NaBr Ionic Compound: Unlocking Its Secrets!
Sodium bromide, or NaBr ionic compound, plays a critical role in various chemical applications, extending from its use as a sedative to its application in photography. Understanding the structure of NaBr ionic compound reveals a crystalline lattice, a property extensively studied at institutions like the National Institute of Standards and Technology (NIST). The ionic bond's strength within this NaBr ionic compound directly influences its solubility, analyzed using techniques like X-ray diffraction, commonly employed by researchers globally. Furthermore, its behavior in aqueous solutions impacts osmosis, a fundamental process in biological systems where the NaBr ionic compound can act as a solute.
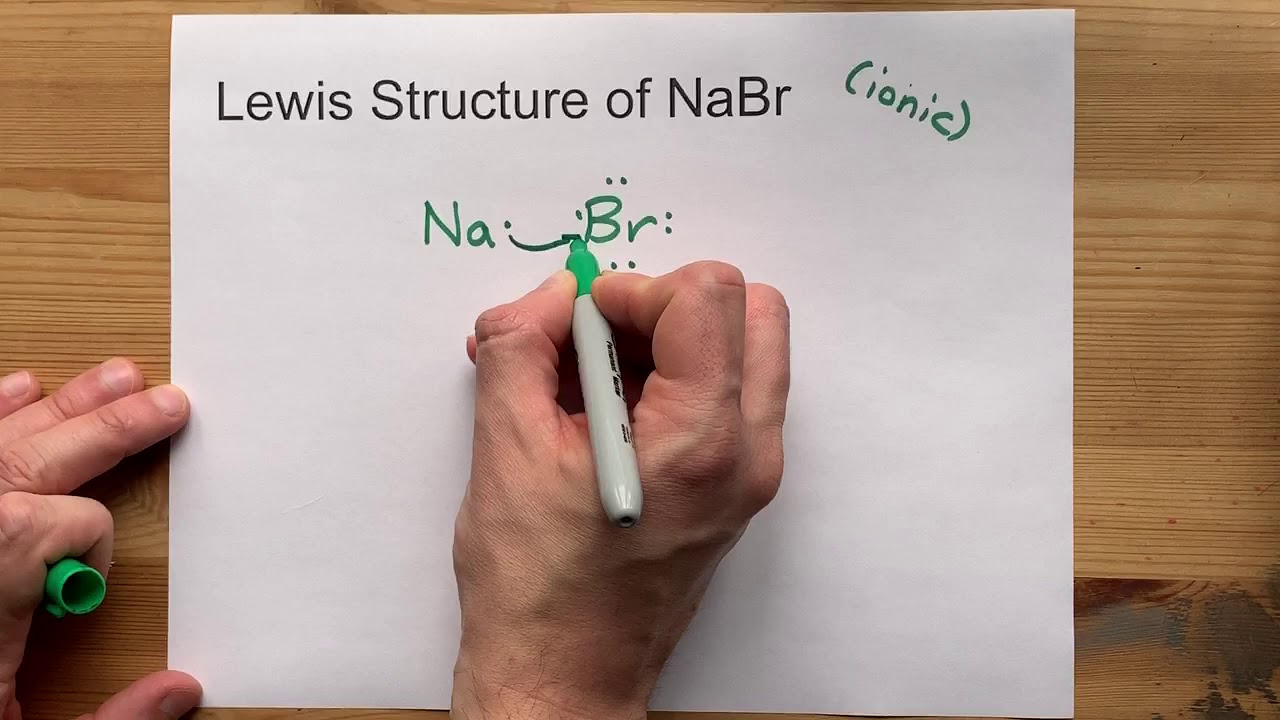
Image taken from the YouTube channel chemistNATE , from the video titled Draw the Lewis Structure of NaBr (sodium bromide) .
Sodium Bromide (NaBr), a compound often encountered yet perhaps not fully appreciated, stands as a critical player in various scientific and industrial arenas. This seemingly simple ionic compound unlocks a wealth of fascinating chemistry and practical applications.
This article embarks on a comprehensive exploration of Sodium Bromide. We aim to dissect its fundamental properties, unravel its intricate structure, and illuminate its diverse applications that span from the realm of medicine to the intricacies of industrial processes.
What Makes NaBr Significant?
NaBr's significance stems from its unique properties as an ionic compound. Ionic compounds, formed through the electrostatic attraction between positively and negatively charged ions, exhibit characteristic behaviors that distinguish them from other classes of chemical substances.
Sodium Bromide, in particular, showcases the archetypal characteristics of ionic compounds: high melting and boiling points, excellent solubility in polar solvents, and the capacity to conduct electricity when dissolved in water.
The Importance of Understanding Ionic Bonds
The properties of NaBr are inextricably linked to the nature of its ionic bond. Understanding the principles governing ionic bonding is therefore essential to grasp the behavior of NaBr.
Ionic bonds arise from the transfer of electrons between atoms with significantly different electronegativities. In the case of Sodium Bromide, sodium (Na) readily donates an electron to bromine (Br), resulting in the formation of positively charged sodium ions (Na+) and negatively charged bromide ions (Br-).
The electrostatic attraction between these oppositely charged ions constitutes the ionic bond, holding the compound together in a stable, crystalline lattice. The strength of this bond dictates many of NaBr's physical and chemical properties, including its high melting point and its ability to dissociate into ions when dissolved in water, rendering it a strong electrolyte.
This examination will not only deepen your understanding of this specific compound but also enrich your appreciation for the broader implications of ionic bonding in the world of chemistry.
The strength of the ionic bond, as we’ve seen, explains many of Sodium Bromide’s observable characteristics. But before we delve further into its specific properties, let's examine how this compound comes to be. Understanding its chemical formula and formation is crucial to appreciating its behavior.
Chemical Formula and Formation: The Building Blocks of NaBr
The chemical formula NaBr represents Sodium Bromide, indicating that the compound is composed of an equal number of sodium (Na) and bromine (Br) atoms. This simple notation encapsulates a fundamental relationship: for every sodium ion present, there exists one bromide ion, ensuring electrical neutrality within the compound.
The Genesis of NaBr: From Elements to Compound
Sodium Bromide doesn't just appear spontaneously; it is formed through a chemical reaction between its constituent elements: sodium (Na), a soft, silvery-white metal, and bromine (Br), a reddish-brown liquid.
The reaction is highly exothermic, releasing a significant amount of energy in the form of heat and light.
This vigorous reaction is a direct consequence of the drive towards achieving a stable electron configuration, a core principle of chemical bonding.
The Electron Transfer: The Key to Ionic Bonding
At the heart of NaBr formation lies the transfer of electrons. Sodium, with one valence electron, readily donates this electron to bromine, which needs only one electron to complete its octet.
This transfer results in the formation of a positively charged sodium ion (Na+) and a negatively charged bromide ion (Br-).
The electrostatic attraction between these oppositely charged ions constitutes the ionic bond, the "glue" that holds the NaBr compound together.
Achieving Stability: The Octet Rule and Noble Gas Configuration
The driving force behind the electron transfer is the octet rule, which states that atoms tend to gain, lose, or share electrons to achieve a full complement of eight valence electrons, mirroring the stable electron configuration of noble gases.
Sodium, by losing one electron, attains the same electron configuration as neon (Ne), while bromine, by gaining one electron, achieves the same electron configuration as krypton (Kr).
This newfound stability is the energetic justification for the formation of the ionic bond and the creation of the NaBr compound.
The electrostatic attraction between these oppositely charged ions constitutes the ionic bond, the very essence of NaBr's existence. But what exactly makes this bond so strong, and how does it dictate the properties of the compound?
Ionic Bonding in NaBr: The Force That Holds It Together
The formation of Sodium Bromide isn't just about atoms coming together; it's about a fundamental restructuring of electron configurations to achieve stability. The ionic bond that emerges is the glue that holds NaBr together.
The Dance of Electrons: Na+ and Br-
Sodium (Na) possesses a single valence electron, a lonely outlier in its outer shell. Bromine (Br), on the other hand, is just one electron shy of a complete octet, a state of blissful electronic equilibrium.
This sets the stage for an elegant electron transfer. Sodium eagerly donates its valence electron to bromine.
This seemingly simple act transforms both atoms into ions: Na becomes Na+, a positively charged cation, and Br becomes Br-, a negatively charged anion.
The Electrostatic Embrace: Formation of the Ionic Bond
The magic truly happens once the ions are formed. Opposites attract, as the saying goes, and the positively charged Na+ and negatively charged Br- are no exception.
The strong electrostatic force between these oppositely charged ions is the ionic bond. This is the fundamental force that binds the ions together in a crystal lattice.
It's this powerful attraction that defines many of NaBr's key characteristics.
Stability Achieved: The Enduring Nature of NaBr
The formation of the ionic bond in NaBr isn't just a fleeting interaction; it's a pathway to enhanced stability.
By achieving full outer electron shells, both sodium and bromine ions reach a lower energy state than their neutral atomic counterparts.
This stability is reflected in NaBr's relatively high melting and boiling points, properties that indicate the substantial energy required to disrupt the ionic bonds holding the compound together.
Quantifying the Bond: Lattice Energy
The strength of the ionic bond in NaBr can be quantified using a concept called lattice energy. Lattice energy is defined as the energy required to completely separate one mole of a solid ionic compound into its gaseous ions.
A high lattice energy indicates a strong ionic bond, reflecting the intense electrostatic attraction between the ions.
Factors influencing lattice energy include the charge of the ions and the distance between them. Higher charges and smaller distances generally lead to stronger ionic bonds and higher lattice energies.
The formation of the ionic bond in NaBr is, in essence, the foundational step, but it doesn't paint the complete picture. These positively and negatively charged ions don't just float around independently; they arrange themselves in a very specific, highly ordered structure that dictates the macroscopic properties we observe.
Crystal Structure of NaBr: A Lattice of Ions
Sodium bromide, like many other ionic compounds, doesn't exist as individual molecules in the solid-state. Instead, it assembles into a highly ordered, three-dimensional array known as a crystal lattice.
The Cubic Lattice Arrangement
The crystal structure of NaBr is typically a cubic lattice, specifically a face-centered cubic (FCC) arrangement, similar to that of sodium chloride (NaCl).
This arrangement minimizes the energy of the system. It does so by maximizing the attractive forces between oppositely charged ions while minimizing the repulsive forces between ions of the same charge.
Ionic Arrangement Within the Lattice
In the NaBr crystal lattice, each Na+ ion is surrounded by six Br- ions, and conversely, each Br- ion is surrounded by six Na+ ions.
This alternating arrangement extends throughout the entire crystal, creating a vast network of interconnected ions.
The specific distances between ions are determined by the ionic radii of Na+ and Br-, as well as the balance between attractive and repulsive forces.
Stability and Properties
This highly ordered crystal structure contributes significantly to the stability and physical properties of NaBr.
-
High Melting Point: The strong electrostatic forces holding the ions together require a substantial amount of energy to overcome, resulting in a relatively high melting point.
-
Brittleness: While the ionic bonds themselves are strong, the crystal structure is susceptible to fracture. When stress is applied, ions can shift position, bringing ions of like charge into close proximity. The resulting repulsion can lead to cleavage along specific crystal planes.
-
Solubility in Polar Solvents: The polar nature of water molecules allows them to effectively solvate the Na+ and Br- ions, disrupting the crystal lattice and leading to dissolution.
Understanding the crystal structure of NaBr provides valuable insights into its macroscopic properties and behavior. The orderly arrangement of ions within the lattice is not merely a structural detail; it is fundamental to understanding the compound's inherent characteristics.
The alternating arrangement of sodium and bromide ions within the crystal lattice dictates many of NaBr's physical characteristics. However, to truly understand how NaBr participates in chemical reactions, we need to consider a fundamental property: its molar mass. This value serves as a crucial bridge between the macroscopic world of grams and the microscopic realm of atoms and molecules.
Molar Mass: The Keystone of Stoichiometry for NaBr
Molar mass is a fundamental concept in chemistry, representing the mass of one mole of a substance. It is expressed in grams per mole (g/mol) and serves as the bridge between mass and the number of particles (atoms, molecules, ions) in a sample.
For any compound, the molar mass is calculated by summing the atomic masses of all the atoms in its chemical formula. Let's delve into the specifics for NaBr.
Calculating the Molar Mass of NaBr
To determine the molar mass of NaBr, we need the atomic masses of sodium (Na) and bromine (Br) from the periodic table.
- Atomic mass of Na ≈ 22.99 g/mol
- Atomic mass of Br ≈ 79.90 g/mol
Since NaBr contains one sodium atom and one bromine atom, the molar mass of NaBr is:
Molar mass of NaBr = Atomic mass of Na + Atomic mass of Br
Molar mass of NaBr = 22.99 g/mol + 79.90 g/mol
Molar mass of NaBr ≈ 102.89 g/mol
This means that one mole of NaBr weighs approximately 102.89 grams.
Significance in Stoichiometric Calculations
The molar mass of NaBr is indispensable in stoichiometric calculations. Stoichiometry is the branch of chemistry that deals with the quantitative relationships between reactants and products in chemical reactions.
Essentially, molar mass allows us to convert between grams (a readily measurable quantity) and moles (a unit that directly relates to the number of molecules or ions).
Consider a scenario where you need to prepare a solution with a specific concentration of NaBr.
You would need to calculate the mass of NaBr required to dissolve in a given volume of solvent. This calculation directly relies on the molar mass of NaBr.
Example: To prepare 1 liter of a 1.0 M (molar) solution of NaBr, you would need 1 mole of NaBr. Using the molar mass, you can easily determine that you need 102.89 grams of NaBr.
Molar Mass and Chemical Reactions Involving NaBr
In chemical reactions, the molar mass of NaBr is crucial for determining the amount of NaBr involved as a reactant or produced as a product. Balanced chemical equations provide the mole ratios of reactants and products.
By using the molar mass, we can convert these mole ratios into mass ratios, enabling us to predict the mass of NaBr required for a reaction or the mass of NaBr that will be produced.
For instance, if NaBr is involved in a precipitation reaction, knowing its molar mass allows us to calculate the theoretical yield of NaBr precipitate based on the amount of reactants used. This is vital in industrial settings and research laboratories, where precise control over reaction outcomes is essential.
Molar mass allows us to move from the weight of a sample to the number of molecules it contains, and vice versa. But beyond its mass, how does NaBr respond to changes in temperature? The answer lies in its melting and boiling points, thermal properties that reveal much about the strength of its internal structure.
Melting and Boiling Points: Thermal Properties of NaBr
The melting point and boiling point of a substance are critical indicators of its thermal stability and the strength of intermolecular forces holding it together. For sodium bromide (NaBr), a high-melting and boiling point are characteristic features, reflecting the robust nature of its ionic bonds.
Defining Melting and Boiling Points
The melting point is the temperature at which a solid transitions into a liquid state.
The boiling point is the temperature at which a liquid transforms into a gaseous state.
These transitions require energy to overcome the attractive forces between the constituent particles.
The Melting Point of NaBr
Sodium bromide has a high melting point of approximately 747°C (1377°F).
This elevated temperature indicates that a significant amount of energy is needed to disrupt the ionic lattice structure and allow the ions to move freely in the liquid phase.
The strong electrostatic attraction between the positively charged sodium ions (Na+) and negatively charged bromide ions (Br-) is responsible for this high energy requirement.
The Boiling Point of NaBr
NaBr also boasts a high boiling point, around 1390°C (2534°F).
This extremely high value signifies that even more energy is required to completely separate the ions from each other, allowing them to exist as a gas.
At the boiling point, the ions must overcome not only the electrostatic forces but also the forces that keep them in relatively close proximity in the liquid phase.
Ionic Bond Strength and Thermal Properties
The high melting and boiling points of NaBr are directly related to the strength of its ionic bonds.
Ionic bonds are formed through the electrostatic attraction between oppositely charged ions.
This attraction is considerably stronger than the intermolecular forces found in many covalent compounds.
Consequently, more thermal energy is needed to overcome these forces and induce phase transitions.
The magnitude of the charge on the ions and the distance between them influence the strength of the ionic bond.
Smaller interionic distances and higher charges lead to stronger bonds and, therefore, higher melting and boiling points.
Energy Required for Phase Transitions
The energy required to change the phase of a substance without changing its temperature is known as latent heat.
The latent heat of fusion is the energy needed to melt a solid, while the latent heat of vaporization is the energy needed to boil a liquid.
For NaBr, both the latent heat of fusion and the latent heat of vaporization are substantial, reflecting the high energy input necessary to overcome the strong ionic bonds.
This can be quantified using calorimetry, which measures the heat absorbed or released during a phase transition.
The large amounts of energy involved are a testament to the stability of the NaBr crystal lattice.
Solubility: How NaBr Interacts with Solvents
As we've established, NaBr's high melting and boiling points indicate strong ionic bonds. But what happens when NaBr is introduced to different environments? Its behavior in various solvents, particularly water, is a key property that determines its applications and interactions with other substances. This section explores the solubility of sodium bromide, the factors influencing it, and the molecular dynamics of its dissolution.
NaBr Solubility in Water
Sodium bromide is highly soluble in water, meaning a significant amount of NaBr can dissolve in a given quantity of water.
This high solubility is primarily due to water’s polarity and its ability to effectively solvate ions.
Water molecules are polar, possessing a slightly negative charge on the oxygen atom and slightly positive charges on the hydrogen atoms.
These partial charges allow water molecules to interact strongly with both the positively charged sodium ions (Na+) and the negatively charged bromide ions (Br-).
The Dissolution Process at a Molecular Level
When NaBr is added to water, the polar water molecules surround the ions on the surface of the crystal lattice.
This process, called hydration, involves electrostatic interactions between the water molecules and the ions.
The negatively charged oxygen atoms of water are attracted to the Na+ ions, while the positively charged hydrogen atoms are attracted to the Br- ions.
These interactions weaken the ionic bonds within the NaBr crystal, eventually leading to the separation of the ions from the lattice.
Once separated, the ions are surrounded by a shell of water molecules, effectively dispersing them throughout the solution.
This dispersion of ions is what defines the dissolution of NaBr in water, forming a homogeneous solution.
Solubility in Other Solvents
While NaBr is highly soluble in water, its solubility in other solvents varies significantly depending on the solvent's polarity.
Polar solvents, like water, tend to dissolve ionic compounds like NaBr better than nonpolar solvents.
This is because polar solvents can effectively solvate the ions, as described above.
In nonpolar solvents, such as hexane or benzene, NaBr is practically insoluble.
Nonpolar solvents lack the partial charges needed to interact strongly with the ions, so they cannot effectively overcome the ionic bonds holding the crystal lattice together.
As a result, NaBr remains undissolved in these solvents.
Factors Affecting Solubility
Several factors can influence the solubility of NaBr in a given solvent. These include:
-
Temperature: Generally, the solubility of NaBr in water increases with temperature. As the temperature rises, the kinetic energy of the water molecules increases, allowing them to more effectively break the ionic bonds in the crystal lattice. This leads to a higher concentration of dissolved NaBr in the solution.
-
Pressure: Pressure has a negligible effect on the solubility of solid solutes like NaBr in liquid solvents.
-
Presence of Other Solutes: The presence of other dissolved salts in the solution can affect the solubility of NaBr. This is known as the common ion effect. If the solution already contains a high concentration of either sodium or bromide ions, the solubility of NaBr will decrease. This is due to the equilibrium shifting to reduce the concentration of the added common ion.
With a grasp on how readily NaBr dissolves, it’s natural to wonder about its inherent compactness. Just how much "stuff" is packed into a given space when we're dealing with sodium bromide? The answer lies in its density, a fundamental property that provides valuable insights into its structure and behavior.
Density: Measuring the Compactness of NaBr
Density is a crucial physical property that describes how much mass is contained within a given volume. For sodium bromide (NaBr), understanding its density provides insight into the arrangement of its atoms and ions within its crystal lattice.
Density Value at Room Temperature
The density of NaBr at room temperature (approximately 25°C) is approximately 3.21 g/cm³.
This means that one cubic centimeter of NaBr has a mass of 3.21 grams. It's a characteristic value, useful for identification, purity checks, and calculations involving volume and mass conversions.
Significance of Density
Density is more than just a number; it's a window into the internal structure of a compound.
A higher density generally indicates that the atoms or ions are packed more closely together. Conversely, a lower density suggests a more open or less compact structure.
Density and Crystal Structure
The density of NaBr is directly related to its crystal structure, which, as we've discussed, is a cubic lattice.
In this lattice, sodium ions (Na+) and bromide ions (Br-) are arranged in an alternating pattern, maximizing the electrostatic attractions between oppositely charged ions.
The specific arrangement and the ionic radii of Na+ and Br- dictate how closely these ions can pack together.
Since bromine is a relatively large atom, the bromide ions significantly contribute to the overall volume of the unit cell.
The efficient packing of these ions within the cubic lattice results in the characteristic density of NaBr.
Factors Influencing Density
While the inherent crystal structure is the primary determinant of density, external factors can also have a minor influence.
Temperature, for instance, can cause slight changes in density due to thermal expansion.
As temperature increases, the ions vibrate more vigorously, leading to a slight increase in the average distance between them and a corresponding decrease in density.
Pressure can also affect density, but the effect is generally small for solids like NaBr unless extremely high pressures are applied.
Applications of Density Information
Knowing the density of NaBr is important for several reasons.
In the laboratory, it allows for the conversion between mass and volume, which is crucial for preparing solutions of specific concentrations.
In industrial settings, density measurements can be used to assess the purity of NaBr samples and to monitor the consistency of production processes.
Furthermore, in research, density data can be used to validate theoretical models of the crystal structure and to understand the effects of different factors on the packing of ions within the lattice.
By measuring the density of NaBr, we gain a tangible understanding of the relationship between its atomic composition, crystal structure, and macroscopic properties.
With a grasp on how readily NaBr dissolves, it’s natural to wonder about its inherent compactness. Just how much "stuff" is packed into a given space when we're dealing with sodium bromide? The answer lies in its density, a fundamental property that provides valuable insights into its structure and behavior. From this understanding of its physical presence, our investigation naturally leads us to consider how NaBr behaves when it's not a solid – specifically, its behavior in solution and its capacity to conduct electricity.
Electrolyte Behavior: NaBr in Solution
Sodium bromide (NaBr) isn't just a compound with a specific density and crystal structure; it's also a strong electrolyte.
This means that when NaBr is dissolved in water, it undergoes a dramatic transformation, separating into its constituent ions: sodium cations (Na+) and bromide anions (Br-).
This dissociation process is the key to understanding its electrical conductivity and its role in various chemical and biological systems.
Why NaBr is a Strong Electrolyte
The classification of NaBr as a strong electrolyte stems from its near-complete dissociation in aqueous solutions.
Unlike weak electrolytes, which only partially ionize, NaBr breaks down almost entirely into its ions when dissolved in water.
This behavior is primarily due to the nature of the ionic bond between sodium and bromide ions.
The electronegativity difference between sodium and bromine is significant, leading to a highly polar bond.
When water molecules surround the NaBr crystal, their polar nature helps to overcome the electrostatic forces holding the Na+ and Br- ions together.
The positively charged hydrogen atoms in water molecules are attracted to the negatively charged bromide ions.
Simultaneously, the negatively charged oxygen atoms in water are attracted to the positively charged sodium ions.
This solvation process effectively shields the ions from each other, reducing the attractive forces and facilitating their separation into the solution.
Dissociation into Ions in Aqueous Solution
The dissociation of NaBr in water can be represented by the following equation:
NaBr (s) → Na+ (aq) + Br- (aq)
Here, (s) denotes the solid state, and (aq) indicates that the ions are dissolved in water (aqueous solution).
This equation illustrates that one mole of solid NaBr produces one mole of sodium ions and one mole of bromide ions when dissolved in water.
The resulting solution contains freely moving charged particles (ions), which are capable of conducting electricity.
Conductivity of NaBr Solutions
The ability of an NaBr solution to conduct electricity is directly proportional to the concentration of ions present.
Since NaBr is a strong electrolyte and dissociates almost completely, even relatively dilute solutions contain a significant number of ions.
This results in a high electrical conductivity compared to solutions of weak electrolytes or non-electrolytes.
The movement of ions in response to an applied electric field is what allows the solution to conduct electricity.
The positive sodium ions migrate towards the negatively charged electrode (cathode), while the negative bromide ions migrate towards the positively charged electrode (anode).
This flow of charge constitutes an electric current, demonstrating the electrolyte behavior of NaBr in solution.
With a grasp on how readily NaBr dissolves, it’s natural to wonder about its inherent compactness. Just how much "stuff" is packed into a given space when we're dealing with sodium bromide? The answer lies in its density, a fundamental property that provides valuable insights into its structure and behavior. From this understanding of its physical presence, our investigation naturally leads us to consider how NaBr behaves when it's not a solid – specifically, its behavior in solution and its capacity to conduct electricity. But beyond its electrical properties, sodium bromide also participates in a range of chemical reactions, making it a versatile reagent in various applications. Let's delve into the chemical reactivity of NaBr and examine some of the common reactions it undergoes.
Chemical Properties: Unpacking the Reactivity of NaBr
Sodium bromide (NaBr), at its core, behaves as a typical ionic compound. This means its reactivity is largely governed by the behavior of its constituent ions: the sodium cation (Na+) and the bromide anion (Br-). While Na+ is generally less reactive, the Br- ion plays a significant role in many chemical reactions.
Understanding NaBr's Reactive Nature
NaBr's reactivity primarily stems from its ionic nature and the stability of the individual ions it forms. The compound itself is stable under normal conditions, but its ions can participate in a variety of reactions. These reactions include precipitation reactions, displacement reactions, and redox reactions, where the bromide ion acts as a reducing agent.
Precipitation Reactions: Forming Insoluble Salts
One of the most common reactions involving NaBr is its participation in precipitation reactions. These reactions occur when NaBr is mixed with a solution containing metal cations that form insoluble bromides.
For instance, when NaBr is mixed with a solution of silver nitrate (AgNO3), a white precipitate of silver bromide (AgBr) forms. The net ionic equation for this reaction is:
Ag+(aq) + Br-(aq) → AgBr(s)
This reaction is a classic example of a precipitation reaction, driven by the low solubility of silver bromide in water. The formation of the solid precipitate removes the silver and bromide ions from the solution, effectively driving the reaction to completion.
Displacement Reactions: Halide Exchange
NaBr can also participate in displacement reactions, where the bromide ion is replaced by another halide ion. This type of reaction typically occurs with more reactive halogens, such as chlorine or fluorine.
For example, if chlorine gas (Cl2) is bubbled through a solution of NaBr, the following reaction occurs:
Cl2(g) + 2NaBr(aq) → 2NaCl(aq) + Br2(l)
In this reaction, chlorine displaces bromine from the NaBr, forming sodium chloride (NaCl) and elemental bromine (Br2). This reaction demonstrates the higher reactivity of chlorine compared to bromine, as chlorine is more electronegative and has a greater tendency to gain electrons.
Redox Reactions: Bromide as a Reducing Agent
The bromide ion in NaBr can act as a reducing agent, meaning it can donate electrons to other substances. However, bromine is a relatively weak reducing agent compared to other halides like iodide. Therefore, NaBr is not commonly used as a strong reducing agent in chemical reactions.
However, under specific conditions, such as in the presence of a strong oxidizing agent, the bromide ion can be oxidized to elemental bromine. For example, the reaction with concentrated sulfuric acid (H2SO4) can lead to the formation of bromine gas.
Reaction with Acids: Formation of Hydrogen Bromide
When NaBr reacts with strong acids, such as sulfuric acid (H2SO4) or phosphoric acid (H3PO4), it can produce hydrogen bromide (HBr) gas.
The reaction with sulfuric acid is:
2NaBr(s) + H2SO4(l) → Na2SO4(s) + 2HBr(g)
Hydrogen bromide is a strong acid and a useful reagent in various chemical syntheses. However, it's important to note that concentrated sulfuric acid can also oxidize HBr to bromine, as mentioned in the previous section. Therefore, using phosphoric acid is often preferred for generating pure HBr, as it is a weaker oxidizing agent.
With an understanding of NaBr's chemical reactivity, it's time to examine its sensory characteristics.
Physical Properties: Describing NaBr
Beyond its chemical interactions, sodium bromide possesses a distinct set of physical attributes that define its appearance and behavior under various conditions. These properties, observable through our senses or measurable with instruments, offer crucial clues about its underlying structure and how it interacts with the world around it.
Appearance and Color
Sodium bromide, in its purest form, is a white crystalline solid.
The term "crystalline" indicates that the atoms and ions within NaBr are arranged in a highly ordered, repeating pattern.
This ordered structure contributes to its solid state at room temperature and its characteristic appearance.
While pure NaBr is white, slight impurities can sometimes impart a subtle off-white or grayish hue.
However, a distinct or strong coloration would generally suggest the presence of significant contaminants.
Crystalline Structure
As mentioned earlier, NaBr forms cubic crystals.
Each sodium ion (Na+) is surrounded by six bromide ions (Br-), and vice versa, in a three-dimensional lattice.
This arrangement maximizes the electrostatic attraction between the oppositely charged ions, contributing to the stability of the crystal structure.
The crystalline structure not only dictates its appearance but also influences other physical properties like its hardness and cleavage.
Taste and Odor
NaBr has a strong saline (salty) taste.
However, tasting NaBr is generally not recommended due to potential health hazards and the availability of safer methods for identification.
In its pure form, sodium bromide is odorless.
Any detectable odor would likely indicate the presence of impurities or decomposition products.
Hygroscopicity
Sodium bromide is hygroscopic, meaning it readily absorbs moisture from the air.
This property is due to the attraction of water molecules to the polar Na+ and Br- ions on the surface of the crystal.
When exposed to humid air, NaBr can gradually absorb water and may even deliquesce, forming a solution.
Therefore, NaBr should be stored in a tightly sealed container to prevent moisture absorption.
With an understanding of NaBr's chemical reactivity, it's time to examine its sensory characteristics. Now, transitioning from the observable properties of sodium bromide, let's delve into how this compound is actually created in the lab. The synthesis of NaBr involves specific chemical reactions and carefully controlled conditions.
Preparation of NaBr: Synthesizing the Compound
Sodium bromide (NaBr), while readily available commercially, can also be synthesized in the laboratory. Several methods exist for its preparation. These methods rely on well-established chemical reactions. Each approach offers unique advantages and considerations.
Neutralization of Hydrobromic Acid with a Base
One of the most common methods for synthesizing NaBr involves a neutralization reaction. This process entails reacting hydrobromic acid (HBr) with a suitable base, such as sodium hydroxide (NaOH) or sodium carbonate (Na2CO3).
Reaction with Sodium Hydroxide
The reaction between HBr and NaOH is a simple acid-base neutralization. The reaction proceeds as follows:
HBr(aq) + NaOH(aq) → NaBr(aq) + H2O(l)
This reaction is highly exothermic. It releases significant heat. Therefore, it's crucial to perform it slowly and with adequate cooling to prevent splattering or boiling.
The resulting solution contains NaBr and water. To obtain solid NaBr, the water needs to be evaporated. This can be done using a rotary evaporator or by gently heating the solution.
After evaporation, the solid NaBr can be further purified by recrystallization. This involves dissolving the crude NaBr in a minimal amount of hot water. The solution is then cooled to allow pure NaBr crystals to form.
Reaction with Sodium Carbonate
Another approach involves using sodium carbonate (Na2CO3) as the base. The reaction proceeds as follows:
2HBr(aq) + Na2CO3(aq) → 2NaBr(aq) + H2O(l) + CO2(g)
In this reaction, carbon dioxide gas (CO2) is evolved. This provides a visual indication that the reaction is occurring.
Similar to the NaOH method, the resulting solution is evaporated. The solid NaBr is then purified by recrystallization.
Direct Reaction of Sodium with Bromine
NaBr can also be synthesized by the direct reaction of sodium metal with liquid bromine.
2Na(s) + Br2(l) → 2NaBr(s)
This reaction is highly vigorous and exothermic. It requires careful handling due to the reactivity of both sodium metal and bromine. This method is generally not suitable for small-scale laboratory preparations due to the hazards involved.
Safety Considerations
When preparing NaBr, several safety precautions are essential. Hydrobromic acid is a corrosive substance. Appropriate personal protective equipment (PPE), including gloves, safety goggles, and a lab coat, must be worn.
Reactions involving strong acids and bases should be performed in a well-ventilated area. This is to avoid inhaling any fumes.
The direct reaction of sodium with bromine should only be performed by experienced chemists with proper training and equipment. Bromine is a toxic and corrosive substance. Sodium metal reacts violently with water and air.
With an understanding of NaBr's chemical reactivity, it's time to examine its sensory characteristics. Now, transitioning from the observable properties of sodium bromide, let's delve into how this compound is actually created in the lab. The synthesis of NaBr involves specific chemical reactions and carefully controlled conditions.
Applications in Medicine: Historical Uses of NaBr
Sodium bromide, or NaBr, has a noteworthy history in the field of medicine, primarily as a sedative and hypnotic. While its use has largely been superseded by newer medications, understanding its historical role provides valuable context in the evolution of pharmacological treatments for anxiety and sleep disorders.
NaBr as a Sedative and Hypnotic
From the late 19th century through the mid-20th century, sodium bromide was a widely prescribed sedative. It was used to treat a variety of conditions, including:
- Nervousness
- Anxiety
- Insomnia
- Epilepsy
Its effectiveness in these areas stemmed from its ability to depress the central nervous system, inducing a calming effect and promoting sleep.
Historical Context and Widespread Use
The popularity of NaBr as a sedative can be attributed to several factors:
- Ready availability
- Relative affordability
- Perceived safety compared to alternatives like barbiturates
It was even available over-the-counter in many countries, contributing to its widespread use and, unfortunately, occasional misuse.
Mechanism of Action
The sedative effects of NaBr are believed to be related to the bromide ion's influence on neuronal excitability. Bromide ions can interfere with chloride ion transport in neurons, hyperpolarizing the cell membrane.
This makes it more difficult for neurons to fire, leading to a decrease in overall nervous system activity. The result is a calming or sedative effect.
Drawbacks and Gradual Replacement
Despite its widespread use, NaBr had significant drawbacks:
- Relatively slow onset of action
- Potential for accumulation in the body
- Risk of bromism, a toxic condition caused by chronic bromide exposure
Symptoms of bromism include:
- Neurological disturbances
- Skin rashes
- Gastrointestinal issues
Due to these concerns and the development of safer and more effective alternatives (like benzodiazepines), NaBr gradually fell out of favor. Its use as a general sedative significantly declined by the mid-20th century.
Modern Applications in Veterinary Medicine
While largely replaced in human medicine, NaBr still finds limited use in veterinary medicine. It is used as an antiepileptic drug in dogs to manage seizures.
The longer half-life of bromide in dogs, compared to humans, makes it a practical option for controlling epileptic episodes. However, even in veterinary applications, careful monitoring is necessary to avoid bromism.
Legacy and Lessons Learned
The history of NaBr serves as a valuable case study in the evolution of medical treatments. It highlights the importance of:
- Careful assessment of drug safety
- Understanding potential side effects
- Continuous development of safer and more effective medications
Although NaBr is no longer a mainstay in human medicine, its legacy reminds us of the ongoing quest to improve patient care through scientific advancement.
With a grasp of NaBr’s medicinal history established, its journey doesn't end in the pharmacy. Sodium bromide's unique chemical characteristics make it valuable far beyond healthcare, finding applications in diverse industrial processes.
Industrial Applications: Beyond Medicine
Sodium bromide's versatility extends far beyond its historical uses in medicine, making it a crucial component in various industrial processes. These applications leverage NaBr's chemical properties, demonstrating its importance in different sectors, from photography to oil and gas extraction.
NaBr in Photography
Historically, sodium bromide played a significant role in the photographic industry. Specifically, it was used in the production of light-sensitive silver bromide (AgBr), a key ingredient in photographic film.
The process involves reacting NaBr with silver nitrate (AgNO3), resulting in the precipitation of AgBr.
AgNO3(aq) + NaBr(aq) → AgBr(s) + NaNO3(aq)
The silver bromide crystals are then suspended in gelatin to form the emulsion coated on photographic film.
When exposed to light, AgBr undergoes a photochemical reaction, forming a latent image that can be developed into a visible picture.
While digital photography has reduced the demand for silver-based films, AgBr remains essential in specialized photographic applications and certain types of scientific imaging.
Sodium Bromide in Oil and Gas Industry
NaBr is extensively employed in the oil and gas industry, particularly in well completion and workover fluids. These fluids are essential for maintaining wellbore stability, minimizing formation damage, and facilitating efficient drilling operations.
NaBr solutions are used due to their high density, which helps to counterbalance the pressure exerted by the surrounding geological formations. This prevents the well from collapsing or experiencing uncontrolled fluid influx.
Compared to other dense brines, NaBr offers advantages such as:
- Lower corrosivity to equipment.
- Compatibility with various drilling fluids.
- Relatively low toxicity.
The use of NaBr brines is particularly important in high-pressure, high-temperature (HPHT) wells where maintaining wellbore stability is critical.
Other Industrial Uses of NaBr
Beyond photography and the oil & gas industry, NaBr finds uses in:
-
Chemical Synthesis: As a source of bromide ions in various chemical reactions, facilitating the production of other bromine-containing compounds.
-
Water Treatment: As an algicide and disinfectant in certain water treatment applications.
-
Textile Industry: As a component in some dyeing processes.
These varied applications underscore the value of NaBr as an industrially significant chemical compound. Its unique properties make it suitable for many specialized tasks across diverse sectors.
With its applications in photography and oil and gas well maintenance clearly defined, it becomes apparent that sodium bromide is more than just a chemical curiosity. Its range of uses extends into several surprising domains, further highlighting its adaptability.
Other Applications of NaBr
While NaBr's presence in medicine (historically) and key industries like photography and oil drilling is well-established, its versatility doesn't end there. Sodium bromide finds uses in diverse niche applications, solidifying its place as a valuable compound in various fields.
Analytical Chemistry
NaBr serves as a reagent in certain analytical chemistry procedures.
It can be used in qualitative tests to identify specific ions or compounds through precipitation reactions. The formation of characteristic precipitates can indicate the presence of certain substances in a sample.
Furthermore, NaBr can be used as a supporting electrolyte in electrochemical experiments, providing a conductive medium for redox reactions.
As a Source of Bromide Ions
NaBr is a convenient and relatively safe source of bromide ions.
In chemical synthesis, bromide ions are essential building blocks for creating various bromine-containing organic and inorganic compounds.
NaBr can be used to introduce bromine into molecules, leading to the formation of new chemical entities with unique properties.
This makes it valuable in research and development for synthesizing new pharmaceuticals, agrochemicals, or specialty materials.
Use in Hair Care Products
Sodium Bromide can be found in some hair care products.
It can be an ingredient in some hair straightening or perming formulas. In these applications, it functions as a reducing agent, aiding in breaking and reforming the disulfide bonds in hair keratin. This allows for altering the hair's natural structure.
However, its use in such products requires careful formulation and adherence to safety guidelines due to its potential irritant properties.
Fire Retardant
NaBr can be used as a fire retardant in specific applications.
When combined with other fire-retardant materials, it can enhance their effectiveness in suppressing flames.
Bromide ions released during combustion can interfere with the chain reactions that sustain fire.
This application is less common than other bromine-containing fire retardants, but it still holds relevance in niche industries where specific fire safety requirements exist.
These additional applications of NaBr, while perhaps less widely known than its role in photography or medicine, showcase the breadth of its utility. From analytical chemistry to specialized chemical synthesis, sodium bromide continues to be a valuable tool in a variety of scientific and industrial endeavors, demonstrating its adaptable nature.
Video: NaBr Ionic Compound: Unlocking Its Secrets!
FAQs: Unlocking the Secrets of NaBr Ionic Compound
Here are some frequently asked questions to further clarify the properties and uses of Sodium Bromide (NaBr).
What exactly is an ionic compound, and how does NaBr fit that description?
Ionic compounds are formed through the electrostatic attraction between oppositely charged ions. NaBr ionic compound is a perfect example. Sodium (Na) readily loses an electron to become a positive ion (cation), while Bromine (Br) readily gains an electron to become a negative ion (anion). These oppositely charged ions strongly attract, forming the NaBr crystal lattice.
Why is NaBr soluble in water?
NaBr's solubility in water is due to the polar nature of water molecules. Water molecules surround the Na+ and Br- ions, effectively shielding them from each other and weakening the ionic bonds in the NaBr ionic compound. This allows the ions to disperse throughout the water, resulting in dissolution.
What are some common applications of NaBr?
NaBr ionic compound finds use in various applications. It is commonly used as a sedative and hypnotic. In the oil and gas industry, NaBr solutions are used as completion fluids. Additionally, NaBr is utilized in photographic processing.
Is NaBr toxic, and what precautions should be taken when handling it?
While NaBr ionic compound isn't acutely toxic in small amounts, excessive ingestion can lead to bromide poisoning. Symptoms can include nausea, vomiting, and neurological effects. It's always best to handle NaBr with care, avoiding ingestion or prolonged skin contact. Wear appropriate personal protective equipment like gloves when handling large quantities.
So, there you have it – a glimpse into the fascinating world of NaBr ionic compound! Hopefully, this article helped demystify some of its secrets. Go forth and explore!