Archaebacteria vs Eubacteria: The Ultimate Summary!
The field of microbiology provides foundational insights into the structure and function of cellular life. Carl Woese's groundbreaking work in molecular phylogeny revolutionized our understanding of prokaryotes. This understanding led to the establishment of Archaea as a distinct domain separate from Bacteria, necessitating a comprehensive archaebaceria and eubacteria summary. The phylogenetic tree of life accurately represents the evolutionary relationships between these domains. Understanding the differences between Archaea and Bacteria is crucial for fields like biotechnology and understanding extremophiles.
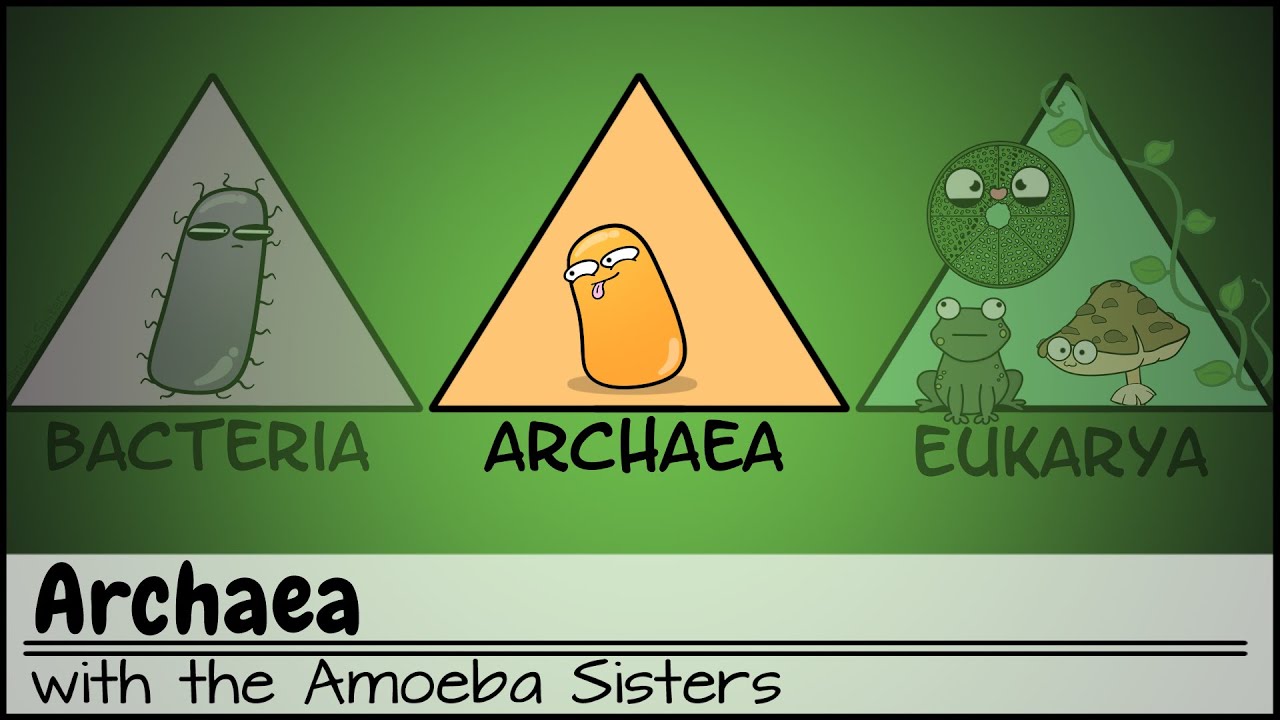
Image taken from the YouTube channel Amoeba Sisters , from the video titled Archaea .
Life on Earth exhibits a breathtaking spectrum of forms, ranging from the macroscopic wonders of towering redwoods and majestic whales to the microscopic marvels that teem in every drop of water and handful of soil.
Among these less visible life forms, the microbial world reigns supreme in both its sheer abundance and its staggering diversity. Understanding this microbial realm is not merely an academic exercise; it is crucial for comprehending the very fabric of our planet's ecosystems, the intricacies of human health, and the potential for groundbreaking biotechnological advancements.
The Prokaryotic Divide: Archaea and Bacteria
Within the vast microbial landscape, two prominent groups stand out: Archaea and Bacteria. These two domains represent fundamental divisions within the prokaryotic world. Prokaryotes are single-celled organisms that lack a nucleus and other complex membrane-bound organelles.
For a long time, all prokaryotic life was classified under one kingdom, but pioneering work, most notably by Carl Woese, revealed the profound differences that separated Archaea from Bacteria, warranting their classification into distinct domains.
These differences extend beyond superficial characteristics, delving into the very molecular machinery that governs their cellular functions.
Purpose and Scope
This article aims to provide a clear and concise summary of the key differences between Archaea and Bacteria.
By exploring their unique features, we hope to illuminate the evolutionary divergence that has shaped these two fundamental branches of life and to foster a deeper appreciation for the remarkable diversity and adaptability of the microbial world. We will focus on the core distinctions that set them apart, providing a foundational understanding of their respective roles and significance.
Life on Earth exhibits a breathtaking spectrum of forms, ranging from the macroscopic wonders of towering redwoods and majestic whales to the microscopic marvels that teem in every drop of water and handful of soil. Among these less visible life forms, the microbial world reigns supreme in both its sheer abundance and its staggering diversity. Understanding this microbial realm is not merely an academic exercise; it is crucial for comprehending the very fabric of our planet's ecosystems, the intricacies of human health, and the potential for groundbreaking biotechnological advancements. The Prokaryotic Divide: Archaea and Bacteria Within the vast microbial landscape, two prominent groups stand out: Archaea and Bacteria. These two domains represent fundamental divisions within the prokaryotic world. Prokaryotes are single-celled organisms that lack a nucleus and other complex membrane-bound organelles. For a long time, all prokaryotic life was classified under one kingdom, but pioneering work, most notably by Carl Woese, revealed the profound differences that separated Archaea from Bacteria, warranting their classification into distinct domains. These differences extend beyond superficial characteristics, delving into the very molecular machinery that governs their cellular functions. Now, before we dive deeper into the specific distinctions between Archaea and Bacteria, let’s establish a firm understanding of the foundational characteristics that define prokaryotic life.
Prokaryotic Life: A Fundamental Overview
Prokaryotes represent a cornerstone of life on Earth.
Their simple cellular organization belies their immense impact on the planet's biogeochemical cycles, ecological balance, and even the evolution of more complex life forms.
Defining Prokaryotes
The defining characteristic of prokaryotes is the absence of a nucleus.
Unlike eukaryotic cells, which possess a membrane-bound nucleus to house their genetic material, prokaryotic cells have their DNA located in the cytoplasm, in a region called the nucleoid.
This lack of internal compartmentalization extends to other membrane-bound organelles as well. Prokaryotes do not possess mitochondria, endoplasmic reticulum, Golgi apparatus, or other complex structures found in eukaryotes.
This streamlined cellular architecture allows for rapid reproduction and adaptation, but also limits their overall size and complexity.
The Ubiquitous Nature of Prokaryotes
Prokaryotes are the most abundant and widespread life forms on Earth.
They thrive in virtually every conceivable environment, from the deepest ocean trenches to the highest mountain peaks, and even within the bodies of other organisms.
Their adaptability is a testament to their evolutionary resilience and metabolic versatility.
A single gram of soil can contain billions of prokaryotic cells, representing thousands of different species.
Their metabolic diversity allows them to utilize a wide range of energy sources, including sunlight, organic matter, and inorganic compounds.
Ecological Importance and Roles
Prokaryotes play vital roles in numerous ecosystems.
They are essential for nutrient cycling, including the decomposition of organic matter, the fixation of nitrogen, and the cycling of carbon, sulfur, and phosphorus.
In aquatic environments, prokaryotes form the base of the food web, supporting a vast array of larger organisms.
Some prokaryotes are also important symbionts, forming mutually beneficial relationships with plants and animals.
For example, nitrogen-fixing bacteria in the roots of legumes convert atmospheric nitrogen into a form that plants can use.
Furthermore, prokaryotes are essential for human health.
The human gut microbiome, composed primarily of bacteria, plays a critical role in digestion, immunity, and vitamin synthesis.
Disruptions in the gut microbiome have been linked to a variety of diseases, highlighting the importance of these microbial communities for human well-being.
In summary, prokaryotes, defined by their lack of a nucleus and other complex organelles, are extraordinarily abundant and perform essential ecological roles, underscoring their significance in the grand tapestry of life.
Prokaryotic Life: A Fundamental Overview highlighted the shared characteristics that define this domain. Now, let's narrow our focus and venture into the fascinating world of Archaea, a group of microorganisms that are not only distinct from Bacteria but also hold profound clues about the early evolution of life on Earth.
Archaea: Ancient Pioneers of Extreme Environments
Archaea, often dubbed the ancient pioneers, represent a domain of life as distinct from Bacteria as Eukaryotes (organisms with cells containing a nucleus) are from both. Their discovery revolutionized our understanding of the tree of life and highlighted the remarkable adaptability of life in extreme conditions.
The Woese Revolution and 16S rRNA
The traditional classification of life had long placed all prokaryotes into a single kingdom. This view was challenged by Carl Woese in the 1970s.
Woese's groundbreaking work centered on the analysis of 16S ribosomal RNA (rRNA), a molecule present in all living organisms and vital for protein synthesis.
By comparing the 16S rRNA sequences of various prokaryotes, Woese uncovered a fundamental divide. The rRNA sequences of certain prokaryotes were so distinct from those of Bacteria that they warranted their own separate domain: Archaea.
This discovery was met with initial skepticism but has since become a cornerstone of modern biology, reshaping our understanding of evolutionary relationships.
Unique Characteristics of Archaea
Archaea possess a unique set of characteristics that distinguish them from both Bacteria and Eukaryotes. These differences extend from the molecular level to their ecological adaptations.
Cellular Structure: A Tale of Membranes and Walls
The cell membrane of Archaea differs significantly from that of Bacteria. Bacterial membranes are composed of phospholipids with ester linkages connecting the glycerol backbone to fatty acids.
In contrast, archaeal membranes contain isoprenoids linked to glycerol via ether linkages.
These ether linkages are more resistant to heat and chemical degradation, a crucial adaptation for many Archaea inhabiting extreme environments.
Some Archaea even possess a lipid monolayer, where the isoprenoids of both halves of the membrane are fused, forming a single layer that further enhances stability.
The cell wall composition also sets Archaea apart. Unlike Bacteria, Archaea lack peptidoglycan in their cell walls. Instead, most Archaea possess a cell wall composed of a surface-layer protein (S-layer).
Others may have walls made of polysaccharides. This difference in cell wall composition is a key distinguishing feature between the two domains.
Ribosomes: The Protein Factories
Archaea, like Bacteria, possess ribosomes responsible for protein synthesis. However, archaeal ribosomes exhibit similarities to both bacterial and eukaryotic ribosomes.
For example, the size of archaeal ribosomes is similar to that of bacteria (70S), but their structure and sensitivity to certain antibiotics more closely resemble those of Eukaryotes.
DNA Characteristics and Organization
The DNA of Archaea shares some similarities with Eukaryotes. Like Eukaryotes, archaeal DNA is associated with histone-like proteins, which help to organize and compact the DNA.
This is in contrast to Bacteria, where DNA is typically not associated with histones. Additionally, the process of DNA replication, transcription, and translation in Archaea shares similarities with eukaryotic processes, further highlighting the evolutionary link between these two domains.
Extremophiles: Masters of the Extreme
One of the most remarkable characteristics of Archaea is their ability to thrive in extreme environments. Many Archaea are extremophiles, meaning they are adapted to survive and flourish in conditions that would be lethal to most other organisms.
Thermophiles: Embracing the Heat
Thermophiles are Archaea that thrive in high-temperature environments, such as hot springs, geysers, and hydrothermal vents.
These organisms possess enzymes and proteins that are remarkably stable at high temperatures, allowing them to function optimally in these extreme conditions.
Halophiles: Lovers of Salt
Halophiles are Archaea that flourish in environments with high salt concentrations, such as salt lakes and salterns.
These organisms have evolved mechanisms to maintain osmotic balance in their cells, preventing water loss in highly saline environments.
Methanogens: Methane Producers
Methanogens are Archaea that produce methane as a metabolic byproduct in anaerobic conditions.
They play a crucial role in the global carbon cycle and are found in a variety of environments, including wetlands, sediments, and the digestive tracts of animals.
Ecological Roles and Significance
Archaea play diverse and significant ecological roles in various environments. They are involved in nutrient cycling, decomposition, and other processes essential for maintaining ecosystem health.
Their presence in extreme environments expands the boundaries of the biosphere and challenges our understanding of the limits of life. Furthermore, Archaea are increasingly recognized for their potential in biotechnological applications, such as the production of biofuels and enzymes.
Archaea’s remarkable adaptations to extreme environments and unique molecular signatures underscore their distinct place in the tapestry of life. This understanding paves the way to explore another domain of prokaryotic life: Bacteria, often referred to as the "true bacteria," which exhibit their own set of diverse characteristics and play equally crucial roles in the biosphere.
Bacteria: The Realm of True Bacteria
Bacteria, forming a vast and diverse domain of prokaryotic life, are characterized by their ubiquitous presence across nearly every environment on Earth. From the soil beneath our feet to the depths of the ocean and even within the bodies of other organisms, bacteria thrive and exert significant influence. Their versatility stems from a wide array of metabolic capabilities and structural adaptations, setting them apart from other forms of life.
General Characteristics of Bacteria
Bacteria are single-celled microorganisms lacking a nucleus and other membrane-bound organelles. Their sizes typically range from 0.5 to 5 micrometers, although some species can be significantly larger. Reproduction occurs primarily through binary fission, a process of asexual reproduction in which one cell divides into two identical daughter cells. This rapid reproduction rate, combined with their ability to acquire new genetic material through horizontal gene transfer, contributes to the remarkable adaptability and evolutionary potential of bacteria.
Cellular Structure: Walls, Membranes, and More
Bacterial cells are structurally diverse, yet they share some common features.
Cell Wall and Peptidoglycan
A defining characteristic of most bacteria is the presence of a rigid cell wall composed primarily of peptidoglycan. This complex polymer, unique to bacteria, provides structural support and protects the cell from osmotic lysis. The structure of peptidoglycan varies between bacterial species, forming the basis for the Gram staining technique, which differentiates bacteria into Gram-positive and Gram-negative types based on cell wall composition. Gram-positive bacteria possess a thick layer of peptidoglycan, while Gram-negative bacteria have a thinner layer surrounded by an outer membrane containing lipopolysaccharide (LPS).
Cell Membrane Composition
Beneath the cell wall lies the cell membrane, a phospholipid bilayer that encloses the cytoplasm. The bacterial cell membrane functions as a selective barrier, regulating the passage of substances into and out of the cell. It also plays a crucial role in energy production, housing the electron transport chain in aerobic bacteria. The lipid composition of the bacterial cell membrane differs from that of Archaea and Eukaryotes, primarily composed of phospholipids with ester linkages.
Ribosomes: Protein Synthesis Powerhouses
Like all living organisms, bacteria contain ribosomes, the molecular machines responsible for protein synthesis. Bacterial ribosomes, known as 70S ribosomes, are smaller than the 80S ribosomes found in eukaryotic cells. This difference in ribosome structure is a target for many antibiotics that selectively inhibit bacterial protein synthesis without affecting the host organism.
DNA and RNA: Genetic Blueprints and Messengers
The bacterial genome typically consists of a single, circular chromosome located in the cytoplasm within a region called the nucleoid. In addition to the chromosome, bacteria may also contain plasmids, small, circular DNA molecules that carry genes encoding for specific traits, such as antibiotic resistance or metabolic capabilities.
Bacteria utilize DNA as the genetic blueprint and RNA as the messenger molecule to carry genetic information from DNA to ribosomes for protein synthesis. The process of gene expression in bacteria involves transcription of DNA into RNA and translation of RNA into protein, which is often regulated by environmental factors.
Diversity in Morphology, Metabolism, and Ecological Roles
Bacteria exhibit remarkable diversity in their morphology, metabolism, and ecological roles.
Morphologically, bacteria can be classified into various shapes, including cocci (spherical), bacilli (rod-shaped), and spirilla (spiral-shaped). Metabolically, bacteria employ a wide range of strategies for obtaining energy and carbon, including photosynthesis, chemosynthesis, and heterotrophic consumption of organic matter.
Ecologically, bacteria play diverse roles in various environments, acting as decomposers, pathogens, mutualistic symbionts, and primary producers. Some bacteria are capable of fixing atmospheric nitrogen, converting it into ammonia, which is essential for plant growth. Others play a crucial role in the cycling of nutrients, such as carbon, sulfur, and phosphorus.
Ecological Importance: Nutrient Cycling and Decomposition
Bacteria are essential for maintaining the health and stability of ecosystems. They play a critical role in nutrient cycling, breaking down complex organic matter into simpler compounds that can be used by other organisms. For instance, bacteria are involved in the decomposition of dead plants and animals, releasing nutrients back into the soil and water. They also participate in the nitrogen cycle, converting nitrogen gas into forms that plants can use.
In addition to nutrient cycling, bacteria are also important for decomposition. They break down organic matter, such as dead plants and animals, into simpler compounds. This process helps to recycle nutrients and prevent the build-up of organic waste.
Archaea’s remarkable adaptations to extreme environments and unique molecular signatures underscore their distinct place in the tapestry of life. This understanding paves the way to explore another domain of prokaryotic life: Bacteria, often referred to as the "true bacteria," which exhibit their own set of diverse characteristics and play equally crucial roles in the biosphere.
Archaea vs. Bacteria: A Side-by-Side Comparison
While both Archaea and Bacteria are prokaryotes, lacking a nucleus and other membrane-bound organelles, significant differences exist in their fundamental molecular biology. These distinctions, often invisible to the naked eye, reveal divergent evolutionary paths and specialized adaptations. A direct comparison illuminates these key differences, showcasing the unique nature of each domain.
Key Areas of Divergence
The most striking differences between Archaea and Bacteria lie in their cell wall composition, membrane lipids, ribosome sensitivity to antibiotics, and DNA/RNA structures. These molecular-level variations have profound implications for their respective physiologies and ecological niches. The subsequent sections will delve into each of these characteristics, highlighting what makes these two groups unique.
Cell Wall Composition: A Defining Difference
Perhaps the most well-known distinction is the presence or absence of peptidoglycan in the cell wall. Bacteria invariably possess a cell wall composed of peptidoglycan, a mesh-like structure made of sugars and amino acids. This rigid layer provides structural support and protection.
In contrast, Archaea lack peptidoglycan entirely. Instead, they employ a variety of cell wall structures, the most common being a surface layer composed of protein or glycoprotein, known as an S-layer. This difference is a fundamental characteristic that separates these two domains.
Cell Membrane Lipid Composition: Mirror Images
The lipid composition of the cell membrane also differs significantly between Archaea and Bacteria. Bacterial membranes are typically composed of phospholipids with ester linkages connecting fatty acids to a glycerol backbone.
Archaea, on the other hand, possess unique lipids with ether linkages between isoprenoids and glycerol. These ether linkages are more resistant to heat and chemical attack, contributing to the ability of some Archaea to thrive in extreme environments. Furthermore, archaeal membrane lipids can form tetraethers, creating lipid monolayers that further enhance membrane stability.
Ribosome Sensitivity to Antibiotics: A Target for Selective Inhibition
Ribosomes, the protein synthesis machinery of the cell, also exhibit differences in sensitivity to antibiotics. Many antibiotics that effectively inhibit bacterial ribosomes have no effect on archaeal ribosomes.
This is due to differences in the structure and composition of the ribosomal RNA (rRNA) and ribosomal proteins. This differential sensitivity is often exploited in research to selectively inhibit bacterial growth while leaving archaeal populations unaffected.
DNA Structure and Organization: Subtle but Significant
While both Archaea and Bacteria possess circular chromosomes, differences exist in their DNA structure and organization. Archaea have histone proteins, similar to those found in eukaryotes, that package and organize DNA. Bacteria lack histones.
Furthermore, differences exist in the enzymes involved in DNA replication, transcription, and translation. These differences highlight the distinct evolutionary pathways of these two domains.
RNA Polymerase Structure: A Reflection of Evolutionary Distance
The RNA polymerase, the enzyme responsible for transcribing DNA into RNA, also differs significantly between Archaea and Bacteria. Bacterial RNA polymerase is a relatively simple enzyme composed of a few subunits.
Archaeal RNA polymerase, however, is more complex, bearing a closer resemblance to eukaryotic RNA polymerase II. This similarity provides further evidence for the close evolutionary relationship between Archaea and Eukaryotes.
Unveiling Evolutionary History Through Phylogeny
The field of phylogeny, which focuses on understanding the evolutionary relationships between organisms, is critical for understanding the divergence of Archaea and Bacteria. Comparative analysis of ribosomal RNA (rRNA) sequences, particularly 16S rRNA, has been instrumental in delineating these domains.
These studies reveal that Archaea and Bacteria represent distinct evolutionary lineages that diverged early in the history of life. Studying their evolutionary relationships provides valuable insights into the origins and diversification of life on Earth.
Archaea and Bacteria showcase the diversity of life, but their distinctions lead to a bigger question: how do they relate to each other and to the rest of life on Earth? Understanding their evolutionary history is crucial to grasping the full picture of life's origins and development, and it is this exploration of evolutionary relationships and adaptive mechanisms that is discussed in the following section.
Evolutionary Implications: Tracing the Tree of Life
The domains of Archaea, Bacteria, and Eukaryotes represent the three primary branches in the tree of life. Unraveling their relationships has been a monumental task, relying on molecular data, genomic comparisons, and phylogenetic analyses. This investigation reveals not just how they differ, but why they differ, providing insights into the forces that have shaped life on Earth.
The Three Domains and the Last Universal Common Ancestor (LUCA)
The current consensus places Archaea and Eukaryotes as more closely related to each other than either is to Bacteria. This understanding stems primarily from analyses of ribosomal RNA (rRNA) and other conserved genes.
These molecular comparisons suggest a shared ancestry, with Archaea and Eukaryotes diverging from a common ancestor after the split from Bacteria. The hypothetical ancestor of all life, often referred to as LUCA (Last Universal Common Ancestor), likely possessed a simpler genetic makeup.
It also would have had metabolic capabilities that were subsequently refined and diversified in its descendants.
Endosymbiotic Theory and the Origin of Eukaryotes
The endosymbiotic theory provides a compelling explanation for the origin of Eukaryotes. This theory proposes that mitochondria and chloroplasts, key organelles within eukaryotic cells, were once free-living bacteria. These bacteria were engulfed by an ancestral archaeal cell, establishing a symbiotic relationship.
Over time, these endosymbionts evolved into the organelles we recognize today, contributing essential functions like energy production (mitochondria) and photosynthesis (chloroplasts) to their host cells. The evidence supporting this theory is substantial. It includes the double membranes surrounding these organelles, their own distinct DNA, and the bacterial-like ribosomes found within them.
Evolution as a Driver of Adaptation
Evolution, driven by natural selection, is the engine behind the remarkable adaptations seen in both Archaea and Bacteria. Their ability to thrive in diverse and often extreme environments is a testament to the power of evolutionary processes.
Adaptation is a dynamic process, shaped by environmental pressures and genetic variations. Horizontal gene transfer (HGT) plays a significant role, especially in Bacteria, allowing for the rapid spread of advantageous genes across different species.
This horizontal exchange accelerates adaptation, enabling bacteria to quickly acquire traits like antibiotic resistance or novel metabolic capabilities.
The Importance of Studying Microbial Evolution
Understanding the evolutionary history of Archaea and Bacteria is not merely an academic exercise. It has profound implications for various fields, including medicine, biotechnology, and environmental science. By studying how these microorganisms have adapted to different environments, we can gain insights into developing new drugs, engineering novel metabolic pathways, and mitigating environmental challenges.
Furthermore, unraveling the evolutionary relationships between these domains sheds light on the fundamental processes that have shaped the biosphere, providing a deeper appreciation for the intricate web of life on Earth.
Video: Archaebacteria vs Eubacteria: The Ultimate Summary!
Archaebacteria vs. Eubacteria: Your Burning Questions Answered
Still scratching your head about archaebacteria and eubacteria? This FAQ section tackles the most common questions to solidify your understanding.
What's the single biggest difference between archaebacteria and eubacteria?
While both are prokaryotes, their cell wall composition is a key differentiator. Eubacteria have peptidoglycan in their cell walls, a substance absent in archaebacterial cell walls. Understanding the differences in cell structure is crucial for an archaebaceria and eubacteria summary.
Where are archaebacteria typically found?
Archaebacteria often thrive in extreme environments, such as hot springs, salt lakes, and anaerobic conditions. These harsh conditions where many eubacteria cannot survive, make archaebacteria uniquely adapted. This is a key feature to remember for your archaebaceria and eubacteria summary.
Are archaebacteria and eubacteria more closely related to each other or to eukaryotes?
Archaebacteria are actually more closely related to eukaryotes (organisms with a nucleus, like plants and animals) than they are to eubacteria. This surprising relationship is based on similarities in their ribosomal RNA and other biochemical features, This is a interesting feature that makes the archaebaceria and eubacteria summary unique.
Why is understanding the differences between archaebacteria and eubacteria important?
Understanding these differences is crucial for comprehending the diversity of life and evolutionary relationships. It also has practical applications in fields like biotechnology and environmental science. Studying an archaebaceria and eubacteria summary helps to broaden your general understanding of biology.