Approach Temp: Secrets of Heat Exchangers Revealed!
Understanding the nuances of heat exchanger performance is crucial for efficient thermal management in diverse engineering applications. Approach temperature heat exchanger design fundamentally impacts the overall efficiency of systems. Shell and tube heat exchangers, commonly employed in industries, exhibit performance directly related to the approach temperature. The reduction of this temperature differential, which can be achieved with careful attention to design variables, minimizes energy consumption, a goal championed by organizations like ASME (American Society of Mechanical Engineers). Furthermore, precise calculations, often performed using software like HTRI (Heat Transfer Research Institute) software, are essential to optimize the approach temperature of a heat exchanger, balancing performance with cost and operational constraints.
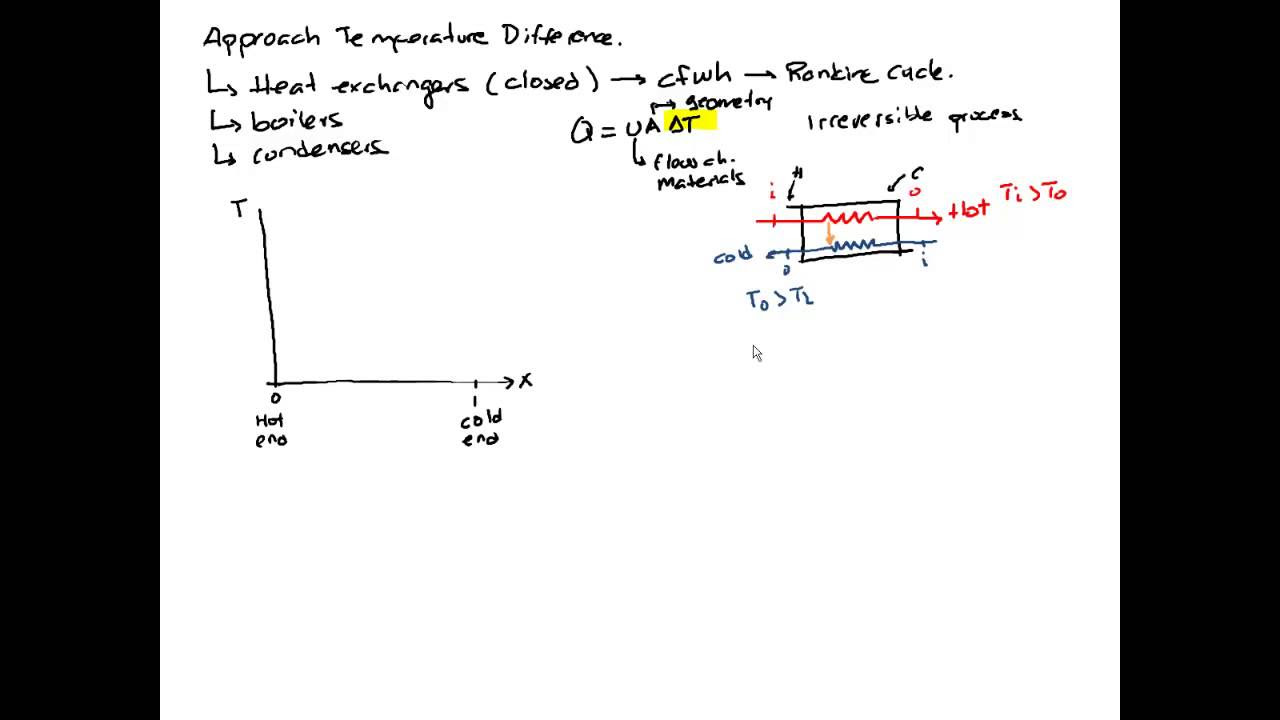
Image taken from the YouTube channel César Gutiérrez PR , from the video titled Approach Temperature Difference .
Heat exchangers are the unsung heroes of countless industrial processes.
From power generation and chemical processing to HVAC systems and even food production, these devices play a vital role.
They efficiently transfer heat between two or more fluids, enabling processes that would otherwise be impossible or highly inefficient.
The effective design and operation of a heat exchanger hinges on a deep understanding of temperature dynamics.
Specifically, the concept of approach temperature is a critical parameter that dictates performance, efficiency, and overall cost-effectiveness.
The Ubiquitous Heat Exchanger
Heat exchangers are integral components in a wide array of industries:
- Chemical Processing: Controlling reaction temperatures, recovering waste heat.
- Power Generation: Steam generation and condensation, cooling turbine exhaust.
- HVAC Systems: Heating and cooling buildings, maintaining comfortable environments.
- Petroleum Refining: Crude oil processing, product cooling.
- Food and Beverage: Pasteurization, sterilization, and temperature control.
The ability to transfer heat efficiently allows for significant energy savings.
It also allows for precise control over process conditions and optimized resource utilization.
Without heat exchangers, many modern industrial processes would simply not be viable.
The Significance of Temperature Difference
At the heart of heat exchanger operation lies the fundamental principle of temperature difference.
Heat naturally flows from a hotter fluid to a colder fluid, and the rate of this heat transfer is directly proportional to the temperature difference between them.
However, it's not just the overall temperature difference that matters.
The specific temperature relationships at the inlet and outlet points of the heat exchanger are equally crucial.
This is where the concept of approach temperature comes into play.
Decoding Approach Temperature
Approach temperature is a key indicator of heat exchanger performance.
It represents the temperature difference between the outlet temperature of one fluid stream and the inlet temperature of the other.
This seemingly simple metric provides valuable insights into how effectively the heat exchanger is operating.
A lower approach temperature generally signifies more efficient heat transfer.
It indicates that the heat exchanger is able to extract more heat from the hot fluid.
It also allows it to transfer that heat more effectively to the cold fluid.
However, achieving lower approach temperatures often requires larger heat exchangers.
This leads to higher capital costs.
Therefore, a careful balance must be struck between performance and investment.
Purpose of This Article
This article aims to demystify the concept of approach temperature.
It will provide a comprehensive understanding of its implications.
We will explore the factors that influence approach temperature.
We will also explore the strategies for optimizing it in various applications.
By understanding approach temperature, engineers and operators can make informed decisions.
These decisions will lead to improved heat exchanger design, enhanced energy efficiency, and reduced operating costs.
The Significance of Temperature Difference
At the heart of heat exchanger operation lies the fundamental principle of temperature difference. Heat naturally flows from a hotter fluid to a colder fluid, and the rate of this heat transfer is directly proportional to the temperature difference between them. However, it's not just the overall temperature difference that matters. The specific temperature relationships at the inlet and outlet points of the heat exchanger are equally crucial. This is where...
Defining Approach Temperature: A Key Indicator of Heat Exchanger Performance
Approach temperature is a critical metric for gauging the efficiency and effectiveness of a heat exchanger. It sheds light on how closely the outlet temperature of one fluid stream approaches the inlet temperature of the other. Understanding and optimizing this parameter is paramount to achieving desired performance and minimizing energy consumption.
What is Approach Temperature?
Approach temperature is defined as the temperature difference between the outlet temperature of one fluid stream and the inlet temperature of the other fluid stream in a heat exchanger. In simpler terms, it tells us how close the exit temperature of the cooled fluid gets to the inlet temperature of the heating fluid (or vice versa).
This temperature difference is a direct indicator of how effectively heat is being transferred. A smaller approach temperature signifies that the fluids are exchanging heat more thoroughly.
Why is Approach Temperature Crucial?
Approach temperature is a crucial parameter for several reasons:
- Performance Indicator: It serves as a key performance indicator (KPI) for heat exchanger efficiency. Lower approach temperatures generally indicate higher heat recovery and better utilization of energy.
- Design Parameter: It is a critical parameter in the design and selection of heat exchangers. The desired approach temperature influences the required heat transfer area, the type of heat exchanger, and the overall cost.
- Operational Optimization: Monitoring approach temperature during operation can help identify fouling, flow imbalances, or other issues that may be hindering performance.
Approach Temperature and Heat Exchanger Performance
The relationship between approach temperature and heat exchanger performance is inversely proportional:
- Lower Approach Temperatures = Higher Efficiency (Generally): Achieving a smaller approach temperature means extracting more heat from the hot fluid or transferring more heat to the cold fluid. This leads to higher energy efficiency and reduced operating costs.
- Lower Approach Temperatures = Larger Heat Exchangers: To achieve a lower approach temperature, a larger heat transfer surface area is typically required. This translates to a larger and more expensive heat exchanger.
This inverse relationship necessitates a careful economic evaluation to balance capital costs (heat exchanger size) and operating costs (energy consumption) to determine the optimal approach temperature for a specific application.
Counter-Flow vs. Parallel-Flow: Impact on Approach Temperature
The configuration of a heat exchanger significantly impacts the achievable approach temperature. The two primary configurations are:
- Counter-Flow Heat Exchangers: In counter-flow heat exchangers, the two fluids flow in opposite directions. This configuration allows for a more uniform temperature difference along the length of the exchanger. Counter-flow designs can achieve significantly lower approach temperatures compared to parallel-flow designs.
- Parallel-Flow Heat Exchangers: In parallel-flow heat exchangers, the two fluids flow in the same direction. The temperature difference between the fluids decreases rapidly along the length of the exchanger, limiting the achievable approach temperature.
The ability of counter-flow heat exchangers to achieve lower approach temperatures makes them preferable for applications where maximum heat recovery is desired.
Log Mean Temperature Difference (LMTD) and Approach Temperature
The Log Mean Temperature Difference (LMTD) is a crucial concept in heat exchanger analysis. It represents the average temperature difference driving heat transfer in the heat exchanger.
LMTD is used in the following equation to calculate the overall heat transfer rate (Q):
Q = U A LMTD
Where:
- Q = Heat transfer rate
- U = Overall heat transfer coefficient
- A = Heat transfer area
- LMTD = Log Mean Temperature Difference
The approach temperature is directly incorporated into the calculation of the LMTD. By manipulating the equation and understanding the desired heat transfer rate, engineers can determine the appropriate LMTD and, consequently, the required approach temperature for a given application. LMTD is essential for accurately predicting heat exchanger performance and optimizing its design.
Factors Influencing Approach Temperature: A Deep Dive
The pursuit of optimal heat exchanger performance inevitably leads to a closer examination of the factors that govern approach temperature. It's not a static value; rather, it's a dynamic outcome influenced by a complex interplay of operational parameters and physical characteristics. Understanding these influences is crucial for both design and operational optimization.
Flow Rates and Fluid Properties
Flow rates exert a significant influence on heat transfer rates within a heat exchanger. Higher flow rates generally lead to increased turbulence, promoting better mixing and enhanced heat transfer. However, this comes at the cost of increased pressure drop and potentially higher pumping power requirements.
Fluid properties such as viscosity, thermal conductivity, specific heat capacity, and density also play a critical role. Fluids with higher thermal conductivity and specific heat capacity are better at absorbing and transferring heat.
Viscosity, on the other hand, can impede heat transfer, especially at lower flow rates, by increasing the resistance to fluid movement and reducing turbulence. The interplay of these fluid properties dictates the overall heat transfer coefficient and, consequently, the achievable approach temperature.
The Impact of Laminar and Turbulent Flow
The nature of the flow regime, whether laminar or turbulent, profoundly impacts heat transfer efficiency. Turbulent flow, characterized by chaotic fluid motion and enhanced mixing, generally promotes significantly higher heat transfer coefficients compared to laminar flow.
Achieving and maintaining turbulent flow within the heat exchanger is often a key design consideration for minimizing approach temperature. Strategies to promote turbulence include increasing flow velocities (within acceptable pressure drop limits) and utilizing enhanced heat transfer surfaces.
The Heat Transfer Coefficient (U-Value)
The overall heat transfer coefficient, commonly denoted as the U-value, is a critical parameter that encapsulates the combined thermal resistance of the heat exchanger. It represents the rate of heat transfer per unit area per unit temperature difference.
A higher U-value signifies a more efficient heat exchanger, capable of transferring more heat for a given temperature difference and surface area. The U-value is influenced by several factors, including the thermal conductivities of the materials used in the heat exchanger, the thickness of the walls separating the fluids, and the convective heat transfer coefficients on both the hot and cold fluid sides.
The relationship between the U-value, the heat transfer area (A), and the Log Mean Temperature Difference (LMTD) is expressed in the fundamental heat transfer equation:
Q = U A LMTD
Where:
- Q is the rate of heat transfer.
- U is the overall heat transfer coefficient.
- A is the heat transfer area.
- LMTD is the Log Mean Temperature Difference.
This equation highlights that, for a given heat duty (Q) and LMTD (which is directly related to the approach temperature), the required heat transfer area (A) is inversely proportional to the U-value.
Therefore, a higher U-value allows for a smaller heat exchanger to achieve the same heat transfer rate.
Fouling Factor: An Insidious Impediment
Fouling, the accumulation of unwanted deposits on heat transfer surfaces, is a common and often unavoidable phenomenon in heat exchangers. These deposits can include scale, rust, organic matter, and biological growth. Fouling acts as an additional thermal resistance, impeding heat transfer and reducing the overall U-value of the heat exchanger.
The fouling factor is a measure of this thermal resistance due to fouling. A higher fouling factor indicates a greater level of fouling and a more significant reduction in heat transfer efficiency.
Fouling significantly impacts the achievable approach temperature. As fouling increases, the heat exchanger's ability to transfer heat diminishes, leading to a higher approach temperature for the same heat duty. To compensate for the effects of fouling, designers often incorporate a fouling allowance into the heat exchanger design, which essentially means oversizing the heat exchanger to account for the anticipated reduction in performance over time.
Regular cleaning and maintenance are essential for mitigating the impact of fouling and maintaining optimal heat exchanger performance.
Heat Exchanger Type: Shell and Tube vs. Plate
The type of heat exchanger employed can also significantly influence the achievable approach temperature, primarily through its impact on flow patterns and heat transfer characteristics.
Shell and Tube Heat Exchangers
Shell and tube heat exchangers are a robust and versatile design commonly used in a wide range of industrial applications. However, their design often results in lower heat transfer coefficients compared to plate heat exchangers, particularly when dealing with fluids that have low thermal conductivities or high viscosities.
Plate Heat Exchangers
Plate heat exchangers, on the other hand, utilize a series of thin plates to create a large surface area for heat transfer in a compact volume. The corrugated patterns on the plates promote turbulent flow and enhance heat transfer efficiency, leading to higher U-values and the ability to achieve lower approach temperatures compared to shell and tube designs.
The choice of heat exchanger type should be carefully considered based on the specific application requirements, taking into account factors such as fluid properties, flow rates, pressure drop limitations, and the desired approach temperature.
Flow regime, fluid properties, and design considerations all play vital roles in determining approach temperature. But what steps can be taken to actively minimize approach temperature, improve efficiency, and ultimately reduce operating costs? Let's investigate.
Optimizing Approach Temperature: Strategies for Efficiency and Cost Savings
Achieving the lowest possible approach temperature isn't just about squeezing every last bit of energy out of a system; it's about striking a delicate balance between upfront investments and long-term operational gains.
This section delves into strategies for minimizing approach temperature, enhancing energy efficiency, and reducing operating costs, while also acknowledging the necessary trade-offs.
The Capital vs. Operating Cost Dilemma
A lower approach temperature invariably demands a larger heat exchanger. This translates to increased capital expenditure (CAPEX), as more materials and a larger footprint are required.
However, this initial investment can pay dividends over time.
A larger heat exchanger, capable of achieving a lower approach temperature, leads to higher energy efficiency. This, in turn, reduces operating expenses (OPEX) due to lower energy consumption.
The optimal approach temperature, therefore, is the point where the total cost – the sum of CAPEX and OPEX over the heat exchanger's lifespan – is minimized. A comprehensive cost-benefit analysis, considering factors like energy prices, discount rates, and the expected operational life, is crucial in making this determination.
Key Strategies for Approach Temperature Optimization
Several strategies can be employed to optimize approach temperature, each with its own advantages and considerations.
Flow Rate Optimization
Optimizing flow rates is essential for maximizing heat transfer efficiency. Increasing flow rates can induce turbulence, leading to higher heat transfer coefficients and a lower approach temperature.
However, this comes at the expense of increased pressure drop and higher pumping power requirements. There's a sweet spot; the optimal flow rate achieves the best balance between heat transfer enhancement and energy consumption from pumping.
Computational Fluid Dynamics (CFD) simulations can be instrumental in determining this optimal flow rate by accurately modeling fluid flow and heat transfer characteristics within the heat exchanger.
Heat Exchanger Selection
Selecting the appropriate type of heat exchanger is critical. Different types of heat exchangers have inherent advantages and disadvantages regarding heat transfer efficiency and achievable approach temperatures.
Plate heat exchangers, for instance, generally offer higher heat transfer coefficients compared to shell and tube exchangers, allowing for closer approach temperatures in a more compact design. However, they may be less suitable for high-pressure or high-temperature applications.
Shell and tube heat exchangers, on the other hand, are more robust and versatile, but they typically require larger surface areas to achieve the same approach temperature as a plate heat exchanger.
The choice ultimately depends on the specific application requirements, including the fluids involved, operating conditions, and budget constraints.
Fouling Mitigation
Fouling, the accumulation of unwanted deposits on heat transfer surfaces, is a major impediment to heat exchanger performance. It reduces the overall heat transfer coefficient, leading to higher approach temperatures and reduced efficiency.
Effective fouling mitigation strategies are, therefore, essential for maintaining optimal performance.
These strategies include:
-
Regular cleaning: Implementing a schedule for regular cleaning, either manually or using automated systems like online tube cleaning, can prevent excessive fouling buildup.
-
Proper pretreatment of fluids: Removing particulate matter and other contaminants from the fluids before they enter the heat exchanger can significantly reduce fouling rates.
-
Optimizing flow velocities: Maintaining sufficiently high flow velocities can help prevent the settling of particulate matter on heat transfer surfaces.
-
Using anti-fouling coatings: Applying specialized coatings to the heat transfer surfaces can reduce the adhesion of fouling agents.
Energy Efficiency and Heat Recovery
Beyond the core strategies, other factors contribute to overall approach temperature optimization. These include:
-
Energy efficiency: Minimizing energy losses throughout the entire process, not just within the heat exchanger, can reduce the heat load on the system and improve overall efficiency.
-
Heat recovery: Implementing heat recovery systems to capture waste heat and reuse it in other parts of the process can significantly reduce energy consumption and improve overall thermal efficiency. For example, waste heat from a cooling process can be used to preheat fluids entering a heating process, reducing the energy required for heating.
By systematically addressing these strategies and considering the trade-offs involved, it's possible to optimize approach temperature, minimize energy consumption, and achieve substantial cost savings in heat exchanger operation.
Flow regime, fluid properties, and design considerations all play vital roles in determining approach temperature. But what steps can be taken to actively minimize approach temperature, improve efficiency, and ultimately reduce operating costs? Let's investigate.
Optimizing Approach Temperature: Strategies for Efficiency and Cost Savings
Achieving the lowest possible approach temperature isn't just about squeezing every last bit of energy out of a system; it's about striking a delicate balance between upfront investments and long-term operational gains. From the intricacies of heat exchanger design to the nuances of operational parameters, a holistic approach is crucial.
This section delves into strategies for minimizing approach temperature, enhancing energy efficiency, and reducing operating costs, while also acknowledging the necessary trade-offs.
The Capital vs. Operating Cost Dilemma
A lower approach temperature invariably demands a larger heat exchanger. This translates to increased capital expenditure (CAPEX), as more materials and a larger footprint are required.
However, this initial investment can pay dividends over time.
A larger heat exchanger, capable of achieving a lower approach temperature, leads to higher energy efficiency. This, in turn, reduces operating expenses (OPEX) due to lower energy consumption.
The optimal approach temperature, therefore, is the point where the total cost – the sum of CAPEX and OPEX over the heat exchanger's lifespan – is minimized. A comprehensive cost-benefit analysis, considering factors like energy prices, discount rates, and the expected operational life, is crucial in making this determination.
Key Strategies for Approach Temperature Optimization
Several strategies can be employed to optimize approach temperature, each with its own advantages and considerations.
Flow Rate Optimization
Optimizing flow rates is essential for maximizing heat transfer. Too low, and you risk laminar flow and reduced heat transfer coefficients. Too high, and you increase pressure drop and pumping costs. The ideal flow rate is the sweet spot where heat transfer is maximized without excessive energy consumption. Computational fluid dynamics (CFD) can be a valuable tool in determining this optimal point.
Heat Exchanger Selection
Choosing the right type of heat exchanger is paramount. Plate heat exchangers, for instance, often achieve lower approach temperatures compared to shell and tube designs due to their higher surface area-to-volume ratio and efficient counter-current flow patterns.
However, shell and tube exchangers may be more suitable for high-pressure or high-temperature applications. The selection process should consider not only the desired approach temperature but also the specific operating conditions and fluid properties.
Fouling Mitigation
Fouling, the accumulation of unwanted deposits on heat transfer surfaces, is a major impediment to efficient heat transfer. It increases thermal resistance, reduces the overall heat transfer coefficient, and increases approach temperature.
Implementing effective fouling mitigation strategies, such as regular cleaning, chemical treatments, and the use of fouling-resistant materials, is crucial for maintaining optimal performance.
Energy Efficiency and Heat Recovery
Implementing strategies to lower the approach temperature often leads to higher energy efficiency and potential for heat recovery. Waste heat, previously discarded, can be preheated or used for other processes. These methods directly translate to operating cost reduction and minimize carbon footprint.
Video: Approach Temp: Secrets of Heat Exchangers Revealed!
Approach Temperature Heat Exchanger FAQs
This section addresses common questions regarding approach temperature and its importance in heat exchanger applications.
What exactly is approach temperature in the context of a heat exchanger?
Approach temperature refers to the temperature difference between the outlet temperature of one fluid and the inlet temperature of the other fluid. In simpler terms, it's how close the exiting cold fluid gets to the entering hot fluid's temperature in a heat exchanger. A lower approach temperature means a more efficient heat exchanger.
Why is monitoring the approach temperature heat exchanger so important?
Monitoring approach temperature allows you to assess the efficiency and performance of a heat exchanger over time. A significant increase in approach temperature heat exchanger indicates potential problems, such as fouling or reduced flow rates, requiring maintenance.
How does the approach temperature heat exchanger impact overall system efficiency?
A high approach temperature means the heat exchanger isn't transferring heat effectively. This can lead to higher energy consumption, as you'll need to use more energy to achieve the desired heating or cooling effect. Optimizing the approach temperature heat exchanger is crucial for minimizing energy waste.
What factors can cause the approach temperature in a heat exchanger to change?
Several factors can influence the approach temperature in heat exchangers. These include changes in flow rates, variations in fluid temperatures, scaling or fouling on heat transfer surfaces, and air accumulation within the system. Regular monitoring helps pinpoint the cause of deviations.