Acetal Hydrolysis: The Ultimate Guide You'll Ever Need
Acetal hydrolysis, a crucial reaction in organic chemistry, finds significant applications in diverse fields such as polymer chemistry and drug delivery. Understanding the reaction mechanism is paramount for effective utilization, with factors like pH levels acting as vital determinants of the hydrolysis rate. Professor Arthur Nicolaou's work significantly advanced our comprehension of reaction mechanisms, including related carbonyl chemistry, providing a deeper appreciation of the complex factors influencing acetal hydrolysis. The Department of Chemistry at MIT actively researches these mechanisms using advanced analytical techniques such as NMR spectroscopy.
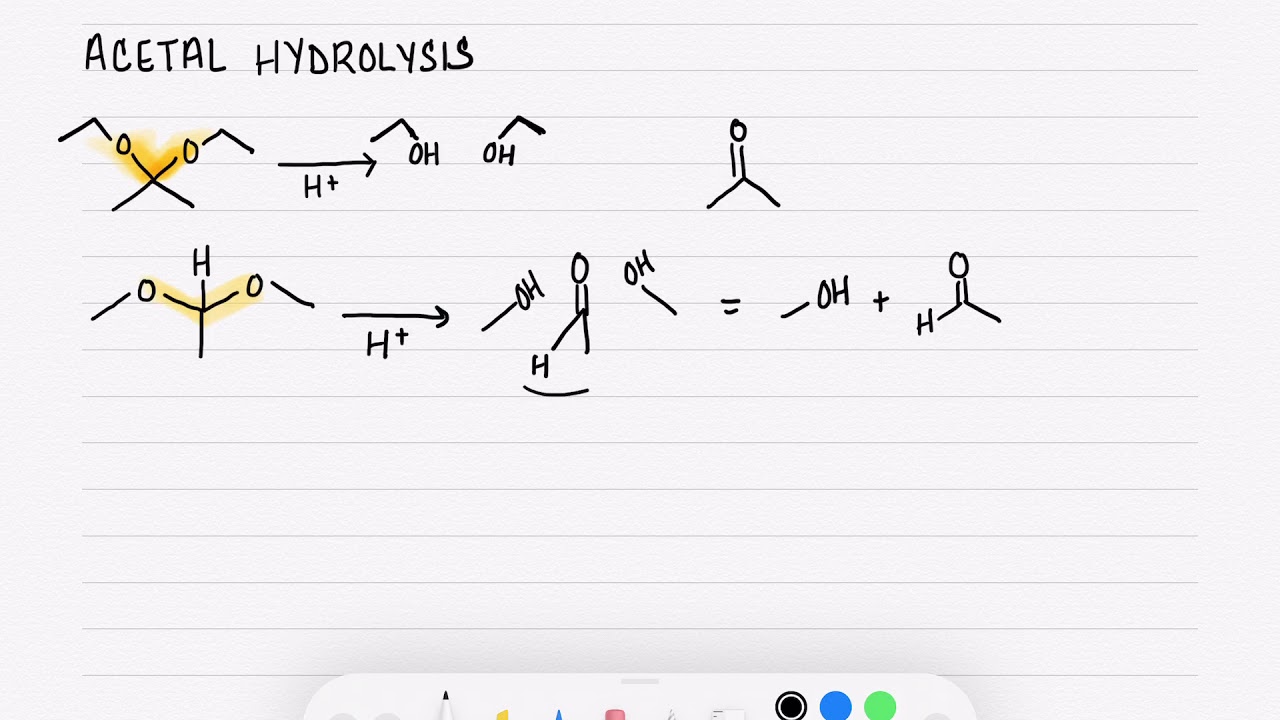
Image taken from the YouTube channel Roxi Hulet , from the video titled 16.8 Hydrolysis of an Acetal .
Organic chemistry, at its core, is about understanding the structure and reactivity of carbon-based molecules. Within this vast landscape, acetals stand out as a fascinating class of compounds with unique properties and a wide range of applications. This section serves as an introduction to the world of acetals, laying the groundwork for a deeper exploration of their behavior, particularly their hydrolysis.
Defining Acetals: Structure and Formation
Acetals and ketals are geminal diethers derived from aldehydes and ketones, respectively. The key difference lies in their origin: acetals are formed from aldehydes, while ketals are formed from ketones.
Acetals are characterized by a central carbon atom bonded to two alkoxy groups (-OR), an alkyl group (R), and a hydrogen atom. Ketals, on the other hand, have two alkoxy groups and two alkyl groups attached to the central carbon.
The general structure of an acetal is RCH(OR')2, where R is an alkyl or aryl group and R' is an alkyl group. This seemingly simple structure unlocks a diverse range of chemical possibilities.
The Reversible Dance: Aldehydes/Ketones + Alcohols ⇌ Acetals/Ketals
Acetals (and ketals) are formed through a reversible reaction between an aldehyde or ketone and an alcohol. This reaction typically requires an acid catalyst to proceed at a reasonable rate.
The general reaction can be represented as follows:
RCHO (aldehyde) + 2 R'OH (alcohol) ⇌ RCH(OR')2 (acetal) + H2O
RCOR (ketone) + 2 R'OH (alcohol) ⇌ RC(OR')2R (ketal) + H2O
The formation of acetals is an equilibrium process, meaning that the reaction can proceed in both forward and reverse directions. Removing water from the reaction mixture favors acetal formation (Le Chatelier's principle).
The Significance of Hydrolysis
Understanding acetal hydrolysis, the reverse of acetal formation, is crucial for several reasons. Acetal hydrolysis is the key to unlocking the carbonyl compound that was used to form the acetal in the first place.
Hydrolysis is the cleavage of a chemical bond by the addition of water. In the case of acetals, hydrolysis results in the breaking of two carbon-oxygen bonds, regenerating the original aldehyde or ketone and releasing two alcohol molecules.
Acetal hydrolysis finds applications in various chemical processes. One notable application is the use of acetals as protecting groups for aldehydes and ketones.
Applications Far and Wide
In industrial settings, acetal hydrolysis is used in the synthesis of various chemicals and pharmaceuticals. In biological systems, enzymatic hydrolysis of acetal-like structures plays a role in the metabolism of carbohydrates and other biomolecules.
The ability to selectively form and hydrolyze acetals makes them valuable tools in organic synthesis. Protecting groups are essential when performing reactions on molecules with multiple reactive functional groups, and acetals are often the protecting group of choice for carbonyls.
Guide Overview
This guide is designed to provide a comprehensive understanding of acetal hydrolysis. We will delve into the detailed mechanism of the reaction, exploring the crucial role of acid catalysis and the formation of key intermediates.
Furthermore, we will examine the factors that influence the rate and equilibrium of acetal hydrolysis, such as pH and substituent effects. Finally, we will discuss the practical applications of acetals as protecting groups, highlighting their importance in organic synthesis. Prepare to embark on a journey into the intricacies of acetal chemistry!
The reversible formation of acetals from aldehydes or ketones and alcohols sets the stage for their equally important reverse reaction: hydrolysis. This process, the breaking of bonds with water, unlocks the protecting potential of acetals and reveals the original carbonyl compounds. Understanding the nuances of this reaction is critical for anyone working with these versatile molecules.
Fundamentals of Acetal Hydrolysis: Breaking Bonds with Water
This section will dive into the fundamental principles governing acetal hydrolysis, the process by which acetals are converted back into their parent aldehydes or ketones. We'll explore the reaction itself, the crucial role of acid catalysis, and the resulting products.
Hydrolysis Defined: Water as a Cleaving Agent
Hydrolysis, in its essence, is the cleavage of a chemical bond through the addition of water. In the context of acetals, this means breaking the carbon-oxygen bonds that define the acetal functional group.
The general reaction scheme for acetal hydrolysis can be represented as follows:
RCH(OR')2 (acetal) + H2O → RCHO (aldehyde) + 2 R'OH (alcohol) RC(OR')2R (ketal) + H2O → RCOR (ketone) + 2 R'OH (alcohol)
Water serves as more than just a solvent; it's an active participant, providing the oxygen atom needed to regenerate the carbonyl group. The equation above highlights water's role.
The Indispensable Role of Acid Catalysis
Acetal hydrolysis proceeds at a negligibly slow rate in neutral or basic conditions. The presence of an acid catalyst is absolutely essential to facilitate the reaction within a reasonable timeframe.
Protonation: The Acid Catalyst's Key Function
The acid catalyst's primary function is to protonate the acetal oxygen. This protonation increases the electrophilicity of the carbon atom bonded to the oxygen, making it more susceptible to nucleophilic attack by water.
This initial protonation step is crucial as it sets the stage for the subsequent bond-breaking events. Without it, the acetal is relatively unreactive towards hydrolysis.
Types of Acid Catalysts
Various acids can serve as catalysts, including both protic acids (e.g., hydrochloric acid, sulfuric acid, p-toluenesulfonic acid) and Lewis acids (e.g., boron trifluoride).
The choice of acid catalyst often depends on the specific acetal and the reaction conditions. Protic acids are the most common choice for laboratory-scale hydrolysis, while Lewis acids may be preferred in specific industrial applications.
Identifying the Products: Carbonyls and Alcohols Reborn
Acetal hydrolysis ultimately yields an aldehyde (from an aldehyde-derived acetal) or a ketone (from a ketone-derived acetal) and two molecules of alcohol.
Structure Determines Products
The structure of the starting acetal directly dictates which aldehyde or ketone is produced. By understanding the starting material, the product can be predicted.
Factors Influencing Product Distribution
In more complex systems with multiple protecting groups or competing reactions, the distribution of products can be influenced by various factors, including:
- Steric hindrance: Bulky substituents near the acetal group may slow down hydrolysis.
- Electronic effects: Electron-donating or electron-withdrawing groups can influence the stability of intermediates.
- Reaction conditions: Temperature, solvent, and the concentration of acid can all play a role in the overall outcome.
Deconstructing the Process: The Acetal Hydrolysis Mechanism
Having established the necessity of acid catalysis and the identity of the products, it's time to delve into the heart of acetal hydrolysis: the intricate mechanism that governs the transformation. Understanding this mechanism is crucial for predicting reactivity, manipulating reaction conditions, and truly appreciating the chemical elegance of this process.
A Step-by-Step Journey Through the Mechanism
Acetal hydrolysis unfolds through a series of carefully orchestrated steps, each involving the movement of electrons and the formation or breaking of chemical bonds. The mechanism can be broken down into distinct stages, with each stage playing a crucial role in the overall transformation.
Step 1: Protonation of the Acetal Oxygen
The first and arguably most important step is the protonation of one of the acetal oxygens by the acid catalyst. This protonation significantly increases the electrophilicity of the carbon attached to that oxygen.
This protonation activates the acetal for subsequent bond cleavage.
Step 2: Cleavage of the Carbon-Oxygen Bond
With the oxygen now protonated, the carbon-oxygen bond becomes weakened, allowing for the departure of an alcohol molecule. This cleavage results in the formation of a carbocation intermediate.
The leaving group is an alcohol, which is protonated after accepting hydrogen.
Step 3: Formation of a Carbocation Intermediate
The carbocation is a highly reactive species with a positive charge on the carbon atom. Its formation is the rate-determining step in the overall hydrolysis mechanism.
The stability of this carbocation will influence the rate of hydrolysis.
Step 4: Nucleophilic Attack of Water
The carbocation, being electron-deficient, is readily attacked by a nucleophile – in this case, water. The oxygen atom of water donates its electron pair to form a new bond with the carbocation carbon.
Step 5: Deprotonation and Hemiacetal Formation
The water molecule that attacked the carbocation is now positively charged. A base (often another water molecule) removes a proton, regenerating the acid catalyst and forming a hemiacetal.
The hemiacetal is a pivotal intermediate.
Step 6: Protonation of the Hemiacetal
The hemiacetal now undergoes protonation on its free hydroxyl group, in a manner analogous to the initial protonation of the acetal. This makes water a better leaving group.
Step 7: Loss of Alcohol and Carbonyl Formation
The protonated hydroxyl group departs as water, leading to the formation of the final carbonyl compound, whether an aldehyde or a ketone, depending on the starting acetal.
The Hemiacetal Intermediate: A Transient Key Player
The hemiacetal intermediate is a fascinating and vital component of the acetal hydrolysis mechanism. It represents a structure that is halfway between the starting acetal and the final carbonyl product.
It is important to understand its role and influence.
Importance and Characteristics
Hemiacetals are generally unstable, especially in acidic conditions, and are quickly converted to the final products. This instability is due to the presence of both an alcohol and an ether functional group on the same carbon atom.
Factors Affecting Stability
Factors such as steric hindrance and electronic effects can influence the stability and reactivity of the hemiacetal. However, under the acidic conditions of acetal hydrolysis, the hemiacetal's conversion to the final carbonyl compound is usually very rapid.
Swift Conversion to Final Products
The hemiacetal's fleeting existence means it's rarely isolated or directly observed during acetal hydrolysis. Its rapid conversion to the carbonyl product is driven by the thermodynamic stability of the aldehyde or ketone. This step completes the cycle.
Acetals as Protecting Groups: Shielding Carbonyls with Precision
Having navigated the intricate mechanism of acetal hydrolysis, we now turn to a practical application of this knowledge: the strategic use of acetals as protecting groups in organic synthesis. The ability to selectively form and hydrolyze acetals makes them invaluable tools for chemists. They allow for the temporary "shielding" of carbonyl functionalities, enabling reactions to proceed at other parts of a molecule without interference.
The Necessity of Carbonyl Protection
Aldehydes and ketones, characterized by their highly reactive carbonyl groups (C=O), are fundamental building blocks in organic chemistry. However, this very reactivity can be a double-edged sword. In multi-step syntheses, where a series of reactions are performed sequentially to build a complex molecule, the carbonyl group can often interfere with desired transformations at other sites within the molecule.
The electrophilic nature of the carbonyl carbon makes it susceptible to attack by nucleophiles. The presence of a carbonyl can also lead to unwanted side reactions such as self-condensation or polymerization.
To circumvent these problems, chemists employ protecting groups: temporary modifications to a functional group that render it unreactive under specific reaction conditions. Once the desired transformation elsewhere in the molecule has been accomplished, the protecting group can be removed, regenerating the original functional group.
Why Acetals Excel as Protecting Groups
Acetals are particularly well-suited for protecting aldehydes and ketones for several reasons:
-
Reactivity Control: Acetals are unreactive towards a wide range of reagents, including strong bases, nucleophiles, oxidizing agents, and reducing agents. This inertness allows chemists to perform a variety of transformations on other parts of the molecule without affecting the carbonyl group.
-
Ease of Formation: The formation of acetals from aldehydes or ketones is generally a straightforward reaction, typically requiring an alcohol and an acid catalyst. The reaction is often driven to completion by removing water, a byproduct of the reaction.
-
Selective Deprotection: The hydrolysis of acetals, as we have explored, is readily achieved under acidic conditions. This allows for the selective removal of the protecting group, unveiling the original carbonyl compound without affecting other acid-sensitive functionalities that may be present in the molecule.
Examples Where Protecting Groups are Essential
Consider a scenario where a chemist wants to reduce an ester group in a molecule containing a ketone. Without protection, the ketone would also be reduced, leading to a mixture of products.
By first converting the ketone to an acetal, the ester can be selectively reduced. The acetal protecting group is then removed, regenerating the desired ketone.
Another common situation involves reactions using Grignard reagents. These reagents are highly reactive and will react with carbonyl groups. Protecting a carbonyl as an acetal allows the Grignard reagent to react selectively with other functional groups, such as esters or halides.
The Art of Selective Hydrolysis: Unveiling the Carbonyl
The real power of acetals as protecting groups lies not only in their ability to mask carbonyl reactivity but also in the ease and selectivity with which they can be removed. This deprotection, or hydrolysis, is typically achieved under aqueous acidic conditions.
However, the art of selective hydrolysis involves carefully controlling the reaction conditions.
Fine-Tuning Reaction Conditions
-
Acid Concentration: The rate of acetal hydrolysis is directly dependent on the concentration of acid. Therefore, the acid concentration must be carefully optimized. Too little acid will result in slow deprotection, while too much acid can lead to unwanted side reactions or the degradation of other acid-sensitive functional groups.
-
Temperature: Elevated temperatures generally accelerate the rate of hydrolysis. However, high temperatures can also promote side reactions. The temperature should be carefully controlled to achieve a balance between reaction rate and selectivity.
-
Solvent Effects: The choice of solvent can also influence the rate and selectivity of acetal hydrolysis. Polar protic solvents, such as water and alcohols, are generally favored.
Considerations for Acid-Sensitive Functional Groups
The presence of other acid-sensitive functional groups in the molecule must also be taken into account. For example, if the molecule contains an acid-labile ester group, strongly acidic conditions should be avoided to prevent its hydrolysis. In such cases, milder acids or buffered solutions may be used.
In conclusion, acetals provide a powerful and versatile means of protecting carbonyl functionalities in organic synthesis. By understanding the principles of acetal formation and hydrolysis, and by carefully controlling the reaction conditions, chemists can wield these protecting groups with precision. This control allows for the synthesis of complex molecules with high selectivity and yield.
Acetals, as protecting groups, provide a critical service in organic synthesis by temporarily masking carbonyl groups. But their utility doesn’t end there. To truly harness their power, we must understand what influences the rate at which these protective shields are removed—that is, the factors affecting acetal hydrolysis.
Factors Influencing Acetal Hydrolysis: A Closer Look at Reactivity
The journey of acetal hydrolysis, as we've seen, involves a delicate dance of protonation, bond cleavage, and nucleophilic attack. However, this dance isn't performed at a constant tempo. Several factors can significantly influence the rate and equilibrium of acetal hydrolysis, with pH and the nature of substituents on the molecule playing leading roles.
The Decisive Role of pH: Acidity is Paramount
pH isn't merely a measurement of acidity; it's a master conductor of the acetal hydrolysis orchestra. The reaction is profoundly sensitive to pH, and acidic conditions are absolutely essential for it to proceed at a reasonable rate.
Why is acidity so crucial? Recall the mechanism: the first step involves the protonation of an acetal oxygen atom. This protonation activates the acetal, making it more susceptible to cleavage of the carbon-oxygen bond.
Without sufficient acid, this initial protonation step is slow and the entire reaction grinds to a halt.
Think of the acid as a catalyst, but also as an enabler. It's not just speeding up the reaction; it's fundamentally necessary for it to occur.
Optimizing pH for Efficient Hydrolysis
So, how do we ensure the reaction has the best chance of success? Maintaining an appropriately acidic environment is key.
However, it's not simply a matter of adding as much acid as possible. Too much acid can lead to unwanted side reactions, especially if other acid-sensitive functional groups are present in the molecule.
The ideal pH range will depend on the specific acetal and the other functional groups present. In practice, a mildly acidic solution, typically with a pH between 1 and 4, is often sufficient. Buffers can be used to maintain a stable pH throughout the reaction. Common acids used include hydrochloric acid (HCl), sulfuric acid (H2SO4), and p-toluenesulfonic acid (TsOH).
Substituent Effects on Reactivity: A Subtle Dance
Beyond pH, the molecular architecture of the acetal itself plays a crucial role. The electronic and steric properties of the substituents attached to the acetal carbon can exert a significant influence on the rate of hydrolysis. This influence is primarily mediated through their impact on the stability of the carbocation intermediate formed during the reaction.
Electronic Effects: Stabilizing the Carbocation
The carbocation intermediate is electron-deficient and therefore highly reactive. Substituents that can donate electron density to the carbocation center will stabilize it, thereby lowering the activation energy of the reaction and accelerating the hydrolysis.
Electron-donating groups (EDGs), such as alkyl groups or alkoxy groups, increase electron density at the carbocation center, reducing the positive charge and stabilizing the intermediate. This, in turn, lowers the activation energy for the formation of the carbocation, speeding up the overall reaction.
Conversely, electron-withdrawing groups (EWGs), such as halogens or nitro groups, pull electron density away from the carbocation center, destabilizing it and slowing down the hydrolysis.
These groups exacerbate the positive charge, making the intermediate even more unstable. This increases the activation energy, thus slowing down the reaction.
Steric Effects: Hindering the Approach
Substituents don't just influence the electronic environment; they also occupy space. Bulky substituents near the acetal carbon can hinder the approach of water molecules during the nucleophilic attack on the carbocation intermediate.
This steric hindrance can significantly slow down the reaction, regardless of the electronic properties of the substituents. The larger the substituents, the more difficult it is for water to access the carbocation center.
In essence, substituents around the acetal carbon engage in a subtle interplay of electronic and steric effects. Understanding these effects allows chemists to fine-tune the reactivity of acetals, optimizing their use as protecting groups and controlling the outcome of complex synthetic reactions.
Video: Acetal Hydrolysis: The Ultimate Guide You'll Ever Need
FAQs: Acetal Hydrolysis Explained
Need a quick refresher on acetal hydrolysis? These frequently asked questions should help clarify key concepts.
What exactly is acetal hydrolysis?
Acetal hydrolysis is a chemical reaction where an acetal is broken down into its corresponding aldehyde or ketone and alcohol, using water and an acid catalyst. Essentially, it's the reverse of acetal formation.
Why is acid needed for acetal hydrolysis?
The acidic environment is crucial for protonating the oxygen atoms in the acetal. This protonation makes the leaving groups (alcohols) better and the reaction proceed more readily. Without the acid catalyst, the reaction is very slow.
Is acetal hydrolysis reversible?
Yes, acetal hydrolysis is a reversible reaction. The reverse reaction, acetal formation, is favored under anhydrous (water-free) conditions and with excess alcohol. Therefore, controlling the amount of water is important.
How is acetal hydrolysis used in organic chemistry?
Acetal hydrolysis is frequently used as a protecting group strategy in organic synthesis. Acetals are stable to many reaction conditions, so they can shield an aldehyde or ketone group while other reactions are performed elsewhere in the molecule. Afterwards, acetal hydrolysis easily removes the protecting group, regenerating the original carbonyl compound.