Unlock ClF3 Molecular Geometry: Simple Guide!
Understanding the VSEPR theory allows chemists to predict the shapes of molecules, and the clf3 molecular geometry offers a compelling example. The shape, resulting from minimizing electron pair repulsion, affects its reactivity. The central chlorine atom's bonding with three fluorine atoms and two lone pairs creates a T-shaped geometry, which differs significantly from more symmetrical arrangements. Analyzing spectroscopic data is crucial for experimentally confirming the predicted clf3 molecular geometry and validating theoretical models.
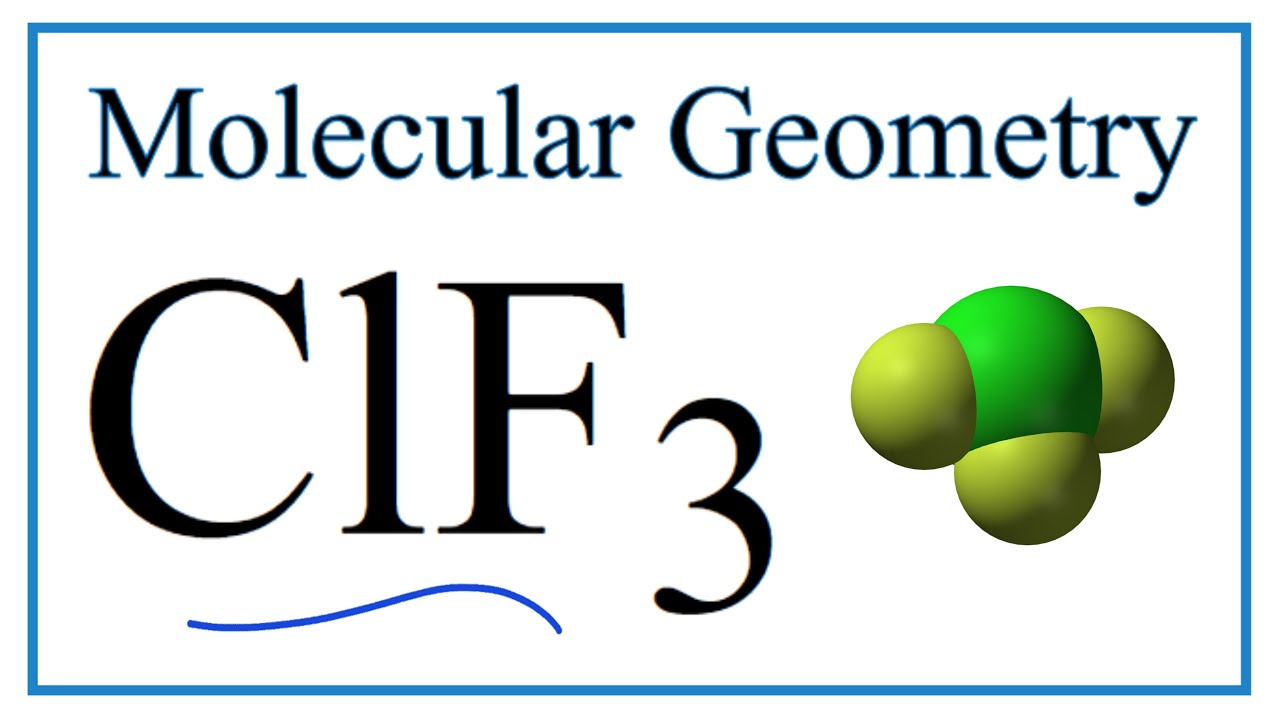
Image taken from the YouTube channel Wayne Breslyn (Dr. B.) , from the video titled ClF3 (Chlorine trifluoride) Molecular Geometry, Bond Angles,& Electron Geometry .
Chlorine Trifluoride (ClF3) is a fascinating and highly reactive interhalogen compound. Its most notable application lies in its potency as a fluorinating agent, often used in industrial processes where strong oxidizing power is required. But beyond its practical applications, ClF3 presents a compelling case study in molecular geometry.
This article sets out to dissect the molecular geometry of ClF3. We aim to present a clear and accessible explanation of why this molecule adopts its specific three-dimensional arrangement.
Understanding molecular geometry is more than just drawing shapes. It’s a cornerstone of chemistry. The shape of a molecule dictates its physical properties, such as boiling point and solubility.
Furthermore, molecular geometry is intrinsically linked to a molecule’s chemical reactivity. The way a molecule interacts with other molecules depends significantly on the spatial arrangement of its atoms. ClF3, with its unusual T-shaped geometry, serves as an excellent example to illustrate these principles.
The Significance of ClF3
ClF3 stands out due to its hypervalent nature, meaning the central chlorine atom appears to have more than the eight valence electrons typically allowed by the octet rule. This characteristic, coupled with the presence of lone pairs, profoundly influences its geometry.
Its geometry deviates from simpler, more symmetrical structures. This makes it a valuable tool for understanding the nuances of Valence Shell Electron Pair Repulsion (VSEPR) theory.
Objective: Demystifying ClF3's Geometry
The primary objective of this article is to elucidate the molecular geometry of ClF3 in a straightforward and easy-to-understand manner.
We will carefully walk through the steps required to predict its shape. This includes constructing the Lewis structure, applying VSEPR theory, and explaining the influence of lone pairs on its final T-shaped configuration.
By the end of this discussion, you will be equipped with the knowledge to confidently predict the geometry of ClF3 and similar molecules.
Molecular Geometry: A Gateway to Understanding
Molecular geometry is not an abstract concept. It has tangible implications in predicting chemical behavior.
The shape of a molecule directly impacts its polarity, its ability to interact with solvents, and its susceptibility to chemical reactions.
A molecule's geometry is crucial in drug design. It dictates how a drug molecule binds to its target receptor.
In materials science, the arrangement of atoms determines the properties of materials. Understanding molecular geometry is therefore essential across various scientific disciplines. ClF3 serves as an excellent example. By investigating it, we hope to inspire further exploration into the world of molecular shapes and their profound effects on the world around us.
Fundamentals: Central Atom and Electron Domains
Before diving into the specifics of ClF3's T-shaped structure, it's vital to establish a solid foundation in the core principles that govern molecular geometry. Understanding the roles of the central atom and electron domains is paramount to grasping the application of VSEPR theory.
The Central Role of Chlorine
In any molecule, the central atom serves as the anchor point, dictating the overall spatial arrangement of the other atoms.
In ClF3, chlorine (Cl) takes center stage.
Its ability to accommodate more than the usual octet of electrons makes it uniquely suited to form bonds with three fluorine atoms while also housing lone pairs, a key factor in determining the molecule's shape.
Chlorine's position as the central atom isn't arbitrary. It's determined by its lower electronegativity compared to fluorine and its capacity to form more bonds.
Understanding Electron Domains
The concept of electron domains is crucial. An electron domain is simply a region around the central atom where electrons are likely to be found.
These domains can be either bonding pairs (electrons shared in a covalent bond with another atom) or non-bonding pairs, also known as lone pairs (electrons that belong exclusively to the central atom).
Both bonding and non-bonding electron pairs exert repulsive forces on each other, influencing the molecule's three-dimensional structure. The arrangement that minimizes these repulsive forces is what determines the observed molecular geometry.
In ClF3, there are five electron domains around the central chlorine atom: three bonding pairs with the fluorine atoms and two lone pairs.
This abundance of electron domains dictates a spatial arrangement that deviates significantly from simpler molecular shapes.
Fluorine as the Ligand
While chlorine is the central player, fluorine (F) atoms act as ligands. They are directly bonded to the central atom.
Fluorine's high electronegativity plays a role in polarizing the Cl-F bonds, but its primary contribution to the molecular geometry is through its bonding interactions with the central chlorine atom.
These bonds, as part of the electron domains around chlorine, contribute to the overall repulsive forces that dictate the molecule's final shape.
Constructing the Lewis Structure of ClF3: A Step-by-Step Guide
Having established the foundational roles of the central atom and electron domains, we now move towards visualizing the arrangement of these components in ClF3. Accurately constructing the Lewis structure is essential, as it serves as the blueprint for predicting molecular geometry using VSEPR theory. This process involves a systematic approach, ensuring all valence electrons are accounted for and arranged to minimize energy.
Calculating Total Valence Electrons: The Foundation
The first crucial step is determining the total number of valence electrons present in the ClF3 molecule. This calculation dictates how many electrons must be depicted in the Lewis structure, ensuring a complete and accurate representation.
Chlorine (Cl) belongs to Group 17 (VIIA) and contributes 7 valence electrons. Fluorine (F), also in Group 17, contributes 7 valence electrons as well.
Since there are three fluorine atoms, their total contribution is 3 7 = 21 valence electrons. Adding chlorine's contribution, the total number of valence electrons in ClF3 is 7 + 21 = 28 valence electrons
**.
Placing Atoms and Drawing Single Bonds
Next, arrange the atoms in a way that reflects the connectivity of the molecule. As we've established, chlorine (Cl) is the central atom, and the three fluorine (F) atoms are bonded to it.
Place Cl in the center and surround it with three F atoms. Draw single bonds between the central chlorine atom and each of the fluorine atoms. Each single bond represents two shared electrons.
At this stage, three single bonds have been drawn, accounting for 3** 2 = 6 valence electrons. Subtracting these from the total (28 - 6), leaves 22 electrons still to be placed.
Distributing Remaining Electrons as Lone Pairs
With the initial bonds in place, the remaining 22 valence electrons must be distributed as lone pairs to satisfy the octet rule (or expanded octet in the case of chlorine).
Start by placing lone pairs around the fluorine atoms until each has a complete octet (8 electrons). Each fluorine atom already has two electrons from the single bond. Therefore, add three lone pairs (6 electrons) to each fluorine atom.
This accounts for 3 fluorine atoms * 6 electrons/atom = 18 electrons. Subtracting these from the remaining electrons (22 - 18) leaves 4 electrons.
These remaining 4 electrons are then placed as lone pairs on the central chlorine atom. This gives chlorine a total of 10 electrons around it (three from the bonding pairs and four from the lone pairs).
Checking for Octet Rule Satisfaction (or Expansion)
Finally, review the Lewis structure to confirm octet rule satisfaction or expansion. Each fluorine atom should have an octet (8 electrons), and the central chlorine atom can have more than an octet due to its ability to expand its valence shell.
Each fluorine atom in ClF3 has 8 electrons (2 from the single bond and 6 from the three lone pairs). The central chlorine atom has 10 electrons (6 from the three bonding pairs and 4 from the two lone pairs). This expansion is permissible for chlorine and other elements in the third period and beyond.
Correct Placement of Lone Pairs and Bond Pairs
The accurate placement of lone pairs and bond pairs around the central chlorine atom is paramount. Remember, lone pairs exert a greater repulsive force than bonding pairs, significantly influencing the molecule's overall shape.
In ClF3, the two lone pairs on the central chlorine atom will influence the spatial arrangement of the fluorine atoms, causing deviations from ideal bond angles. The position of these lone pairs is not arbitrary; they arrange themselves to minimize repulsion.
Determining Bond Pairs and Lone Pairs on the Central Atom
Identifying the number of bond pairs and lone pairs on the central atom is critical for applying VSEPR theory.
In ClF3, the central chlorine atom has three bond pairs (each shared with a fluorine atom) and two lone pairs (non-bonding electron pairs belonging solely to chlorine).
This specific arrangement of three bond pairs and two lone pairs around the central chlorine atom dictates the T-shaped molecular geometry of ClF3, which we will explore in detail in the subsequent sections.
Having carefully constructed the Lewis structure and meticulously placed bonding and non-bonding electron pairs, we now possess the necessary foundation to predict the three-dimensional shape of ClF3. This is where the Valence Shell Electron Pair Repulsion (VSEPR) theory comes into play, providing a powerful framework for understanding molecular geometry.
VSEPR Theory: Predicting Molecular Shape
The Valence Shell Electron Pair Repulsion (VSEPR) theory serves as a cornerstone in predicting the shapes of molecules. It's rooted in a simple but profound principle: electron pairs, whether involved in bonding or existing as lone pairs, repel each other.
This repulsion dictates that electron pairs will arrange themselves around a central atom in a way that minimizes these repulsive forces, thus determining the molecule's geometry. The central idea is that molecules adopt shapes that maximize the distance between regions of high electron density.
Fundamental Principles of VSEPR Theory
At its core, VSEPR theory hinges on the notion that electron domains around a central atom will arrange themselves to be as far apart as possible. An electron domain can be a single bond, a double bond, a triple bond, or a lone pair of electrons. All of these are regions of high electron density.
The strength of repulsion varies, with lone pair-lone pair repulsions being the strongest, followed by lone pair-bond pair repulsions, and finally, bond pair-bond pair repulsions being the weakest. This difference in repulsive strength significantly impacts the final molecular geometry, causing deviations from ideal bond angles.
Applying VSEPR: The AXnEm Notation
To systematically apply VSEPR theory, the AXnEm notation is employed, providing a concise description of the electronic environment around the central atom. Let's break down each component of this notation:
-
A: Represents the central atom in the molecule. In the case of ClF3, A is chlorine (Cl).
-
X: Represents the number of atoms bonded to the central atom, also known as the number of bonding pairs. In ClF3, chlorine is bonded to three fluorine atoms, so X = 3.
-
n: Indicates the number of atoms (X) bonded to the central atom (A). As we established, n = 3 for ClF3.
-
E: Represents the number of lone pairs of electrons on the central atom. These are non-bonding pairs that still influence the molecule's shape.
-
m: Indicates the number of lone pairs (E) on the central atom (A). In the Lewis structure of ClF3, we identified two lone pairs on the chlorine atom, so m = 2.
Therefore, the AXnEm notation for ClF3 is AX3E2. This notation tells us that there are three atoms bonded to the central chlorine atom and two lone pairs residing on the central chlorine atom. This specific arrangement of bonding pairs and lone pairs is what ultimately dictates the T-shaped molecular geometry of ClF3, which we will explore in the following section.
Having carefully constructed the Lewis structure and meticulously placed bonding and non-bonding electron pairs, we now possess the necessary foundation to predict the three-dimensional shape of ClF3. This is where the Valence Shell Electron Pair Repulsion (VSEPR) theory comes into play, providing a powerful framework for understanding molecular geometry.
ClF3's T-Shaped Geometry: The Decisive Role of Lone Pairs
The molecular geometry of Chlorine Trifluoride (ClF3) is a fascinating case study in how lone pairs of electrons exert a profound influence on molecular shape. VSEPR theory predicts that ClF3 will adopt a T-shaped geometry, a departure from the more symmetrical arrangements we might initially expect. But why this specific arrangement, and what role do lone pairs play in dictating it?
Why Not Trigonal Planar? Understanding Electron Domain Geometry
With five electron domains around the central chlorine atom (three bonding pairs and two lone pairs), the electron domain geometry is trigonal bipyramidal. This means the electron domains arrange themselves as if pointing to the corners of a trigonal bipyramid. However, molecular geometry only considers the arrangement of atoms.
If ClF3 were simply trigonal planar, with the three fluorine atoms arranged symmetrically around the chlorine, and the two lone pairs occupying positions above and below the plane, it would seem, at first glance, like a viable structure. However, this arrangement fails to account for the critical differences in repulsive forces.
Lone Pair Repulsion: The Key to T-Shape Formation
The crucial factor driving ClF3's T-shape is the disproportionate repulsive force exerted by lone pairs of electrons. Lone pairs, being more diffuse than bonding pairs, exert a stronger repulsive force on neighboring electron domains.
VSEPR theory dictates that these repulsive forces must be minimized to achieve the most stable molecular geometry. This is achieved when the lone pairs occupy the equatorial positions of the trigonal bipyramid.
This arrangement minimizes the number of 90° interactions between lone pairs and bonding pairs.
The Equatorial Lone Pair Preference
Placing the lone pairs in the axial positions would result in each lone pair having three 90° interactions with bonding pairs.
By placing the lone pairs in the equatorial positions, each lone pair only has two 90° interactions with bonding pairs. This arrangement reduces overall repulsion.
With the lone pairs occupying two of the three equatorial positions, the three fluorine atoms are forced into the remaining two axial positions and one equatorial position, resulting in the characteristic T-shape.
Visualizing the T-Shape and Lone Pair Placement
[Include a diagram here depicting the T-shaped molecular geometry of ClF3. The diagram should clearly show the central chlorine atom, the three fluorine atoms arranged in a T-shape, and the two lone pairs occupying the equatorial positions of the trigonal bipyramidal electron domain geometry.]
The diagram should illustrate how the two lone pairs reside on the same plane as one of the fluorine atoms, effectively "pushing" the other two fluorine atoms into a linear arrangement, forming the stem of the "T."
This visual representation reinforces the concept that the arrangement of atoms is determined by minimizing electron pair repulsion, with lone pairs playing a decisive role. The position of the lone pairs is key in determining the molecular shape.
Having established ClF3’s T-shaped geometry as a direct consequence of lone pair repulsion, the next logical step is to examine the specific bond angles within the molecule and to understand how this unique arrangement contributes to its overall polarity. These factors are critical for predicting ClF3's interactions with other chemical species.
Bond Angles and Molecular Polarity in ClF3
Distorted Bond Angles: The Lone Pair Effect
In an idealized T-shaped geometry, we would expect bond angles of precisely 90° between the central atom and the surrounding ligands. However, ClF3 deviates from this ideal due to the presence of two lone pairs on the chlorine atom.
Lone pairs exert a greater repulsive force than bonding pairs, effectively "squeezing" the fluorine atoms closer together.
This repulsion results in bond angles less than 90°.
Experimentally, the F-Cl-F bond angles in ClF3 are found to be approximately 87.5°.
This seemingly small deviation has significant consequences for the molecule's properties. The impact on molecular polarity is one key aspect.
Molecular Polarity: A Result of Asymmetry
Molecular polarity arises from an uneven distribution of electron density within a molecule.
This uneven distribution is caused by differences in electronegativity between the atoms and by the molecule's geometry.
Fluorine is significantly more electronegative than chlorine, meaning it attracts electrons more strongly.
In a perfectly symmetrical molecule, these individual bond dipoles might cancel each other out, resulting in a nonpolar molecule.
However, the T-shaped geometry of ClF3 prevents this cancellation.
The two lone pairs contribute significantly to the overall electron density distribution, creating a region of high negative charge on the chlorine atom opposite the fluorine atoms.
Understanding the Polarity of ClF3
The bond dipoles of the three Cl-F bonds do not completely cancel, creating a net dipole moment.
This means that ClF3 is a polar molecule, even though it contains highly electronegative fluorine atoms.
The direction of the overall dipole moment points from the central chlorine atom towards the region between the two axial fluorine atoms, reflecting the pull of electron density towards these more electronegative atoms.
This polarity is crucial in determining ClF3's reactivity and its interactions with other polar molecules or ions.
For instance, ClF3 can act as a Lewis acid, accepting electron pairs from Lewis bases due to the electron-deficient character of the chlorine atom.
Video: Unlock ClF3 Molecular Geometry: Simple Guide!
FAQs About ClF3 Molecular Geometry
Here are some frequently asked questions to help you better understand the molecular geometry of ClF3.
What is the shape of the ClF3 molecule?
ClF3 has a T-shaped molecular geometry. This is because it has three bonding pairs and two lone pairs of electrons around the central chlorine atom. These lone pairs influence the overall shape, pushing the fluorine atoms into a "T" configuration.
Why isn't ClF3 tetrahedral if it has four areas of electron density?
While ClF3 has five areas of electron density (three bonding pairs and two lone pairs), the lone pairs exert a stronger repulsive force than bonding pairs. This distorts the geometry from the ideal tetrahedral shape that you would find in a molecule with four bonding pairs and no lone pairs. This lone pair repulsion is key to understanding the clf3 molecular geometry.
How do the lone pairs affect the bond angles in ClF3?
The two lone pairs in ClF3 push the fluorine atoms closer together than they would be in a perfectly symmetrical arrangement. This reduces the F-Cl-F bond angle slightly from the ideal 90° that might be expected in a perfect T-shape, leading to slightly less than 90° bond angles in the clf3 molecular geometry.
What's the difference between molecular geometry and electron geometry for ClF3?
Electron geometry considers all electron pairs (both bonding and lone pairs), so ClF3's electron geometry is trigonal bipyramidal. Molecular geometry, however, only describes the arrangement of atoms. Since the lone pairs aren't visible in the molecular structure, clf3 molecular geometry is described as T-shaped.