Aluminum Bohr Model: Guide for Students
Exploring the aluminum bohr model unveils a fascinating intersection of quantum mechanics and elemental chemistry. Niels Bohr, a Danish physicist, significantly contributed to our atomic structure understanding, providing a basis for models like the aluminum Bohr model. The Bohr model concept simplifies the complex electron configurations of elements such as Aluminum, which has 13 electrons organized into distinct energy levels or shells. Visualizing Aluminum's atomic arrangement through tools like interactive simulations, available from educational resources and institutions, helps students grasp fundamental principles of atomic theory.
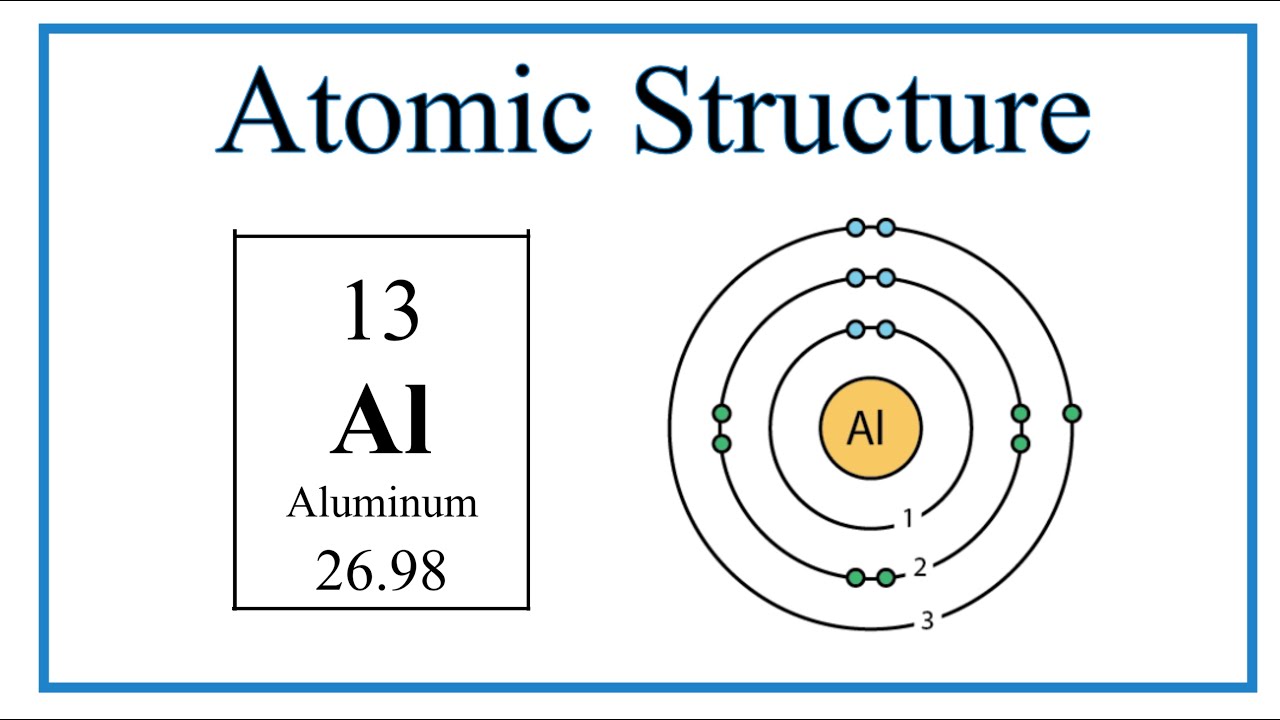
Image taken from the YouTube channel Wayne Breslyn (Dr. B.) , from the video titled Atomic Structure (Bohr Model) for Aluminum (Al) .
Unveiling the Aluminum Bohr Model: A Student's Guide
Let's embark on a journey into the fascinating world of atomic structure, specifically focusing on a simplified yet insightful model: the Aluminum Bohr Model. This model provides a fundamental understanding of how atoms are organized and how their components interact.
It's a stepping stone, a crucial visualization tool, for grasping the more complex realities of quantum mechanics. So, get ready to explore the atom like never before!
A Brief History of Atomic Structure
Our understanding of the atom didn't appear overnight. It's been a gradual process, a series of discoveries and refinements built upon the work of brilliant minds.
From the early philosophical ideas of Democritus to Dalton's atomic theory, the concept of indivisible particles has evolved significantly.
Each step brought us closer to the sophisticated models we use today. Understanding this historical context helps us appreciate the significance of each contribution, including the Bohr model.
Niels Bohr: A Pioneer of Atomic Theory
Niels Bohr stands as a giant in the history of atomic physics. His model, though not entirely accurate by today's standards, was revolutionary for its time.
Bohr proposed that electrons orbit the nucleus in specific, quantized energy levels, much like planets orbiting the sun. This idea introduced the concept of quantization to the atomic world, a radical departure from classical physics.
His work laid the foundation for understanding electron behavior and atomic spectra. This makes him one of the most prominent researchers in modern atomic physics.
Rutherford's Gold Foil Experiment: A Turning Point
Before Bohr, Ernest Rutherford conducted his famous Gold Foil Experiment. This experiment dramatically changed our understanding of the atom.
By firing alpha particles at a thin gold foil, Rutherford observed that some particles were deflected at large angles. This led him to conclude that the atom's positive charge and most of its mass are concentrated in a small, dense nucleus.
This experiment disproved the "plum pudding" model and paved the way for Bohr's model, which incorporated a central nucleus with orbiting electrons.
Aluminum (Al): Our Element of Focus
Now, let's focus on Aluminum (Al), the element we'll be modeling. Aluminum is a common element, found in everything from soda cans to airplanes.
But what makes Aluminum unique at the atomic level? The key lies in its atomic number.
By consulting the Periodic Table, we find that Aluminum has an atomic number of 13. This means that a neutral Aluminum atom has 13 protons in its nucleus and 13 electrons orbiting that nucleus.
This atomic number is crucial for understanding its electronic configuration and how it interacts with other elements. Let's start exploring the Aluminum Bohr model and see what we can discover.
Key Concepts: Energy Levels and Electron Configuration
Now that we've laid the historical groundwork and introduced Aluminum, let's dive into the heart of the Bohr model: energy levels and electron configuration. These concepts are crucial for understanding how electrons are arranged within the atom and, consequently, how Aluminum interacts with other elements.
Think of it like this: understanding electron configuration is like knowing the seating arrangement in a theater. It tells you where each electron "sits" and how much "energy" they have.
Quantized Energy Levels: Orbiting the Nucleus
One of Bohr's most groundbreaking ideas was that electrons don't just orbit the nucleus randomly.
Instead, they exist in specific, quantized energy levels, often visualized as shells or orbits around the nucleus. These energy levels are like rungs on a ladder; an electron can only occupy a specific rung, not the space in between.
This quantization of energy is a fundamental departure from classical physics, where energy is continuous and can take on any value.
Quantum Numbers (n): Defining the Energy Levels
Each energy level is associated with a principal quantum number, denoted by the letter 'n'. The principal quantum number is a positive integer (n = 1, 2, 3, and so on), with higher numbers indicating higher energy levels and greater distances from the nucleus.
For example, n = 1 represents the innermost energy level, closest to the nucleus. This is the lowest energy level an electron can occupy.
As 'n' increases, the energy of the level increases, and the electron is, on average, farther from the nucleus. Think of each 'n' value as a specific "floor" in an atomic building. Electrons "reside" on these floors, with higher floors corresponding to higher energy.
Electron Configuration of Aluminum (Al)
Now, let's apply these concepts to Aluminum. As we know, Aluminum has an atomic number of 13, meaning it has 13 electrons in a neutral atom. How are these 13 electrons arranged in the energy levels?
The electron configuration of Aluminum is 1s² 2s² 2p⁶ 3s² 3p¹.
Let's break this down:
- 1s²: The first energy level (n=1) has one subshell (s), which can hold up to 2 electrons.
- 2s²: The second energy level (n=2) has two subshells (s and p). The s subshell can hold up to 2 electrons.
- 2p⁶: The p subshell can hold up to 6 electrons.
- 3s²: The third energy level (n=3) has three subshells (s, p, and d). The s subshell can hold up to 2 electrons.
- 3p¹: The p subshell contains only 1 electron in this atom of Aluminum.
This notation tells us exactly how many electrons occupy each energy level and subshell in an Aluminum atom.
Valence Electrons: The Key to Chemical Bonding
Of particular importance are the valence electrons. These are the electrons in the outermost energy level (the highest 'n' value) and are responsible for chemical bonding.
For Aluminum, the outermost energy level is n=3, with the electron configuration 3s² 3p¹.
Therefore, Aluminum has 3 valence electrons. These three electrons are what Aluminum uses to interact with other atoms and form chemical bonds. This explains why Aluminum readily forms compounds with other elements.
Visualizing the Aluminum Bohr Model: Diagrams and Animations
Understanding these concepts can be greatly enhanced through visual aids. Diagrams showing the nucleus and electron shells can help you visualize the arrangement of electrons in Aluminum.
Look for diagrams that clearly label the energy levels (n=1, n=2, n=3) and show the number of electrons in each shell.
Even better, animations that show the movement of electrons between energy levels can provide a dynamic understanding of atomic processes. These visual representations can bridge the gap between abstract concepts and concrete understanding, making the Bohr model more accessible and engaging.
Use visuals to better understand the relationships between the nucleus and electron shells.
By understanding energy levels and electron configuration, we gain a powerful tool for predicting and explaining the behavior of Aluminum and other elements.
Electron Transitions and Energy: Excitation and Emission
Having explored the electron configuration of Aluminum, let's now investigate the dynamic processes of electron transitions and how they relate to energy absorption and emission. This understanding reveals how Aluminum interacts with light and other forms of energy.
Think of electrons as tiny dancers, each with a preferred energy level "dance floor." But, unlike dancers, they can change floors if they get the right amount of energy.
Ground State vs. Excited State: Energizing Electrons
Normally, electrons in an Aluminum atom occupy the lowest possible energy levels, filling the innermost shells first. This stable configuration is called the ground state.
However, if an Aluminum atom absorbs energy – perhaps from heat, light, or electrical discharge – one or more of its electrons can jump to a higher energy level, further from the nucleus. This jump results in what is called an excited state.
This is analogous to giving one of our dancers a boost to a higher dance floor, making them less stable in their new position. The electron is temporarily "excited," occupying an energy level it wouldn't normally be in.
Moving Between Energy Levels: The Quantum Leap
The key thing to remember is that electrons don't gradually move between energy levels.
Instead, they make instantaneous jumps, often referred to as quantum leaps. These leaps always involve a very specific amount of energy, exactly matching the difference between the initial and final energy levels.
Think of it like teleporting between floors rather than taking the stairs!
Photon Emission: Releasing Energy as Light
The excited state is unstable. The electron will eventually "fall" back down to a lower energy level, potentially returning to the ground state in one step or several steps.
When this happens, the electron releases the excess energy it absorbed in the form of a photon, a tiny packet of light.
The energy of the emitted photon is precisely equal to the difference in energy between the two levels involved in the transition. In essence, the excited electron is giving back the boost it received in the form of light.
The Electromagnetic Spectrum: A Rainbow of Energy
The emitted photon is part of the electromagnetic spectrum. This is a broad range of electromagnetic radiation, encompassing everything from low-energy radio waves to high-energy gamma rays.
The energy (and therefore the wavelength and frequency) of the emitted photon dictates its position on the electromagnetic spectrum, thus defining the type of light it represents.
For Aluminum, electron transitions can produce photons in the ultraviolet and visible regions of the spectrum, though these transitions are more complex than the Bohr model can fully explain.
Each element has its own specific 'fingerprint' of spectral lines that are produced by the particular electron transitions that are possible for that element.
The Rydberg Formula: Quantifying Energy Transitions
Johannes Rydberg developed a formula that predicts the wavelengths of light emitted when an electron jumps between energy levels in an atom.
While the Rydberg formula works best for hydrogen-like atoms (atoms with only one electron), it provides a foundation for understanding the relationships between energy levels and emitted light in more complex atoms like Aluminum.
The formula highlights the direct connection between energy level differences and the wavelengths of emitted photons, reinforcing the concept of quantized energy.
Understanding electron transitions helps us see the Bohr model as a dynamic representation of the atom, where electrons constantly absorb and emit energy in specific, quantifiable ways. It also helps understand how light is emitted from different elements.
Limitations of the Bohr Model: A Stepping Stone
While the Bohr model offers a valuable initial framework for understanding atomic structure, it's crucial to recognize that it's not a complete picture. It's a simplified representation that falls short when applied to more complex atoms like Aluminum and beyond.
Think of it as a helpful, but ultimately incomplete, map. It gets you started, but you'll need more sophisticated tools to navigate the intricate terrain of the quantum world.
Inaccuracies with Larger Atoms: A Simplified View
One of the most significant limitations of the Bohr model lies in its inability to accurately predict the behavior of atoms with many electrons.
The model treats electrons as if they orbit the nucleus in well-defined paths, like planets around the sun. This is a gross oversimplification.
In reality, the interactions between multiple electrons become incredibly complex, making it impossible to predict their exact positions and energies with the precision suggested by the Bohr model.
The Bohr model struggles to explain the chemical bonding and spectral properties of elements beyond hydrogen, including Aluminum, with its 13 electrons.
It cannot accurately account for phenomena like electron shielding or orbital shapes, which are essential for understanding the behavior of larger atoms.
The Rise of Quantum Mechanics: A More Complete Picture
To overcome the shortcomings of the Bohr model, physicists developed the theory of quantum mechanics.
This is a more advanced and accurate description of atomic structure.
Quantum mechanics replaces the idea of fixed electron orbits with the concept of atomic orbitals.
These are regions of space where electrons are likely to be found.
Instead of orbiting in neat circles, electrons exist in fuzzy, three-dimensional shapes, described by probability distributions.
The Heisenberg Uncertainty Principle
The famous Heisenberg Uncertainty Principle dictates that we cannot know both the position and momentum of an electron with perfect accuracy.
This fundamentally undermines the Bohr model's assumption of well-defined electron orbits.
Quantum mechanics also introduces the concept of wave-particle duality.
Electrons exhibit properties of both particles and waves, further complicating the simple planetary model.
The Bohr Model's Enduring Value
Despite its limitations, the Bohr model remains a valuable tool for introductory chemistry and physics education.
It provides a simple and intuitive way to visualize the basic structure of an atom and to understand the concept of quantized energy levels.
It laid the groundwork for more sophisticated theories.
By understanding the Bohr model's strengths and weaknesses, students can gain a deeper appreciation for the evolution of atomic theory and the power of quantum mechanics.
It serves as an essential stepping stone on the path to understanding the intricacies of the quantum world. It provides that initial picture to build on and refine.
Applications and Significance: Why the Bohr Model Matters
While the Bohr model might seem like a relic of the past compared to the sophisticated models we use today, understanding its relevance and significance is crucial.
Even if its direct applications are limited in our modern, technologically advanced world, the Bohr model served as an indispensable stepping stone in the development of modern atomic theory. It helped shape our current understanding of the atom.
Limited but Illustrative Real-World Applications
It's true, you won't find engineers designing microchips based solely on the Bohr model. Its predictive power for complex systems simply isn't sufficient. However, let's not dismiss its role entirely.
The model offers a simplified, intuitive way to visualize atomic structure. This makes it invaluable in educational settings.
Specifically, the Bohr model laid the foundation for understanding the behavior of semiconductors. These materials are used in everything from computer chips to solar panels.
Although more advanced quantum mechanical models are needed for precise design and optimization, the basic concept of electron energy levels, first introduced by Bohr, is still fundamental.
Think of it this way: The Bohr model provides a conceptual framework. This allows us to grasp the relationship between electronic structure and material properties.
Foundational Role in Atomic Theory
The true significance of the Bohr model lies in its historical context and its role as a catalyst for further scientific breakthroughs.
Before Bohr, the atom was a mysterious entity.
Bohr's postulates, even with their eventual limitations, provided a tangible, workable model that scientists could use to explain observed phenomena.
Here's why it's so important:
- Quantization of Energy: Bohr's most radical idea was the quantization of electron energy levels. This concept revolutionized physics. It paved the way for quantum mechanics.
- Explanation of Atomic Spectra: The model successfully explained the discrete spectral lines observed in hydrogen. This provided strong evidence for the quantized nature of electron energy levels.
- Inspiration for Future Scientists: The Bohr model sparked intense research and debate, motivating scientists like Heisenberg and Schrödinger to develop the more complete theory of quantum mechanics.
The Bohr Model as a Stepping Stone
Imagine building a magnificent skyscraper. You wouldn't start by hoisting the final spire. You'd lay a solid foundation first. The Bohr model is that foundation for our understanding of the atom.
While quantum mechanics provides a more accurate and comprehensive description of atomic structure, it builds upon the conceptual framework established by Bohr.
It is because of the Bohr model that we could begin to ask the right questions and design experiments that would eventually lead to the development of more sophisticated theories.
The principles discovered by scientists like Niels Bohr still influence our understanding of the atom. It's a story of how an incomplete model revolutionized atomic theory.
It is this importance that makes the Bohr Model a lasting legacy in science.
Video: Aluminum Bohr Model: Guide for Students
FAQs: Aluminum Bohr Model Guide
What are the key differences between a Bohr model and the modern atomic model?
The Bohr model, as it applies to the aluminum bohr model, shows electrons orbiting the nucleus in fixed paths or shells. The modern model uses probability to describe electron locations within orbitals, acknowledging that their positions are not precisely defined.
Why is the Bohr model still used to represent the aluminum atom?
Despite its limitations, the Bohr model is a simplified visual representation that effectively illustrates the arrangement of electrons within an aluminum atom. It helps students understand the concept of electron shells and valency, even though the modern model offers a more accurate picture.
What does the number of electrons in the outermost shell of the aluminum bohr model tell us?
The number of electrons in the outermost shell of the aluminum bohr model indicates its valence and how it will interact with other atoms. In aluminum, the three valence electrons explain its tendency to form +3 ions.
How does the aluminum bohr model help understand aluminum's properties?
The aluminum bohr model provides a basic understanding of why aluminum is reactive but also forms stable oxides. The easy loss of three valence electrons explains its reactivity, while the completed outer shell after forming a compound contributes to stability.
So, that's the aluminum Bohr model in a nutshell! Hopefully, this guide has cleared up any confusion and given you a solid understanding. Keep practicing drawing those electron configurations, and you'll be an atom-drawing pro in no time. Good luck with your studies!