Biomass Calculation: Easy Guide for Sustainable Living
Understanding how to calculate biomass is crucial for assessing the potential of renewable energy sources. The U.S. Department of Energy (DOE) emphasizes the importance of accurately determining biomass yields for optimizing energy production. Ecosystem analysis relies heavily on biomass quantification to understand carbon cycling and environmental impact. Several remote sensing techniques, using tools like Normalized Difference Vegetation Index (NDVI), can assist in estimating biomass over large areas. This guide offers practical methods for everyone to leverage biomass calculations for a more sustainable future.
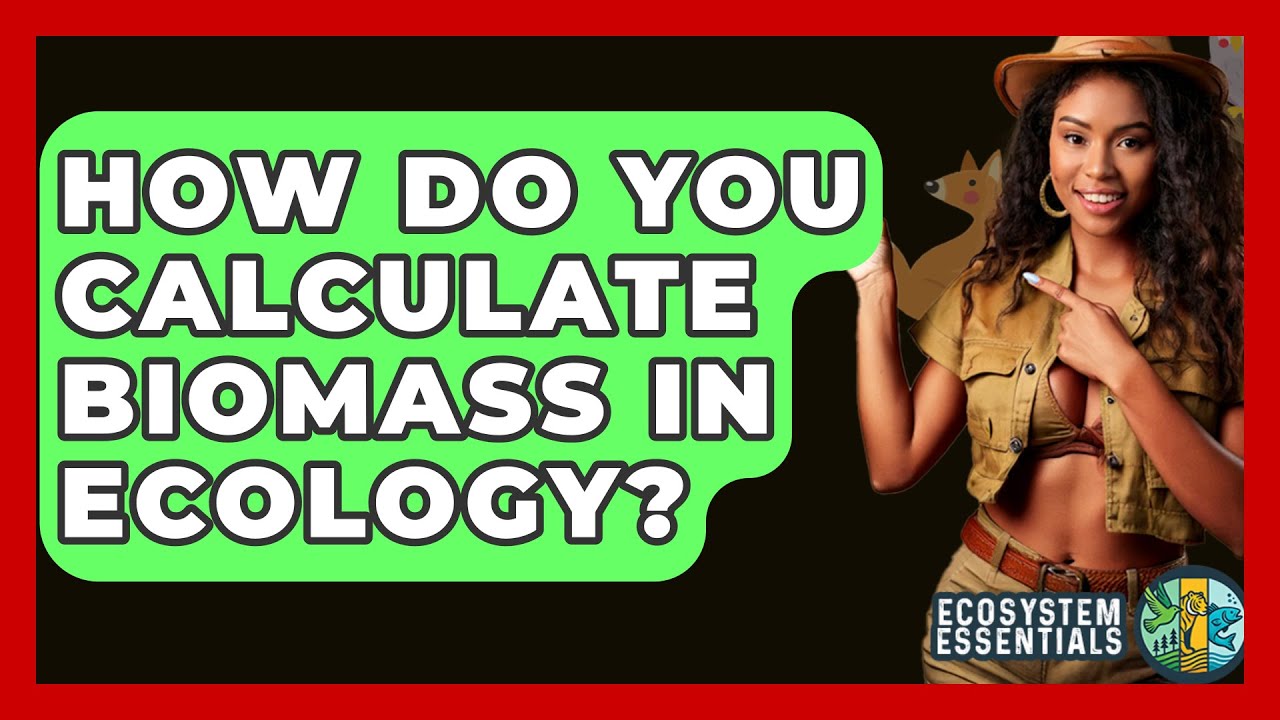
Image taken from the YouTube channel EcosystemEssentials , from the video titled How Do You Calculate Biomass In Ecology? - Ecosystem Essentials .
Biomass stands as a cornerstone of sustainable living and renewable energy strategies. It represents a vital link to a future powered by resources that replenish naturally, contrasting sharply with finite fossil fuels.
Understanding what biomass is, and crucially, how much of it we have, is paramount.
Accurate biomass calculation is essential for gauging the true potential of this renewable resource, and for its effective deployment across a spectrum of applications.
From mitigating climate change to boosting energy efficiency, the responsible management of biomass, guided by precise data, offers a pathway toward a more sustainable world.
Defining Biomass: Nature's Renewable Storehouse
Biomass, in its simplest form, is any organic matter derived from living or recently living organisms.
This encompasses a wide array of materials, broadly categorized as follows:
-
Wood and woody biomass: Trees, shrubs, and forestry residues are all included in this category.
-
Agricultural residues: Crop stalks, leaves, and other leftover plant material from harvesting.
-
Dedicated energy crops: Plants specifically grown for energy production, such as switchgrass or miscanthus.
-
Algae and aquatic plants: Biomass sourced from marine and freshwater environments.
-
Organic waste: Including municipal solid waste, animal waste, and food processing byproducts.
This diversity is one of biomass's strengths, allowing it to be sourced from a multitude of environments and converted into a range of energy products.
Biomass: A Key Renewable Energy Source
As a renewable energy source, biomass holds immense potential. Unlike fossil fuels that take millions of years to form, biomass can be replenished within a human lifespan.
It serves as a versatile energy source, capable of being transformed into:
-
Heat: Direct combustion for heating homes and industrial processes.
-
Electricity: Burning biomass to generate steam, which drives turbines.
-
Liquid biofuels: Converting biomass into fuels like ethanol and biodiesel for transportation.
-
Biogas: Anaerobic digestion of biomass to produce methane-rich biogas for heating, electricity, or vehicle fuel.
The carbon neutrality of biomass is a key argument in its favor. While burning biomass does release CO2, it is ideally offset by the CO2 absorbed by the plants during their growth. When managed responsibly, this results in a closed carbon cycle, minimizing the overall contribution to greenhouse gas emissions.
The Significance of Accurate Biomass Calculation
Accurate biomass calculation is not merely an academic exercise; it is a prerequisite for effective sustainability planning and resource management.
It allows us to:
-
Assess resource availability: Determining the quantity of biomass available in a given area is crucial for planning bioenergy projects and ensuring sustainable sourcing.
-
Optimize energy production: Knowing the energy content of different biomass types allows for efficient conversion and maximizing energy output.
-
Monitor environmental impact: Tracking biomass changes over time can provide insights into ecosystem health, carbon sequestration rates, and the impacts of land use change.
-
Inform policy decisions: Accurate biomass data is essential for developing effective policies related to renewable energy, forestry, agriculture, and waste management.
Without precise data, we risk overestimating the potential of biomass, leading to unsustainable practices and unintended consequences.
Biomass's Impact on Carbon Footprint Reduction
One of the most compelling arguments for biomass utilization is its potential to reduce our carbon footprint.
By replacing fossil fuels with biomass-derived energy, we can significantly decrease greenhouse gas emissions.
Furthermore, sustainable land management practices can enhance the carbon sequestration capacity of biomass resources, turning them into carbon sinks that actively remove CO2 from the atmosphere.
However, it is crucial to acknowledge that biomass is not a panacea. The carbon footprint of biomass energy depends on factors such as:
-
Sourcing practices: Unsustainable harvesting can negate the carbon benefits.
-
Transportation distances: Long transportation distances increase emissions.
-
Conversion technologies: Inefficient conversion processes can reduce the overall carbon savings.
Therefore, a holistic approach, guided by accurate biomass assessment, is essential for realizing the full carbon reduction potential of this valuable resource.
Biomass stands as a cornerstone of sustainable living and renewable energy strategies. It represents a vital link to a future powered by resources that replenish naturally, contrasting sharply with finite fossil fuels. Understanding what biomass is, and crucially, how much of it we have, is paramount. Accurate biomass calculation is essential for gauging the true potential of this renewable resource, and for its effective deployment across a spectrum of applications. From mitigating climate change to boosting energy efficiency, the responsible management of biomass, guided by precise data, offers a pathway toward a more sustainable world.
Expanding on this foundational understanding, let's delve deeper into the nature of biomass itself, exploring its origins, diverse forms, and how it can be harnessed to create energy. Understanding the nuances of biomass is critical to realizing its full potential.
Understanding Biomass and Biomass Energy: A Deeper Dive
Defining Biomass: A Closer Look
Biomass, at its core, is organic matter derived from living or recently deceased organisms. This definition is intentionally broad, encompassing an enormous range of materials. Understanding this breadth is key to appreciating the versatility of biomass as a resource.
Unlike fossil fuels that require millions of years to form, biomass is constantly being replenished through natural biological processes. This inherent renewability makes it a vital component of sustainable energy strategies.
The biological origins of biomass are diverse. It can originate from plant life, animal waste, or even microorganisms. Each source presents unique characteristics and challenges for energy production.
Biomass Energy: Concept and Application
Biomass energy refers to the energy harnessed from biomass through various conversion processes.
This energy can be used for a wide range of applications, including electricity generation, heating, and transportation fuels.
The concept is simple: biomass is converted into a usable energy form, such as heat, electricity, or liquid fuel, providing a renewable alternative to fossil fuels.
This conversion can be achieved through methods like:
- Combustion (burning biomass to produce heat).
- Gasification (converting biomass into a combustible gas).
- Pyrolysis (heating biomass in the absence of oxygen to produce liquid fuels and biochar).
- Anaerobic digestion (using microorganisms to break down biomass in the absence of oxygen to produce biogas).
The application of biomass energy is expanding, with ongoing research and development efforts focused on improving efficiency and reducing environmental impact.
Classifying Biomass Types
Biomass can be classified into several key categories, each with its own characteristics and applications:
-
Agricultural Residues: These include crop stalks, leaves, and other leftover plant material from harvesting. Examples include corn stover, wheat straw, and rice husks. They are abundant and often underutilized resources.
-
Wood and Woody Biomass: This encompasses trees, shrubs, and forestry residues. Examples include logging debris, wood chips, and sawdust. Sustainable forestry practices are crucial for ensuring the long-term availability of this resource.
-
Dedicated Energy Crops: These are plants specifically grown for energy production. Examples include switchgrass, miscanthus, and energy cane. They are selected for their high yields and suitability for conversion into biofuels.
-
Algae and Aquatic Plants: Biomass sourced from marine and freshwater environments. Examples include macroalgae (seaweed) and microalgae. They offer a high potential for biofuel production and wastewater treatment.
-
Organic Waste: Including municipal solid waste, animal waste, and food processing byproducts. Examples include sewage sludge, manure, and food scraps. Using organic waste for energy production can reduce landfill waste and generate renewable energy.
Advantages and Disadvantages of Different Biomass Types
Each type of biomass presents its own set of advantages and disadvantages for energy production:
Agricultural Residues
- Advantages: Abundant, readily available, and can reduce waste.
- Disadvantages: Seasonal availability, potential for soil depletion if not managed properly, and transportation costs.
Wood and Woody Biomass
- Advantages: Relatively high energy content, established infrastructure for harvesting and processing.
- Disadvantages: Deforestation concerns if not sustainably managed, air pollution from combustion, and competition with other wood product industries.
Dedicated Energy Crops
- Advantages: High yields, can be grown on marginal lands, and potential for carbon sequestration.
- Disadvantages: Land use competition with food crops, fertilizer requirements, and water consumption.
Algae and Aquatic Plants
- Advantages: High growth rates, can be grown in wastewater, and potential for producing a variety of biofuels.
- Disadvantages: Harvesting and processing challenges, nutrient requirements, and potential for environmental impacts.
Organic Waste
- Advantages: Reduces landfill waste, generates renewable energy, and avoids methane emissions from decomposition.
- Disadvantages: Variable composition, potential for contamination, and requires specialized processing equipment.
Carefully considering these advantages and disadvantages is crucial for selecting the most appropriate biomass type for a specific energy production application. Ultimately, the best choice depends on local resources, environmental considerations, and economic factors.
Biomass, in its myriad forms, stands as a powerful tool for renewable energy and sustainable practices. But to truly unlock its potential, we need to accurately assess its quantity and quality. This requires a move beyond simply knowing what biomass is; it demands a practical understanding of how to measure and calculate it effectively.
Calculating Biomass: A Step-by-Step Guide to Key Methods
Calculating biomass is not a one-size-fits-all endeavor. It requires understanding various methodologies, each with its own strengths and limitations. The selection of an appropriate method depends on factors like the type of biomass, the scale of the assessment, and the available resources.
Methods for Calculating Biomass
Several methods are employed to estimate biomass. These techniques vary in complexity, cost, and accuracy.
Direct measurement involves physically sampling and analyzing the biomass. Remote sensing, on the other hand, utilizes technologies like satellite imagery and LiDAR to estimate biomass over large areas. Modeling approaches use mathematical equations and computer simulations to predict biomass based on environmental factors.
Choosing the right approach is crucial for obtaining reliable data.
The Significance of Net Primary Productivity (NPP)
Net Primary Productivity (NPP) is a fundamental concept in biomass calculation. It represents the rate at which an ecosystem accumulates biomass.
NPP is essentially the difference between the rate of carbon gained through photosynthesis and the rate of carbon lost through respiration. A higher NPP indicates a more productive ecosystem, capable of generating more biomass. Understanding NPP is crucial for assessing the potential of a given area to provide sustainable biomass resources.
Dry Weight and Moisture Content: Essential Parameters
Dry weight and moisture content are critical parameters in biomass calculation. Moisture content significantly affects the energy content and handling characteristics of biomass. Dry weight provides a standardized measure of biomass, removing the variability caused by water content.
Accurate measurement of these parameters is essential for determining the true biomass yield.
Measuring Dry Weight and Moisture Content Accurately
Dry weight is typically determined by oven-drying a sample until it reaches a constant weight. The difference between the fresh weight and the dry weight represents the moisture content.
Proper sampling techniques and calibrated equipment are vital for obtaining accurate results. Standardized protocols, such as those established by organizations like ASTM International, should be followed.
Direct Measurement Techniques in Agriculture and Forestry
Direct measurement techniques are commonly used in agricultural and forestry settings to assess biomass yield. In agriculture, this may involve harvesting and weighing crops from a defined area.
In forestry, tree measurements such as diameter at breast height (DBH) and tree height are used to estimate biomass using allometric equations. These equations relate tree dimensions to biomass based on empirical data.
These direct methods provide valuable data for optimizing management practices.
Biomass Energy Conversion: The Role of Combustion and Pyrolysis
Biomass can be converted into energy through various processes. Combustion involves burning biomass to produce heat, which can then be used to generate electricity. Pyrolysis involves heating biomass in the absence of oxygen to produce bio-oil, biochar, and syngas.
Understanding the principles of combustion and pyrolysis is crucial for optimizing biomass energy conversion processes. Factors such as temperature, pressure, and residence time play critical roles in determining the efficiency and product yield of these processes.
Biomass assessments give us a deeper insight into the health and productivity of our ecosystems. Armed with the foundational understanding of biomass calculation methods and the concept of Net Primary Productivity (NPP), we can now focus on specific areas where these calculations have a tangible impact. Let's examine the pivotal role biomass calculation plays in agriculture, and how this data fosters more sustainable and efficient farming practices.
Biomass Calculation in Agriculture: Fostering Sustainable Practices
Biomass calculation is not just an academic exercise; it's a practical tool that can revolutionize agricultural practices. By accurately measuring and monitoring biomass, farmers and agricultural scientists can gain valuable insights into crop health, soil fertility, and the overall efficiency of their operations. This information can then be used to make informed decisions that promote sustainability, reduce environmental impact, and improve crop yields.
The Foundation of Sustainable Agriculture
Sustainable agriculture aims to produce food and fiber in a way that minimizes environmental damage, supports economic viability, and promotes social equity. Biomass calculations play a crucial role in achieving these goals by providing a quantitative basis for evaluating different farming practices.
-
For instance, knowing the biomass yield of a particular crop helps farmers assess the effectiveness of different fertilization strategies.
-
It informs decisions about irrigation and pest control, and ultimately contributes to more efficient resource use.
-
In essence, biomass calculation is a foundational element of any data-driven approach to sustainable agriculture.
Optimizing Crop Rotation and Soil Management
One of the most significant applications of biomass data is in optimizing crop rotation and soil management strategies. Different crops have different impacts on soil health, and biomass calculations can help farmers understand these impacts more clearly.
-
For example, legumes are known for their ability to fix nitrogen in the soil, which can improve soil fertility and reduce the need for synthetic fertilizers.
-
By measuring the biomass of legume crops, farmers can quantify the amount of nitrogen they are adding to the soil and adjust their fertilization practices accordingly.
-
Similarly, biomass data can be used to assess the effectiveness of different soil management practices, such as cover cropping and no-till farming.
-
Cover crops, which are planted to protect and improve the soil between cash crops, can increase soil organic matter and reduce erosion.
-
Biomass calculations can help farmers determine the optimal mix of cover crops for their specific soil conditions and crop rotation systems.
-
No-till farming, which involves planting crops without plowing the soil, can also improve soil health and reduce erosion.
-
Biomass data can be used to monitor the long-term effects of no-till farming on soil carbon sequestration and overall soil productivity.
-
Reducing Fertilizer Use and Environmental Impact
Excessive fertilizer use is a major environmental problem, contributing to water pollution, greenhouse gas emissions, and soil degradation. Biomass calculations can help farmers reduce their reliance on synthetic fertilizers by providing a more precise understanding of crop nutrient requirements.
-
By measuring the biomass of crops at different growth stages, farmers can determine how much nutrient uptake is occurring and adjust their fertilization practices accordingly.
-
This approach, known as precision fertilization, can significantly reduce fertilizer use without sacrificing crop yields.
-
Moreover, biomass calculations can also help farmers optimize the use of organic fertilizers, such as manure and compost.
-
These organic amendments can improve soil health and reduce the need for synthetic fertilizers, but they must be applied carefully to avoid nutrient imbalances.
-
Biomass data can help farmers determine the appropriate application rates for organic fertilizers, ensuring that crops receive the nutrients they need without causing environmental harm.
-
Biomass calculation serves as a vital tool for fostering sustainability within agricultural practices. By helping to optimize crop management, improve soil health, and reduce environmental impact, this method is crucial for creating a more resilient and environmentally conscious food system.
Biomass calculation, as we've seen, is vital in agriculture. It helps with everything from fertilizer decisions to improving irrigation. Shifting our focus from the farm to the forest, we'll explore how biomass data is just as crucial for the long-term health of our woodlands.
Managing Forestry Resources: Leveraging Biomass Data for Effective Conservation
Forests are more than just collections of trees; they are complex ecosystems. They play a critical role in carbon sequestration, water regulation, and biodiversity conservation. Effective forest management relies on a deep understanding of these intricate systems. This is where biomass calculations become indispensable.
Biomass data provides essential insights into the health and productivity of forests. It allows forest managers to make informed decisions. These decisions impact everything from timber harvesting to conservation efforts. Let's explore how this data is used to ensure the sustainable management of our vital forestry resources.
How Biomass Data Drives Effective Forest Management
Biomass data is the cornerstone of responsible forestry practices. It provides a quantifiable measure of the resources present. This allows managers to make informed decisions about harvesting, replanting, and conservation strategies.
Understanding forest biomass helps to:
- Estimate carbon stocks and sequestration rates, which are critical for climate change mitigation.
- Assess the impact of disturbances like fires, pests, and diseases.
- Determine the sustainable harvesting levels that maintain forest health and productivity.
- Monitor forest growth and development over time, informing long-term management plans.
Sustainable Forestry Practices and Biomass Information
Sustainable forestry aims to meet the needs of the present without compromising the ability of future generations. Biomass information is essential for achieving this balance. It provides the data needed to implement practices that protect forest health. It also supports biodiversity and ensures the long-term productivity of timber resources.
Specifically, biomass data informs decisions related to:
- Reforestation: Determining the optimal species composition and planting density to maximize biomass production and biodiversity.
- Thinning: Identifying and removing trees to reduce competition and promote the growth of remaining trees, thereby enhancing overall forest health and timber quality.
- Prescribed Burning: Using controlled burns to reduce fuel loads, prevent wildfires, and promote the regeneration of fire-adapted species.
Preventing Deforestation and Promoting Biodiversity Through Biomass Assessment
Deforestation is a major threat to global ecosystems and biodiversity. Biomass assessment plays a critical role in combating this threat. It provides data for sustainable forest management practices.
By monitoring biomass changes over time, we can detect illegal logging and other activities that contribute to deforestation. Biomass assessments also help to identify areas that are particularly vulnerable to deforestation. This allows for targeted conservation efforts.
Furthermore, biomass data is essential for promoting biodiversity. Different forest types and management practices support different species. By understanding how biomass is distributed across the landscape, managers can create habitats. These habitats support a diverse range of plant and animal life.
Managing forestry resources through biomass data analysis enables us to take effective conservation measures in our natural forests.
However, the role of biomass extends far beyond the forest and agricultural landscape. Its potential within waste management and recycling is significant, offering a pathway to transform waste into valuable resources.
Biomass's Role in Waste Management and Recycling: Turning Waste into Resource
The growing global waste crisis demands innovative solutions. Biomass offers a compelling avenue to mitigate the environmental impact of waste while simultaneously generating valuable resources.
Biomass plays a crucial role in waste management by converting organic waste materials into usable products, contributing to a more sustainable and circular economy.
Biomass Applications in Waste Management
Biomass applications in waste management are diverse.
They encompass various methods of converting waste into energy and other valuable products.
Anaerobic digestion, for instance, uses microorganisms to break down organic waste in the absence of oxygen. This process produces biogas, a renewable energy source.
Composting transforms organic waste into a nutrient-rich soil amendment, reducing the need for chemical fertilizers.
Incineration with energy recovery burns waste at high temperatures. This generates heat that can be used to produce electricity.
These methods provide viable alternatives to traditional landfill disposal.
Biomass Benefits in Recycling Processes
The integration of biomass into recycling processes offers numerous advantages. It enhances the efficiency and sustainability of waste treatment.
One key benefit is the reduction of reliance on virgin materials. By converting waste into usable resources, biomass helps conserve natural resources and reduces the environmental impact associated with extraction and processing.
For example, paper waste can be processed into new paper products.
This reduces the demand for wood pulp derived from trees.
Biomass can also be used to create bioplastics. These are biodegradable alternatives to conventional plastics derived from fossil fuels.
Reducing Landfill Waste and Promoting a Circular Economy
The implementation of biomass technologies in waste management is essential for reducing landfill waste. It fosters a circular economy.
Landfills pose significant environmental risks. They can contaminate soil and groundwater. Also, they release harmful greenhouse gasses like methane.
By diverting organic waste from landfills and converting it into valuable resources, biomass reduces these risks and promotes a more sustainable waste management system.
This approach aligns with the principles of a circular economy. It minimizes waste generation, maximizes resource utilization, and promotes the reuse and recycling of materials.
A well-managed biomass waste system supports environmental protection, resource conservation, and economic opportunity.
However, the role of biomass extends far beyond the forest and agricultural landscape. Its potential within waste management and recycling is significant, offering a pathway to transform waste into valuable resources. Now, shifting our focus from waste management, let’s explore how biomass calculation plays a vital role in reducing our carbon footprint and fostering environmental sustainability.
Reducing Your Carbon Footprint: The Link Between Biomass Calculation and Environmental Impact
Accurate biomass assessment is more than just a scientific exercise; it's a crucial tool in our fight against climate change. By understanding the carbon dynamics associated with biomass, we can make informed decisions that minimize our environmental impact and promote a more sustainable future.
The Role of Accurate Biomass Assessment
The precision of biomass calculation directly impacts our ability to quantify and reduce carbon emissions.
Inaccurate estimations can lead to flawed carbon accounting, resulting in ineffective climate mitigation strategies.
For instance, underestimating biomass stocks in forests could lead to overestimation of carbon sequestration potential, while overestimating it could result in unsustainable harvesting practices.
Therefore, reliable biomass data is essential for developing realistic and achievable carbon reduction targets.
Biomass Utilization and Positive Environmental Impact
Utilizing biomass as a renewable energy source offers numerous environmental benefits.
When sourced sustainably, biomass can significantly reduce our reliance on fossil fuels, a major contributor to greenhouse gas emissions.
Burning biomass releases carbon dioxide, but this carbon is part of the natural carbon cycle, as it was originally absorbed from the atmosphere during plant growth.
This contrasts with fossil fuels, which release carbon that has been stored underground for millions of years, thus disrupting the carbon balance.
Furthermore, biomass can be used to produce biofuels, bioplastics, and other bio-based products, reducing our dependence on petroleum-based materials and their associated environmental impacts.
Biomass's Contribution to Climate Change Mitigation
Biomass plays a multifaceted role in mitigating climate change.
It acts as a carbon sink, absorbing carbon dioxide from the atmosphere and storing it in plant tissues.
Sustainable forest management practices, such as afforestation and reforestation, can enhance this carbon sequestration capacity, offsetting carbon emissions from other sectors.
Biomass energy can displace fossil fuels, reducing greenhouse gas emissions from electricity generation, transportation, and industrial processes.
Additionally, biochar, a byproduct of biomass pyrolysis, can be used as a soil amendment to improve soil fertility and sequester carbon in the soil for long periods.
The Importance of Sustainable Practices
It's crucial to emphasize that the climate benefits of biomass are contingent on sustainable practices.
Unsustainable harvesting of forests or agricultural residues can lead to deforestation, soil degradation, and increased carbon emissions.
Therefore, biomass utilization should always be guided by principles of sustainability, ensuring that it contributes to long-term environmental health and climate stability.
In conclusion, accurate biomass calculation is fundamental to understanding and mitigating our carbon footprint. By embracing sustainable biomass practices, we can harness its potential to reduce greenhouse gas emissions, promote environmental sustainability, and combat climate change effectively.
Real-World Success: Case Studies of Biomass Calculation in Action
The theoretical underpinnings of biomass calculation are essential, but their true value lies in practical application. Examining real-world case studies allows us to understand the tangible benefits, the inevitable challenges, and the valuable lessons learned when implementing biomass calculation methodologies in agriculture and forestry. These examples showcase the transformative potential of accurate biomass assessment.
Biomass Calculation in Agriculture: Optimizing Yield and Sustainability
One compelling case study involves a large-scale agricultural project focused on optimizing corn yield while minimizing environmental impact. The project utilized precise biomass calculation to determine the optimal fertilizer application rates for different corn varieties.
Enhanced Fertilizer Efficiency and Reduced Runoff
By accurately assessing the biomass of the corn plants at various growth stages, the project team was able to tailor fertilizer application to meet the plant's specific needs. This resulted in significant reductions in fertilizer use, leading to cost savings for the farmers and a decreased risk of nutrient runoff into nearby waterways.
The data collected through biomass calculation also informed irrigation strategies, minimizing water waste and further reducing the environmental footprint of the agricultural operation. This project demonstrated that accurate biomass assessment is not just an academic exercise, but a powerful tool for enhancing agricultural efficiency and sustainability.
Forestry Management: Preserving Carbon Sinks and Biodiversity
In the realm of forestry, biomass calculation plays a critical role in sustainable resource management and conservation efforts. A case study in the Pacific Northwest highlights the use of biomass data in managing a large tract of old-growth forest.
Balancing Timber Harvesting with Ecosystem Health
The forestry management team utilized a combination of remote sensing data and ground-based measurements to estimate the total biomass of the forest. This information was crucial in developing a sustainable harvesting plan that balanced timber production with the need to maintain the forest's carbon sequestration capacity and biodiversity.
By carefully selecting trees for harvesting based on their biomass and growth rate, the team was able to minimize the impact on the overall forest ecosystem. The biomass data also informed reforestation efforts, ensuring that harvested areas were replanted with appropriate tree species to promote long-term carbon storage.
Overcoming Challenges: Data Integration and Scalability
The implementation of biomass calculation methodologies is not without its challenges. In the forestry case study, integrating data from multiple sources (remote sensing, ground surveys, and historical records) proved to be a complex task.
The team invested significant effort in developing robust data processing and analysis workflows to ensure data quality and consistency. Another challenge was scaling up the biomass assessment techniques to cover a large and heterogeneous forest area.
This required the use of sophisticated statistical modeling and spatial analysis techniques to extrapolate biomass estimates from sample plots to the entire forest.
Key Lessons Learned from Implementation
The success of these and other biomass calculation projects underscores the importance of several key factors:
- Accurate Data Collection: Reliable biomass data is essential for generating meaningful insights and making informed decisions.
- Integration of Multiple Data Sources: Combining remote sensing, ground-based measurements, and other data sources can improve the accuracy and robustness of biomass assessments.
- Collaboration and Knowledge Sharing: Effective communication and collaboration between scientists, resource managers, and policymakers are crucial for successful implementation.
By embracing these principles, we can unlock the full potential of biomass calculation to promote sustainable practices and build a more resilient future.
Looking Ahead: Future Trends and Technologies in Biomass Calculation
The field of biomass calculation is not static; it's a dynamic area driven by innovation and the urgent need for more precise and efficient assessment methods. As we strive for greater sustainability and a deeper understanding of our ecosystems, the tools and techniques we use to measure biomass are constantly evolving. Let's explore the exciting future trends and technologies poised to revolutionize this critical field.
Emerging Technologies: Revolutionizing Biomass Assessment
Several cutting-edge technologies are poised to transform how we measure and analyze biomass. These advancements promise to enhance accuracy, reduce costs, and provide more comprehensive insights into biomass dynamics.
Remote sensing has already made significant strides, but future iterations will offer even greater resolution and analytical capabilities. Drones equipped with hyperspectral cameras and LiDAR (Light Detection and Ranging) technology can capture detailed data on vegetation structure and composition over large areas.
This data can then be used to create accurate biomass maps and models, providing valuable information for resource management and conservation efforts.
Artificial intelligence (AI) and machine learning algorithms are also playing an increasingly important role. These powerful tools can analyze complex datasets from various sources to identify patterns and predict biomass changes over time.
AI can be used to automate the process of biomass estimation, reducing the need for manual measurements and improving efficiency. Furthermore, AI can help us to refine existing biomass models and develop new ones that are more accurate and robust.
Advancements in Data Analysis and Modeling
The sheer volume of data generated by modern biomass assessment techniques requires sophisticated analytical tools and models. Advancements in these areas are crucial for extracting meaningful insights and informing effective decision-making.
Improved statistical modeling techniques are enabling us to better account for uncertainties and biases in biomass estimates. These models can also incorporate data from multiple sources, such as remote sensing, field measurements, and climate data, to provide a more holistic picture of biomass dynamics.
Big data analytics are also becoming increasingly important. By leveraging the power of cloud computing and advanced algorithms, we can process and analyze vast amounts of biomass data in real-time.
This allows us to detect trends, identify anomalies, and make informed decisions about resource management. For example, big data analytics can be used to track deforestation rates, monitor the impact of climate change on forest biomass, and optimize harvesting strategies.
The Role of Geographic Information Systems (GIS)
Geographic Information Systems (GIS) are powerful tools for visualizing, analyzing, and managing spatial data. In the context of biomass calculation, GIS can be used to integrate data from various sources, such as remote sensing, field surveys, and climate models, to create comprehensive biomass maps and models.
GIS enables us to understand the spatial distribution of biomass, identify areas of high and low biomass density, and assess the impact of environmental factors on biomass productivity.
Furthermore, GIS can be used to support decision-making in a variety of applications, such as forest management, agricultural planning, and conservation efforts.
For example, GIS can be used to identify areas that are suitable for biomass energy production, assess the risk of wildfires, and design effective conservation strategies. The integration of GIS with other technologies, such as remote sensing and AI, is opening up new possibilities for biomass assessment and management.
Video: Biomass Calculation: Easy Guide for Sustainable Living
FAQs: Biomass Calculation for Sustainable Living
This FAQ section addresses common questions related to understanding and applying biomass calculation for sustainable living practices. We aim to provide clarity and practical guidance.
Why is calculating biomass important for sustainability?
Calculating biomass helps us understand the quantity of renewable organic matter available. This is crucial for assessing the potential of biomass energy sources, monitoring ecosystem health, and making informed decisions about sustainable resource management. Knowing how to calculate biomass enables better resource allocation.
What are the primary methods used to calculate biomass?
Common methods include direct harvesting and weighing, allometric equations that relate biomass to easily measured tree dimensions (like diameter), and remote sensing techniques. The most appropriate method depends on the scale of the assessment and the resources available. Learning how to calculate biomass using different methods allows for flexibility.
How accurate is biomass calculation, and what factors affect it?
Accuracy varies based on the method used. Direct harvesting is most accurate but destructive. Allometric equations rely on established relationships, which may not be universally applicable. Factors affecting accuracy include species variation, environmental conditions, and measurement errors. Proper technique when learning how to calculate biomass improves result reliability.
Can biomass calculation be used for waste management?
Yes, understanding how to calculate biomass is very important. Biomass calculation can assess the amount of organic waste (e.g., food waste, agricultural residues) available for composting, anaerobic digestion, or biofuel production. This helps in optimizing waste management strategies and reducing landfill waste. It helps determine if a waste-to-energy project is viable.