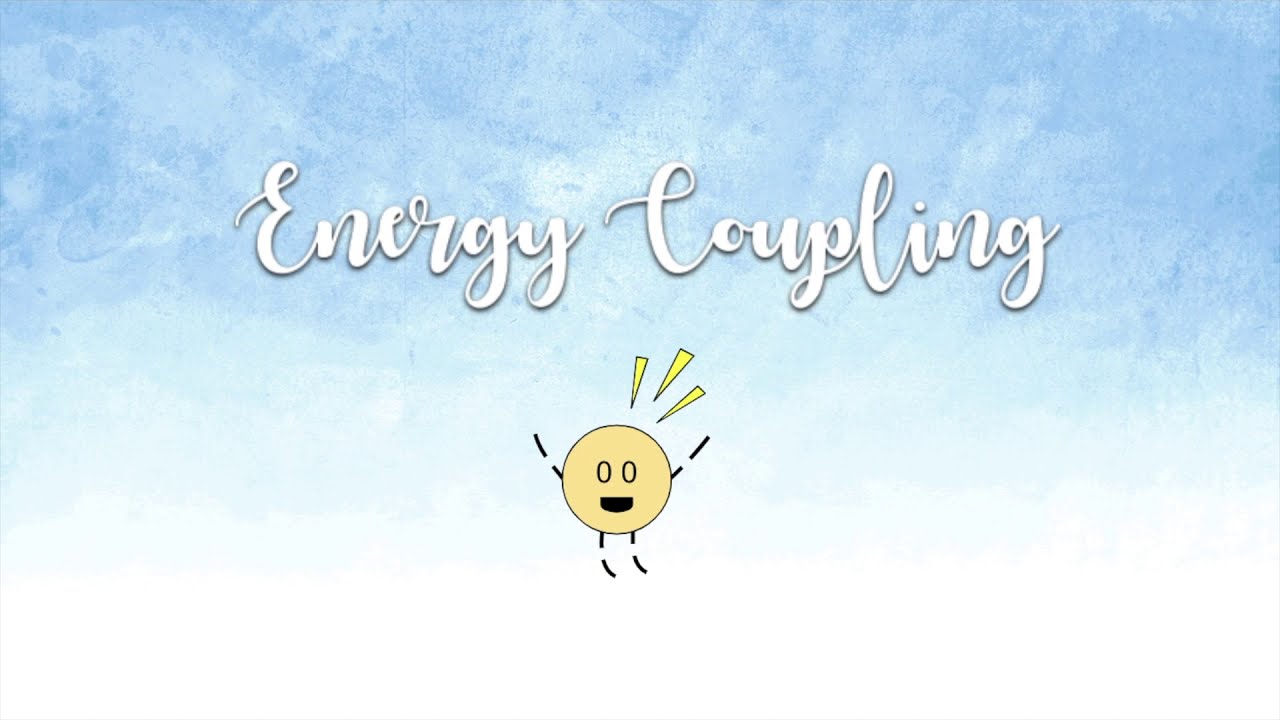
Image taken from the YouTube channel Jeanne Tran , from the video titled Energy Coupling .
The Intricate World of Biological Energy Dynamics
Life, in all its vibrant forms, is fundamentally powered by energy. Think of it as the invisible force driving every process, from the flutter of a hummingbird's wings to the complex computations occurring in your brain right now. This section delves into the fascinating realm of biological energy dynamics, exploring how living systems capture, transform, and utilize energy to sustain themselves.
Energy Coupling: The Cornerstone of Biological Efficiency
Imagine trying to build a house without the right tools or a blueprint. It would be chaotic and inefficient, right? Similarly, many essential biological processes require an initial investment of energy to get going.
This is where energy coupling comes into play. It's the ingenious mechanism by which cells link energy-releasing (exergonic) reactions with energy-requiring (endergonic) reactions.
This coupling allows cells to harness the energy liberated from one process to fuel another, preventing energy waste and maximizing efficiency.
It's like using the energy from burning wood to power a steam engine – a clever way to make the most of available resources.
ATP: The Universal Energy Currency
If energy coupling is the strategy, then Adenosine Triphosphate (ATP) is the currency. ATP is a small, yet mighty, molecule that serves as the primary energy carrier in all living cells. Think of it as the cell's equivalent of cash – readily available and universally accepted.
What Makes ATP So Special?
ATP's power lies in its unique structure: an adenosine molecule attached to three phosphate groups. The bonds between these phosphate groups are high-energy bonds.
When one of these bonds is broken through a process called hydrolysis (adding water), energy is released. This energy can then be used to power a wide range of cellular activities.
It's like breaking a dam and releasing a surge of water to drive a turbine.
The Multifaceted Roles of ATP
ATP is not a one-trick pony; it's a versatile workhorse that powers a diverse array of cellular functions.
-
Mechanical Work: ATP fuels muscle contraction, enabling movement.
-
Transport Work: ATP powers the movement of molecules across cell membranes, maintaining the right balance of substances inside and outside the cell.
-
Chemical Work: ATP provides the energy needed to synthesize complex molecules like proteins and DNA, essential for growth and repair.
In essence, ATP is the lifeblood of the cell, enabling it to perform the countless tasks necessary for survival. Without ATP, life as we know it would simply cease to exist.
Core Components: Molecules, Processes, and Catalysts Driving Energy Flow
Life, in all its vibrant forms, is fundamentally powered by energy. Think of it as the invisible force driving every process, from the flutter of a hummingbird's wings to the complex computations occurring in your brain right now. This section delves into the fascinating realm of biological energy dynamics, exploring the core components that make it all possible.
We'll uncover the essential molecules, processes, and catalysts that orchestrate energy transformations within living organisms. It's a microscopic world of amazing efficiency and intricate choreography!
Molecules of Energy: ATP and ADP
At the heart of cellular energy lies Adenosine Triphosphate, or ATP. It's the cell's primary energy currency, ready to be spent on various tasks. Think of it like a tiny battery, always ready to power up a reaction!
But let's not forget its close relative, Adenosine Diphosphate, or ADP. ADP is essentially the "uncharged" form of ATP.
Understanding their relationship is key to grasping how energy flows within a cell.
ATP Hydrolysis: Releasing the Energy
So, how does ATP actually do work? The magic happens through a process called hydrolysis. It is when ATP releases energy.
Hydrolysis involves breaking one of the phosphate bonds in ATP. This cleavage process converts it into ADP and a free phosphate group.
This bond breaking releases energy, which can then be harnessed to drive other cellular processes. That energy is used for muscle contraction, nerve impulse transmission, and protein synthesis, just to name a few. It's a beautiful example of cause and effect at the molecular level!
Phosphorylation: Recharging the Battery
Now, what happens to the "used" ADP? The cell cleverly "recharges" it back into ATP through a process called phosphorylation.
Phosphorylation involves adding a phosphate group back onto ADP, regenerating ATP.
This process requires energy, of course! This energy can come from the breakdown of food molecules, or even from sunlight in photosynthetic organisms. It's a continuous cycle of energy expenditure and replenishment, keeping the cellular machinery running smoothly.
Fundamental Processes: Hydrolysis, Redox Reactions, and Metabolic Pathways
Beyond the ATP/ADP cycle, several other key processes play crucial roles in energy dynamics. These processes include hydrolysis, redox reactions, and metabolic pathways.
Hydrolysis: Breaking Down to Build Up
We've already touched on hydrolysis with ATP. It’s the process by which water is used to break down a molecule.
This releases energy, and it’s crucial not only for ATP breakdown. But also for breaking down larger molecules like carbohydrates and proteins. It’s a fundamental way for cells to access the energy stored within these molecules.
Redox Reactions: The Electron Shuffle
Oxidation-reduction reactions, or redox reactions, are essential for unlocking the energy stored in the food we eat. These reactions involve the transfer of electrons from one molecule to another.
One molecule loses electrons (oxidation) and another gains electrons (reduction). These reactions are always coupled!
Think of it like a seesaw: one side goes up as the other goes down. These electron transfers release energy that cells can then use to generate ATP.
Metabolic Pathways: The Energy Flow Network
Within a cell, reactions rarely occur in isolation. They are usually organized into interconnected sequences called metabolic pathways.
Each step in a pathway is catalyzed by a specific enzyme. That enzyme transforms one molecule into another, until the final product is reached.
These pathways are like carefully designed assembly lines, ensuring that energy flows efficiently and is used where it's needed. Metabolic pathways can be catabolic (breaking down molecules to release energy) or anabolic (building complex molecules using energy).
Enzymes: The Catalytic Conductors
No discussion of energy dynamics would be complete without mentioning enzymes. These biological catalysts dramatically speed up metabolic reactions, making life as we know it possible.
Without enzymes, reactions would occur too slowly to sustain life!
Enzymes work by lowering the activation energy of a reaction, the energy needed to start the reaction. They do so by binding to the reactant molecules (substrates) and bringing them together in a way that facilitates the reaction.
Coenzymes: The Enzyme's Helpers
Some enzymes require assistance from other molecules called coenzymes.
Coenzymes bind to the enzyme and participate in the reaction, often by carrying electrons or chemical groups. Many vitamins act as coenzymes!
For example, NAD+ and FAD are coenzymes that play a crucial role in redox reactions during cellular respiration. They help enzymes catalyze reactions involved in energy coupling.
Together, enzymes and coenzymes form a powerful team, efficiently managing the energy transformations within the cell.
Cellular Respiration: Harnessing Energy from Food
Life, in all its vibrant forms, is fundamentally powered by energy. Think of it as the invisible force driving every process, from the flutter of a hummingbird's wings to the complex computations occurring in your brain right now. This section delves into the fascinating realm of cellular respiration, the intricate process through which cells expertly extract this life-sustaining energy from the food we consume. Get ready to explore how our bodies convert everything from a simple sugar molecule to a complex, energetic force!
Stages of Cellular Respiration
Cellular respiration isn't a single, instantaneous event; it's a meticulously orchestrated series of steps, each playing a vital role in the energy extraction process. Let’s break down these stages, step by fascinating step.
Glycolysis: The Initial Breakdown
Glycolysis, meaning "sugar splitting," is the first act in this energy-generating drama. It occurs in the cell's cytoplasm and involves the breakdown of glucose, a six-carbon sugar, into two molecules of pyruvate, a three-carbon compound.
While glycolysis does yield a small amount of ATP and NADH (another energy-carrying molecule), its primary purpose is to prepare glucose for the subsequent stages. Think of it as the opening scene, setting the stage for the main performance.
Citric Acid Cycle (Krebs Cycle): Unlocking More Potential
Also known as the Krebs cycle, the Citric Acid Cycle takes place within the mitochondria. Here, pyruvate is further processed to extract even more energy.
Through a series of enzymatic reactions, the cycle generates ATP, NADH, and FADH2 (another electron carrier). Perhaps most importantly, it releases carbon dioxide as a byproduct – the same gas we exhale!
The Citric Acid Cycle is a critical turning point, converting chemical energy into forms that can be harnessed in the next stage.
Electron Transport Chain (ETC): The ATP Powerhouse
This is where the real magic happens! The electron transport chain, also located in the mitochondria, is a series of protein complexes that accept electrons from NADH and FADH2, generated in the previous stages.
As these electrons move down the chain, they release energy. This energy is used to pump protons (H+) across the inner mitochondrial membrane, creating an electrochemical gradient.
Think of it like water building up behind a dam, storing potential energy.
Oxidative Phosphorylation: Capturing the Energy
Oxidative phosphorylation is intimately linked to the ETC. The electrochemical gradient established by the electron transport chain drives the synthesis of ATP.
Specifically, the protons flow back across the membrane through an enzyme called ATP synthase, which uses the energy of this flow to attach a phosphate group to ADP, forming ATP.
This process generates the vast majority of ATP produced during cellular respiration.
Chemiosmosis: Powering ATP Synthase
Chemiosmosis is the engine that drives ATP synthase. As protons diffuse down their concentration gradient through ATP synthase, the enzyme rotates, mechanically attaching a phosphate to ADP and generating ATP.
Without the carefully maintained proton gradient, ATP synthase would be unable to function, and the cell’s energy production would grind to a halt. It's an elegant and efficient system.
ATP Synthase: The ATP Factory
ATP synthase is a remarkable molecular machine. It is not simply a passive channel; it's an enzyme that actively uses the proton gradient to catalyze the formation of ATP.
This nanoscale factory is essential for life, cranking out the energy currency that powers all cellular activities. It's a testament to the intricate beauty and efficiency of biological systems.
The Central Role of Mitochondria
Mitochondria are often referred to as the "powerhouses of the cell," and for good reason. These organelles are the primary sites of cellular respiration. Their unique structure, with its folded inner membrane (cristae), maximizes the surface area available for the electron transport chain and ATP synthase.
Without mitochondria, cells would be drastically limited in their ability to produce ATP, severely impacting their ability to function. They are absolutely indispensable for energy production in eukaryotic cells.
Metabolic States: Anabolism and Catabolism - A Dynamic Duo
Life, in all its vibrant forms, is fundamentally powered by energy. Think of it as the invisible force driving every process, from the flutter of a hummingbird's wings to the complex computations occurring in your brain right now. This section delves into the fascinating relationship between anabolism and catabolism, the two opposing yet perfectly synchronized metabolic states that govern how your body manages energy.
These processes are not just about breaking down and building up; they're about maintaining a delicate balance that keeps you alive and thriving. Let's explore this dynamic duo and understand how they work together!
Anabolism: Building Up Life's Structures
Anabolism, in its essence, is the constructive phase of metabolism. It's the set of metabolic processes where simpler molecules are assembled into more complex ones. Think of it like constructing a building: you start with bricks, mortar, and wood, and you combine them to create walls, floors, and roofs.
Similarly, anabolism takes small molecules like amino acids, sugars, and fatty acids and uses them to create proteins, carbohydrates, and lipids. This requires an input of energy, like a construction crew needs fuel to power their equipment.
Key Anabolic Processes:
- Protein Synthesis: Amino acids are joined together to form proteins, essential for virtually all cellular functions.
- DNA Replication: Creating copies of DNA ensures the continuity of genetic information.
- Glycogenesis: Glucose molecules are linked to form glycogen, the storage form of glucose in the liver and muscles.
- Lipogenesis: Fatty acids and glycerol are combined to form triglycerides, the main component of body fat.
Anabolism isn't just about getting bigger; it's about maintaining, repairing, and optimizing your body's structures. Without anabolism, you wouldn't be able to heal wounds, grow new cells, or store energy for future use.
Catabolism: Breaking Down for Energy
Catabolism is the destructive counterpart to anabolism. It involves breaking down complex molecules into simpler ones, releasing energy in the process.
Think of it like dismantling an old building to salvage its materials. Catabolism takes large molecules like carbohydrates, lipids, and proteins and breaks them down into smaller molecules, like glucose, fatty acids, and amino acids, along with a release of energy.
This energy is captured in the form of ATP (adenosine triphosphate), the cell's primary energy currency.
Key Catabolic Processes:
- Glycolysis: Glucose is broken down into pyruvate, yielding ATP and NADH.
- Lipolysis: Triglycerides are broken down into fatty acids and glycerol.
- Proteolysis: Proteins are broken down into amino acids.
- Cellular Respiration: Pyruvate and fatty acids are fully oxidized to produce carbon dioxide, water, and large amounts of ATP.
Catabolism isn't just about tearing things down; it's about providing the energy needed to fuel all of your body's activities. From running a marathon to simply breathing, catabolism makes it all possible.
The Interplay: A Constant Balancing Act
Anabolism and catabolism are constantly working in tandem to maintain homeostasis, a state of balance within the body. When you eat, anabolism is favored, as your body uses the incoming nutrients to build and store.
When you're fasting or exercising, catabolism takes precedence, as your body breaks down stored molecules to release energy.
This constant interplay is regulated by a variety of hormones, enzymes, and other factors, ensuring that your body always has the energy and building blocks it needs to function optimally.
Factors influencing this balance include:
- Diet: The availability of nutrients directly impacts both anabolic and catabolic processes.
- Hormones: Insulin promotes anabolism, while cortisol and glucagon promote catabolism.
- Activity Level: Exercise increases energy demand, shifting the balance towards catabolism.
- Age: As we age, anabolic processes tend to decline, while catabolic processes may become more dominant.
Understanding the interplay between anabolism and catabolism is crucial for maintaining a healthy metabolism and overall well-being. By making informed choices about diet, exercise, and lifestyle, you can support this dynamic duo and ensure that your body has the energy and building blocks it needs to thrive.
Nutrient Metabolism: Fueling the Body's Energy Needs
Life, in all its vibrant forms, is fundamentally powered by energy. Think of it as the invisible force driving every process, from the flutter of a hummingbird's wings to the complex computations occurring in your brain right now. This section delves into the fascinating relationship between the food we eat and the energy our bodies create, with a special focus on how different nutrients are metabolized to keep us going strong.
Primary Fuel Sources: Glucose and Fatty Acids
Our bodies are incredibly adaptable machines, capable of extracting energy from a variety of sources. However, some fuels are favored over others due to their efficiency and availability. Let's take a look at the two main players: glucose and fatty acids.
Glucose: The Cell's Preferred Sugar
Glucose, a simple sugar, is the body’s primary and preferred source of energy for many cells. Think of it as the go-to fuel for quick bursts of activity and essential functions. It’s easily broken down and readily available.
This makes it an ideal choice, particularly for the brain, which has a high energy demand and relies almost exclusively on glucose for fuel.
Fatty Acids: The Power of Fats
Fatty acids, derived from the breakdown of fats (lipids), are another major source of energy. They're like a longer-lasting, more concentrated fuel compared to glucose.
While glucose offers a quick boost, fatty acids provide sustained energy, making them essential for endurance activities and maintaining overall energy balance.
Macronutrient Metabolism: Carbohydrates, Fats, and Proteins
To understand how we harness energy from food, it's crucial to explore macronutrient metabolism. These are the big three: carbohydrates, fats (lipids), and proteins. Each plays a distinct role in fueling our bodies and supporting various functions.
Carbohydrates: The Body's Glucose Reservoir
Carbohydrates are often considered the body’s preferred and most accessible source of glucose. When you eat carbs, your digestive system breaks them down into glucose molecules.
These are then either used immediately for energy or stored as glycogen in the liver and muscles for later use. It is essentially a glucose reservoir!
Fats (Lipids): High-Energy Storage
Fats, or lipids, are high-energy storage molecules. They provide more than twice the energy per gram compared to carbohydrates or proteins!
When the body needs a sustained energy source, it breaks down stored fats into fatty acids. The fatty acids fuel various metabolic processes. This process keeps our energy levels stable over longer periods.
Proteins: Building Blocks and an Emergency Fuel
While primarily known for their role in building and repairing tissues, proteins can also be used as an energy source.
However, the body typically prefers to use carbohydrates and fats first. Proteins are essential for structural and functional roles. They are generally reserved as an energy source when other options are limited.
The Digestive Process: Unlocking Nutrients from Food
The digestive system is the unsung hero of energy metabolism. It is responsible for breaking down the food we eat into usable nutrients.
This complex process involves a series of mechanical and chemical reactions, starting in the mouth and continuing through the stomach, small intestine, and large intestine.
Enzymes play a crucial role in breaking down complex molecules. Complex molecules such as carbohydrates, fats, and proteins into their simpler forms. These simple forms are then absorbed into the bloodstream and transported to cells throughout the body, where they can be used for energy production and other vital functions. Ultimately, the digestive process transforms food into the fuel our bodies need to thrive.
Systemic Integration: The Orchestration of Energy Use Across Organs and Systems
[Nutrient Metabolism: Fueling the Body's Energy Needs Life, in all its vibrant forms, is fundamentally powered by energy. Think of it as the invisible force driving every process, from the flutter of a hummingbird's wings to the complex computations occurring in your brain right now. This section delves into the fascinating relationship between the...]
Maintaining the delicate balance of energy within a complex organism like ourselves requires a symphony of coordinated actions. It's not just about individual cells doing their thing; it's about how different organs and systems communicate and cooperate to ensure everyone has the fuel they need. Let's explore this intricate orchestration, highlighting the key players and their vital roles.
Key Organs in the Energy Orchestra
Each organ plays a specific role in the larger energetic process.
Think of them as specialized instruments in an orchestra, each contributing to the overall harmonious energy balance.
The Brain: A Glucose-Hungry Maestro
The brain, our command center, is remarkably energy-intensive. It relies almost exclusively on glucose to function properly. Even though it only accounts for about 2% of our body weight, it consumes about 20% of our total energy!
This relentless demand underscores the need for a constant, reliable supply of glucose to keep our thoughts sharp and our bodies functioning.
Any disruption to this supply can have serious consequences.
The Liver: The Energy Storage and Distribution Hub
The liver acts as a central hub in energy metabolism, performing many functions. It's an incredible organ, involved in glucose metabolism, storage, and distribution.
After a meal, the liver takes up glucose from the bloodstream and stores it as glycogen.
When blood sugar levels drop, the liver breaks down glycogen and releases glucose back into the circulation, ensuring a steady supply for the brain and other tissues.
It also plays a key role in processing fats and proteins, further contributing to energy homeostasis.
The Pancreas: The Blood Sugar Regulator
The pancreas is vital for controlling blood sugar levels. This is done through the hormones it produces: insulin and glucagon. Think of it as the body's blood sugar thermostat.
Insulin lowers blood sugar by facilitating glucose uptake into cells, while glucagon raises blood sugar by stimulating the liver to release stored glucose.
These two hormones work in tandem to maintain blood glucose within a narrow range, preventing both hyperglycemia (high blood sugar) and hypoglycemia (low blood sugar).
Major Systems Supporting Energy Dynamics
The organs of the body act in concert with larger physiological systems to achieve energy homeostasis.
The Circulatory System: The Nutrient and Oxygen Highway
The circulatory system, with the heart as its engine, acts as a critical transport network. It delivers vital nutrients and oxygen to every cell in the body – the raw materials for energy production.
It also carries away waste products, such as carbon dioxide, preventing them from building up and hindering cellular function.
Without efficient circulation, cells would quickly run out of fuel and become overwhelmed by waste, disrupting energy production.
The Respiratory System: The Oxygen Supplier
The respiratory system, with the lungs at its core, is indispensable for cellular respiration. It provides the oxygen needed for the electron transport chain, the final stage of energy production, and removes carbon dioxide, a waste product of the process.
Efficient gas exchange in the lungs is crucial for maximizing ATP production. Impaired respiratory function can significantly reduce the amount of energy cells can generate.
Maintaining Balance: Homeostasis and Metabolic Rate Regulation
Having explored the intricate dance of energy transfer within cells and the orchestration of energy use across various organs, it's now time to understand how the body maintains a delicate balance in this energy economy. This crucial equilibrium, known as homeostasis, ensures that our cells have the right amount of energy to function optimally, while metabolic rate regulation ensures that energy expenditure matches energy intake.
What is Metabolic Rate?
Metabolic rate, at its core, is the speed at which your body burns calories. It represents the total amount of energy your body uses to carry out all its necessary functions.
Think of it as the engine of your body, constantly running to keep you alive and kicking!
This rate varies depending on a multitude of factors, including age, sex, body composition, activity level, and even genetics.
Basal Metabolic Rate (BMR)
A key component of metabolic rate is the Basal Metabolic Rate (BMR). This represents the minimum amount of energy required to keep your body functioning at rest.
It's the energy needed for essential functions such as breathing, circulation, and maintaining body temperature.
BMR typically accounts for the largest portion of your daily energy expenditure.
Factors Influencing Metabolic Rate
Several factors can influence your metabolic rate, causing it to fluctuate throughout the day and over longer periods.
Age and Gender
Generally, metabolic rate tends to decline with age due to loss of muscle mass and other physiological changes.
Men typically have a higher metabolic rate than women due to their generally greater muscle mass.
Body Composition
Muscle tissue burns more calories than fat tissue, even at rest. Therefore, individuals with a higher muscle mass tend to have a higher metabolic rate.
Activity Level
Physical activity significantly increases metabolic rate. The more active you are, the more calories you burn.
Hormones
Hormones, such as thyroid hormones, play a crucial role in regulating metabolic rate.
An overactive thyroid can lead to an increased metabolic rate, while an underactive thyroid can slow it down.
Homeostasis: The Body's Balancing Act
Homeostasis refers to the body's ability to maintain a stable internal environment despite external fluctuations. It's a dynamic process that involves intricate feedback mechanisms to regulate various physiological parameters.
When it comes to energy balance, homeostasis ensures that energy intake matches energy expenditure over time.
Energy Intake vs. Energy Expenditure
The body carefully monitors energy intake (calories consumed through food) and energy expenditure (calories burned through activity and bodily functions).
When energy intake exceeds energy expenditure, the excess energy is stored as fat.
Conversely, when energy expenditure exceeds energy intake, the body taps into its energy reserves, leading to weight loss.
Hormonal Regulation of Energy Balance
Several hormones play a critical role in regulating energy balance.
Leptin, produced by fat cells, signals to the brain about the body's energy stores, reducing appetite and increasing energy expenditure.
Ghrelin, produced by the stomach, stimulates appetite.
Insulin, produced by the pancreas, helps regulate blood sugar levels and promotes the storage of glucose as glycogen in the liver and muscles.
Maintaining a Healthy Energy Balance
Understanding the interplay between metabolic rate and homeostasis is crucial for maintaining a healthy weight and overall well-being. Here are some practical tips:
-
Regular Exercise: Incorporate both aerobic exercise and strength training to boost your metabolic rate and build muscle mass.
-
Balanced Diet: Consume a balanced diet rich in fruits, vegetables, whole grains, and lean protein to provide your body with the nutrients it needs for optimal energy production.
-
Adequate Sleep: Aim for 7-8 hours of quality sleep per night. Sleep deprivation can disrupt hormonal balance and negatively impact metabolic rate.
-
Stress Management: Practice stress-reducing techniques such as yoga, meditation, or spending time in nature. Chronic stress can lead to hormonal imbalances and weight gain.
By understanding these principles and adopting healthy lifestyle habits, you can effectively support your body's energy dynamics and maintain a vibrant, healthy life.
Imbalances and Disorders: When Energy Systems Fail
Having explored the intricate dance of energy transfer within cells and the orchestration of energy use across various organs, it's now time to understand how the body maintains a delicate balance in this energy economy. This crucial equilibrium, known as homeostasis, ensures that our cells and tissues receive the energy they need to function optimally.
But what happens when this finely tuned system falters?
What happens when the cellular power plants malfunction, or when the pathways that fuel our bodies become disrupted?
The consequences can range from subtle fatigue to severe metabolic disorders, significantly impacting our overall health and well-being.
Let's delve into some of the ways our energy systems can fail and the conditions that may arise.
Metabolic Disorders: Disruptions in Energy Pathways
Metabolic disorders are a diverse group of conditions that disrupt the body's normal metabolic processes. These processes involve a complex series of chemical reactions that convert food into energy and building blocks for cells.
When these pathways are compromised, it can lead to a buildup of toxic substances or a deficiency in essential molecules, resulting in a wide range of health problems.
Mitochondrial Dysfunction: When Power Plants Malfunction
Mitochondria, often dubbed the "powerhouses of the cell," are responsible for generating the majority of ATP (adenosine triphosphate), the primary energy currency of our cells.
When mitochondria malfunction, the consequences can be far-reaching.
Mitochondrial dysfunction can stem from genetic mutations, environmental factors, or oxidative stress.
This can lead to reduced ATP production.
A cascade of effects throughout the body results from this reduced energy output.
Symptoms can vary widely depending on the specific tissues and organs affected, but common manifestations include:
- Fatigue
- Muscle weakness
- Neurological problems
- Organ dysfunction
Think of it like a city experiencing rolling blackouts due to a failing power grid; essential functions begin to degrade and systems start to shut down.
Energy-Related Conditions: Manifestations of Energy Depletion
While metabolic disorders represent fundamental disruptions in energy pathways, other conditions can arise as a result of overall energy depletion or inefficient energy utilization.
These energy-related conditions often manifest as persistent and debilitating symptoms that significantly impact quality of life.
Fatigue: More Than Just Feeling Tired
We all experience fatigue from time to time. However, chronic fatigue is more than just feeling tired after a long day.
It's a persistent and overwhelming sense of exhaustion that isn't relieved by rest.
It can significantly interfere with daily activities and overall well-being.
While the exact causes of chronic fatigue are complex and multifactorial, reduced energy production often plays a significant role.
When cells are unable to generate enough ATP, the body's systems struggle to function optimally. This struggle manifests as:
- Persistent tiredness
- Lack of motivation
- Difficulty concentrating
It's important to differentiate chronic fatigue from simple tiredness. If fatigue persists despite adequate rest and healthy lifestyle habits, it's crucial to seek medical evaluation to rule out underlying conditions.
By understanding the intricate mechanisms that govern energy production and utilization, we can take proactive steps to support our body's energy needs. We can also recognize the signs and symptoms of imbalances, disorders, or energy-related conditions. Seeking appropriate medical attention is very important. This way, we can prioritize our overall health and well-being.
Oxidative Stress and Protection: Combating Free Radicals
Having explored the intricate dance of energy transfer within cells and the orchestration of energy use across various organs, it's now time to understand a key threat to these processes: oxidative stress. This section will uncover the causes of oxidative stress and the remarkable role of antioxidants in safeguarding our cells from the damaging effects of free radicals. Let's dive in!
Understanding Free Radicals and Their Cellular Impact
Imagine your cells constantly facing a barrage of tiny, unstable molecules searching for stability. These molecules are called free radicals, and they can wreak havoc if left unchecked. Understanding their origin and impact is crucial for protecting our health.
The Nature of Oxidative Stress
Oxidative stress arises when there's an imbalance between the production of free radicals and the body's ability to neutralize them with antioxidants. Think of it like a scale: on one side, you have free radicals; on the other, antioxidants. When free radicals outweigh antioxidants, the scale tips, leading to cellular damage.
Free radicals are naturally produced during normal metabolic processes, like cellular respiration. However, their production can be significantly increased by external factors such as pollution, smoking, alcohol consumption, radiation, and even intense exercise.
This overproduction is what pushes the cell into a state of oxidative stress.
How Free Radicals Damage Cells
So, what exactly do these free radicals do? Their primary goal is to achieve stability by snatching electrons from other molecules. This electron theft can damage vital cellular components such as:
-
DNA: Leading to mutations and increasing the risk of diseases like cancer.
-
Proteins: Disrupting their function and leading to cellular dysfunction.
-
Lipids (fats): Contributing to the formation of plaques in arteries, increasing the risk of cardiovascular diseases.
The cascade of damage initiated by free radicals can lead to inflammation, accelerated aging, and the development of various chronic diseases.
Antioxidants: Our Body's Defense Force
Fortunately, our bodies are not defenseless against the onslaught of free radicals. Antioxidants act as our body's natural defense force, neutralizing free radicals by donating electrons without becoming unstable themselves.
How Antioxidants Work
Antioxidants work by acting as electron donors. They willingly give up an electron to stabilize the free radical, effectively neutralizing it and preventing it from damaging other molecules.
This generous act of electron donation halts the chain reaction of cellular damage.
Sources of Antioxidants
We can obtain antioxidants from various sources, primarily through our diet. Key sources of antioxidants include:
-
Fruits and Vegetables: Especially colorful ones like berries, spinach, kale, and bell peppers.
-
Vitamins: Vitamin C, Vitamin E, and beta-carotene are potent antioxidants.
-
Minerals: Selenium, manganese, and zinc play crucial roles in antioxidant enzyme systems.
-
Other Dietary Compounds: Found in green tea, dark chocolate, and other plant-based foods.
It's important to note that antioxidant supplements should be taken with caution and under the guidance of a healthcare professional, as excessive intake can sometimes be harmful. A balanced diet rich in a variety of fruits and vegetables is usually the best approach.
The Importance of Balance and Protection
Maintaining a balance between free radical production and antioxidant defense is essential for optimal health. By understanding the sources of oxidative stress and incorporating antioxidant-rich foods into our diet, we can support our body's natural defenses and protect our cells from damage.
Remember, a proactive approach to managing oxidative stress is an investment in your long-term health and well-being!
Video: Energy Coupling: Fueling Your Body Naturally
Energy Coupling FAQs: Fueling Your Body Naturally
What does "fueling your body naturally" actually mean?
It means choosing whole, unprocessed foods like fruits, vegetables, and lean proteins. These provide the nutrients your body needs for optimal function. This supports efficient energy coupling and overall health.
How does energy coupling work with my food?
Energy coupling is how your body uses energy released from food to power other processes. Enzymes break down the food into usable forms, like glucose. This fuel then powers reactions like muscle contraction or cell growth.
Why is natural fueling better than relying on processed foods or supplements?
Natural foods contain a range of vitamins, minerals, and phytonutrients processed foods often lack. These nutrients are critical for effective energy coupling and supporting healthy metabolic processes. Supplements rarely offer the same holistic benefits.
How can I improve my energy levels through natural fueling?
Focus on a balanced diet with consistent meal times and adequate hydration. Avoid skipping meals and prioritize complex carbohydrates over simple sugars for sustained energy. These actions ensure your body has what it needs for optimal energy coupling.
So, there you have it! Understanding energy coupling might sound complicated, but it's really just about how your body cleverly shuffles energy around to keep you going strong. Pay attention to those natural energy boosters and listen to your body's needs, and you'll be well on your way to maximizing that internal power source. Cheers to fueling up the right way!