Benzene Boiling Point: The Ultimate Guide You Need!
Benzene, a fundamental aromatic hydrocarbon, exhibits a characteristic benzene boiling point, a physical property critical for various chemical processes. The stability of its aromatic ring significantly impacts its vapor pressure, thus influencing its boiling point. Measuring the benzene boiling point accurately often involves techniques like distillation, a process refined by notable chemists like Friedrich Kekulé, whose work contributed to understanding benzene's structure. Understanding the benzene boiling point is crucial in industrial applications, particularly in settings such as petrochemical plants where benzene is utilized as a solvent and a chemical intermediate. This guide provides a comprehensive exploration of the benzene boiling point, detailing its underlying principles, factors that influence it, and its practical relevance.
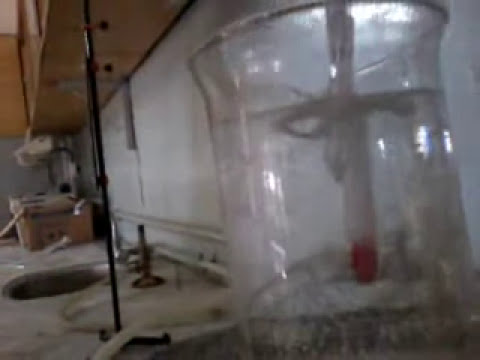
Image taken from the YouTube channel HAI-Tech , from the video titled Boiling Point of an Organic Compound | Boiling Point of Benzene | Chemistry Experiments .
Benzene, a seemingly simple cyclic hydrocarbon, holds a pivotal position in the world of chemistry and industry. Its unique structure and properties make it a fundamental building block for a vast array of chemical products, from pharmaceuticals and polymers to dyes and detergents. However, to effectively utilize benzene, a thorough understanding of its physical characteristics, particularly its boiling point, is essential.
This article serves as a comprehensive guide, dissecting the intricacies surrounding benzene's boiling point. We will explore the fundamental principles governing this crucial physical property, examining the interplay of molecular forces and thermodynamic principles.
Benzene: A Chemical Cornerstone
Benzene (C6H6) is an aromatic hydrocarbon characterized by its cyclic structure with alternating single and double bonds. This unique arrangement gives rise to its exceptional stability and reactivity, making it a versatile component in numerous chemical processes.
Its applications are far-reaching, impacting industries ranging from pharmaceuticals to plastics manufacturing. Benzene is a precursor to many important compounds, highlighting its indispensable role in modern chemistry.
Purpose and Scope
The primary goal of this article is to provide a clear and detailed explanation of benzene's boiling point. We aim to demystify the underlying science and explore the practical implications of this property.
We will cover the following key areas:
- Defining the Concept: Establishing a clear understanding of what boiling point signifies.
- Benzene's Properties: Examining the molecular structure and intermolecular forces that influence its boiling point.
- Factors Affecting Boiling Point: Investigating the impact of pressure, temperature, and vapor pressure.
- Thermodynamic Principles: Delving into the heat of vaporization and the Clausius-Clapeyron equation.
- Practical Applications: Exploring the real-world significance of benzene's boiling point across various industries.
By addressing these aspects, this article aims to equip readers with a comprehensive understanding of benzene's boiling point and its relevance in both theoretical and applied contexts.
Through this exploration, we hope to illuminate not only the specific characteristics of benzene, but also the broader principles that govern the behavior of chemical compounds.
Defining Boiling Point: A Phase Transition Explained
Before delving into the specific characteristics of benzene's boiling point, it's essential to establish a clear understanding of what boiling point signifies in general terms. This fundamental concept underpins all discussions related to phase transitions and the behavior of matter under varying conditions.
What is Boiling Point? A Definition
Boiling point is defined as the temperature at which the vapor pressure of a liquid equals the surrounding pressure and the liquid rapidly transforms into a gas. At this specific temperature, bubbles of vapor form within the liquid and rise to the surface, indicating a significant change in the substance's physical state.
The boiling point is not a fixed value for a substance; it is dependent on the external pressure. This relationship is critical for understanding how substances behave in different environments.
The Liquid-to-Gas Phase Transition
Boiling represents a specific type of phase transition, namely the change from a liquid state to a gaseous state. This transition requires energy, typically in the form of heat, to overcome the intermolecular forces holding the liquid together.
As heat is added, the molecules gain kinetic energy, moving faster and further apart. At the boiling point, the energy is sufficient to break these intermolecular bonds, allowing the molecules to escape into the gaseous phase. This transformation is a physical change, not a chemical reaction, meaning the substance's chemical identity remains unchanged. Only its physical state has changed.
The Role of Temperature in Reaching the Boiling Point
Temperature is the driving force behind the boiling process. As the temperature of a liquid increases, the kinetic energy of its molecules also increases.
This increased energy leads to more frequent and forceful collisions between molecules, allowing them to overcome intermolecular attractions more easily.
When the temperature reaches the boiling point, the molecules possess enough energy to escape the liquid phase entirely, resulting in the formation of vapor. The boiling point is therefore a direct measure of the energy required to overcome these intermolecular forces.
Pressure's Influence on the Boiling Point
Pressure plays a crucial role in determining the boiling point of a substance. Increased pressure requires more energy to overcome, therefore raising the boiling point. Conversely, lower pressure makes it easier for molecules to escape the liquid phase, reducing the boiling point.
This relationship is why water boils at a lower temperature at high altitudes, where the atmospheric pressure is lower. A pressure cooker, on the other hand, increases the pressure, raising the boiling point of water and allowing food to cook faster.
The relationship between pressure and boiling point is described by the Clausius-Clapeyron equation, which will be discussed later in the article.
Benzene Unveiled: Structure, Properties, and Significance
Having established the fundamental principles of boiling points and phase transitions, it's time to turn our attention to benzene itself. Understanding benzene's unique molecular structure and inherent properties is crucial to grasping why it boils at a particular temperature and how it behaves under various conditions. Let's delve into the core aspects that dictate benzene's physical behavior.
The Aromatic Ring: Benzene's Defining Feature
Benzene, with the chemical formula C6H6, possesses a distinct cyclic structure.
Six carbon atoms are arranged in a planar ring, with each carbon atom bonded to one hydrogen atom.
The key characteristic of benzene is its aromaticity. This stems from the delocalization of electrons within the ring, forming a continuous pi electron cloud above and below the plane of the ring.
This electron delocalization results in exceptional stability and gives benzene its characteristic chemical properties.
The bond lengths between the carbon atoms are all identical, intermediate between a single and a double bond, further demonstrating the delocalization of electrons.
Molecular Weight and Boiling Point Correlation
Molecular weight plays a crucial role in determining a substance's boiling point.
Generally, substances with higher molecular weights tend to have higher boiling points.
This is because larger molecules have more electrons, leading to stronger intermolecular forces.
Benzene has a molecular weight of approximately 78.11 g/mol. This value is significant because it influences the strength of the intermolecular forces between benzene molecules, ultimately affecting the energy required to overcome these forces and transition into the gaseous phase.
Intermolecular Forces: The Glue That Holds Benzene Together
Intermolecular forces are the attractive forces between molecules. These forces influence physical properties like boiling point, melting point, and viscosity.
Benzene primarily experiences London Dispersion Forces (LDFs), a type of Van der Waals force.
London Dispersion Forces Explained
LDFs arise from temporary fluctuations in electron distribution, creating instantaneous dipoles.
These temporary dipoles induce dipoles in neighboring molecules, leading to a weak attraction.
The strength of LDFs increases with the size and shape of the molecule. Benzene's symmetrical structure and relatively large size contribute to significant LDFs.
Even though LDFs are individually weak, the cumulative effect across numerous benzene molecules is substantial, influencing its boiling point and other physical properties.
The presence of these intermolecular forces dictates how much energy is required to separate benzene molecules from their liquid state into a gaseous state. Therefore, understanding their nature and magnitude is fundamental to understanding the boiling point of benzene.
Having explored benzene’s unique structure and the influence of molecular weight on its properties, we can now pinpoint the actual boiling point and the key factors that dictate this crucial physical characteristic. Understanding these variables allows us to predict and control benzene's behavior in various applications.
The Boiling Point of Benzene: A Deep Dive
The boiling point of benzene, a foundational piece of information for chemists and engineers alike, is specifically 80.1 degrees Celsius (80.1 °C) or 353.2 Kelvin (353.2 K), under standard atmospheric pressure (1 atm).
This value represents the temperature at which the vapor pressure of benzene equals the surrounding atmospheric pressure, allowing the liquid to transition into a gaseous state.
Factors Influencing Benzene's Boiling Point
Several factors can influence this benchmark figure, most notably pressure and temperature.
These elements operate in tandem to determine whether benzene exists in its liquid or gaseous phase.
The Influence of Pressure
Pressure is a primary determinant of a substance's boiling point.
Lowering the external pressure on benzene will cause it to boil at a lower temperature.
Conversely, increasing the pressure requires a higher temperature to reach the boiling point.
This inverse relationship is crucial in industrial processes where benzene is used at varying pressures.
The Role of Temperature
Temperature, as the driving force behind phase transitions, directly impacts the kinetic energy of benzene molecules.
As temperature increases, the molecules gain energy, move more vigorously, and are more likely to overcome the intermolecular forces holding them in the liquid phase.
When the vapor pressure exerted by these energized molecules equals the external pressure, boiling occurs.
Vapor Pressure and its Relationship to Benzene's Boiling Point
Vapor pressure is the pressure exerted by a vapor in thermodynamic equilibrium with its condensed phases (solid or liquid) at a given temperature in a closed system.
The higher the temperature, the greater the vapor pressure.
When the vapor pressure of benzene reaches the external pressure, the liquid boils.
This principle underlines the very definition of boiling point: the temperature at which vapor pressure equals the surrounding pressure.
For benzene, understanding its vapor pressure at different temperatures is vital for applications such as distillation and chemical reactions.
It dictates the rate of evaporation and the concentration of benzene vapor in a given environment.
Having examined the impact of temperature on molecular kinetic energy and intermolecular forces, it's time to delve into the thermodynamic principles that underpin benzene's phase transition. These principles dictate the energy requirements and predict boiling point behavior under varying conditions, offering a more profound understanding of this critical property.
The Science Behind the Boiling Point: Thermodynamics in Action
The boiling point of benzene isn't merely a temperature; it represents a delicate balance of energy and intermolecular forces, governed by the laws of thermodynamics. To fully grasp this phenomenon, we must consider the heat of vaporization and the Clausius-Clapeyron equation, two fundamental concepts that explain and predict benzene's boiling behavior.
Heat of Vaporization: Quantifying the Energy Input
The heat of vaporization (ΔHvap) is the amount of energy, typically measured in Joules per mole (J/mol) or Kilojoules per mole (kJ/mol), required to transform one mole of a substance from its liquid phase to its gaseous phase at a constant temperature (its boiling point).
For benzene, this value is significant because it quantifies the energy needed to overcome the intermolecular forces (primarily London dispersion forces) holding the molecules together in the liquid state.
A relatively high heat of vaporization indicates that strong intermolecular forces exist. More energy is needed to break these bonds and allow the molecules to escape into the gaseous phase.
Therefore, benzene's specific heat of vaporization is a direct reflection of the strength of its intermolecular attractions.
Understanding the heat of vaporization is crucial for designing industrial processes involving benzene. Such as distillation or evaporation, since it dictates the energy input required to achieve the desired phase change.
The Clausius-Clapeyron Equation: Predicting Boiling Point Shifts
The Clausius-Clapeyron equation is a powerful thermodynamic relationship that describes the variance of vapor pressure with temperature. More importantly, it allows us to calculate the boiling point of a liquid at different pressures, provided we know the heat of vaporization and the boiling point at one specific pressure.
The equation is expressed as:
ln(P2/P1) = - (ΔHvap/R) * (1/T2 - 1/T1)
Where:
- P1 and P2 are the vapor pressures at temperatures T1 and T2, respectively.
- ΔHvap is the heat of vaporization.
- R is the ideal gas constant (8.314 J/(mol·K)).
- T1 and T2 are the absolute temperatures (in Kelvin).
Applying the Equation to Benzene
By knowing benzene's standard boiling point (80.1 °C or 353.2 K at 1 atm) and its heat of vaporization, we can use the Clausius-Clapeyron equation to predict its boiling point at different pressures.
For example, if we decrease the pressure, the equation allows us to calculate the new, lower boiling point. This is particularly useful in industrial settings where benzene might be used under vacuum conditions.
Limitations and Considerations
It's crucial to acknowledge that the Clausius-Clapeyron equation relies on certain assumptions, such as the ideal behavior of the gas phase and the constancy of the heat of vaporization over the temperature range considered.
In reality, ΔHvap can vary slightly with temperature, so the equation provides an approximation. However, for many practical applications, it offers a sufficiently accurate prediction of benzene's boiling point at varying pressures.
In conclusion, thermodynamics provides the framework for understanding and predicting benzene's boiling point behavior.
The heat of vaporization quantifies the energy required for the phase transition, while the Clausius-Clapeyron equation allows us to calculate boiling points under different pressure conditions. These principles are essential for chemical engineers, researchers, and anyone working with benzene in practical applications.
Having explored the thermodynamics that dictate benzene's phase transition, it becomes clear that this knowledge isn't merely academic. The precise control and manipulation of benzene's boiling point are essential in numerous industrial processes. Let's examine the practical implications and widespread uses that stem from a thorough understanding of this fundamental property.
Benzene's Boiling Point: Practical Applications and Industrial Importance
The boiling point of benzene, a seemingly simple characteristic, holds profound implications for a multitude of industrial processes and applications. Understanding and controlling this parameter is not just a matter of scientific curiosity; it's a cornerstone of efficiency, safety, and product quality across diverse sectors.
Crucial Role in Industrial Processes
The chemical industry relies heavily on precise temperature control for various operations, and benzene is no exception. Distillation, a fundamental separation technique, absolutely depends on the boiling point.
Distillation Processes
Distillation is used to separate benzene from other hydrocarbons.
Understanding its boiling point ensures that benzene can be effectively isolated from mixtures based on differences in volatility.
Without this knowledge, efficient separation is impossible, impacting the purity and yield of the desired product.
Reaction Control
Many chemical reactions that involve benzene are temperature-sensitive.
Maintaining benzene at or near its boiling point can optimize reaction rates and yields, while preventing unwanted side reactions or decomposition.
This precise control ensures product quality, reduces waste, and increases overall efficiency.
Solvent Applications
Benzene's solvent properties are closely tied to its boiling point.
The temperature at which benzene boils affects its ability to dissolve and extract various compounds.
This is critical in industries such as pharmaceuticals and petrochemicals, where benzene is often used as a solvent.
Common Uses Reliant on Benzene's Boiling Point
Beyond its role in industrial processes, benzene's boiling point is a key factor in several everyday applications. These include manufacturing of various important everyday use products.
Polymer Production
Benzene is a crucial precursor in the synthesis of various polymers, including polystyrene and nylon.
The polymerization process often involves heating benzene-derived monomers to specific temperatures, relying on the understanding of its boiling point to ensure the reaction proceeds correctly and the desired polymer is formed.
Production of Dyes and Pharmaceuticals
The production of numerous dyes and pharmaceuticals involve chemical reactions using benzene.
The desired temperatures for reactions can be determined or maintained when using benzene as a solvent by using its boiling point as a reference.
This guarantees the safety of the workers and quality of products.
Gasoline Additive
Although now less common due to health concerns, benzene was historically used as an additive to gasoline to increase octane rating and improve engine performance.
Its boiling point played a role in determining its volatility and its contribution to the overall fuel mixture.
In summary, benzene's boiling point is more than just a physical property; it is a key parameter that dictates its behavior in a variety of industrial and practical applications.
A thorough understanding of this property is essential for optimizing processes, ensuring product quality, and promoting safety in the chemical industry and beyond.
Video: Benzene Boiling Point: The Ultimate Guide You Need!
Frequently Asked Questions About Benzene Boiling Point
This FAQ addresses common questions regarding the boiling point of benzene and factors that influence it, building on the information presented in our guide.
What is the boiling point of benzene under standard conditions?
The boiling point of benzene under standard atmospheric pressure (1 atm or 760 mmHg) is approximately 80.1 degrees Celsius (176.2 degrees Fahrenheit). This is a fundamental physical property of benzene and is important for its identification and use.
What factors can affect the benzene boiling point?
Pressure is the most significant factor affecting the boiling point. Lower pressure reduces the boiling point, while higher pressure increases it. Impurities within the benzene sample can also slightly alter the benzene boiling point.
Why is understanding the benzene boiling point important?
Knowing the boiling point is crucial for various applications, including distillation, purification processes, and safe handling procedures. It helps to predict its behavior under different conditions and ensures proper temperature control during experiments or industrial processes involving benzene.
Is the benzene boiling point high or low compared to other similar organic solvents?
The benzene boiling point is considered moderately high compared to solvents like diethyl ether (34.6 °C) but lower than solvents like water (100°C) or toluene (110.6 °C). The strength of the intermolecular forces (primarily van der Waals forces) is a significant factor determining the benzene boiling point.