Xenon Valence Electrons: Bonding Explained Simply
Xenon, an element recognized within the periodic table, exhibits unique chemical properties due to its electronic configuration. Specifically, xenon valence electrons, typically considered inert, participate in bonding under certain conditions, defying the traditional octet rule. Linus Pauling's theories on chemical bonding provide a theoretical framework for understanding how xenon, like other noble gases, can form stable compounds such as xenon fluorides. These compounds, often synthesized in specialized laboratories, demonstrate the reactivity of xenon valence electrons, pushing the boundaries of chemical understanding and application.
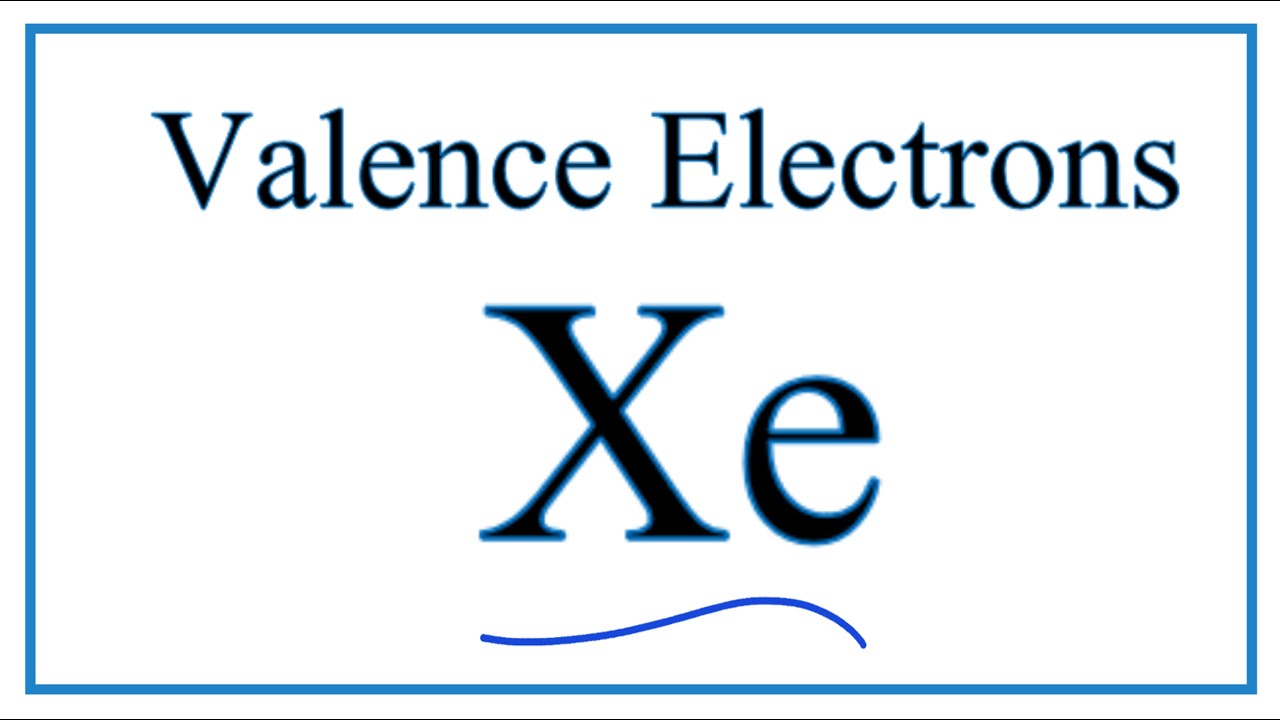
Image taken from the YouTube channel Wayne Breslyn (Dr. B.) , from the video titled How to Find the Valence Electrons for Xenon (Xe) .
Unveiling the Chemistry of Xenon: A Noble Gas No More
Xenon, a name derived from the Greek word "xenos" meaning "stranger," holds a unique position in the realm of chemical elements. For decades, it was considered the quintessential inert gas, a member of Group 18 of the periodic table, also known as the noble gases.
These elements, including helium, neon, argon, krypton, and radon, were believed to be chemically aloof, content with their filled valence electron shells and disinclined to form bonds with other atoms. However, the narrative surrounding xenon took an unexpected turn, revealing a surprisingly rich and reactive chemistry.
Xenon's Place in the Periodic Table
Xenon resides in the fifth period of the periodic table, nestled among the heavier noble gases. Its atomic number is 54, indicating 54 protons in its nucleus and, in a neutral atom, 54 electrons.
The electronic configuration of Xenon is [Kr] 4d10 5s2 5p6. The filled 5s and 5p orbitals are what contribute to its noble gas classification.
However, unlike its lighter counterparts like helium and neon, Xenon possesses a larger atomic radius and a relatively lower ionization energy. This makes it more susceptible to interactions with highly electronegative elements.
The Dawn of Noble Gas Chemistry: Bartlett's Breakthrough
The perception of noble gases as chemically inert was shattered in 1962 by Neil Bartlett. He synthesized the first noble gas compound, xenon hexafluoroplatinate (Xe+[PtF6]-).
This groundbreaking achievement challenged the established chemical dogma and opened the door to a new era of noble gas chemistry.
Bartlett's experiment demonstrated that Xenon could, in fact, participate in chemical bonding. It paved the way for the synthesis of numerous other Xenon compounds. This discovery earned Bartlett widespread recognition and fundamentally altered our understanding of chemical reactivity.
Redefining Chemical Bonding: The Significance of Xenon Compounds
The discovery of Xenon compounds had a profound impact on chemical theory. It forced scientists to reconsider the traditional rules governing chemical bonding.
The existence of these compounds demonstrated that the octet rule, while generally applicable, is not inviolable, especially for heavier elements.
Moreover, the study of Xenon compounds has broadened our understanding of factors influencing chemical reactivity. This includes the role of ionization energy, electronegativity, and orbital interactions.
By studying these seemingly "inert" gas compounds, we gain valuable insights into the nuances of chemical bonding principles. These insights expand our knowledge of chemical behavior across the periodic table.
These compounds highlight the limitations of simplified models and the importance of considering relativistic effects in heavier elements.
Fundamental Concepts: The Building Blocks of Xenon Chemistry
To grasp the intricacies of Xenon's unusual bonding behavior, we must first establish a firm foundation in fundamental chemical concepts. These concepts provide the lens through which we can understand how Xenon, despite its noble gas status, engages in chemical interactions.
This section will delve into valence electrons, the octet rule, chemical bonding types, Lewis structures, and VSEPR theory. These are essential to unraveling the seeming paradox of Xenon's reactivity.
Valence Electrons: The Architects of Chemical Bonds
Valence electrons are the outermost electrons of an atom. They are the primary players in chemical bonding and dictate an element's reactivity.
It's the configuration of valence electrons that determines how an atom interacts with others, whether it forms ionic, covalent, or metallic bonds.
Elements with similar valence electron configurations tend to exhibit similar chemical behavior due to these interactions.
Understanding the number and arrangement of valence electrons is crucial for predicting how an atom will participate in chemical reactions.
The Octet Rule and Noble Gas Stability
The octet rule states that atoms tend to gain, lose, or share electrons to achieve a full complement of eight valence electrons, mirroring the electron configuration of the noble gases.
Noble gases, with their filled valence shells (except for helium, which has two), are exceptionally stable and generally considered chemically inert.
However, Xenon, while a noble gas, presents an exception to this rule, especially under specific conditions such as in the presence of highly electronegative elements.
Its ability to form compounds highlights the limitations of the octet rule. This underscores the nuanced nature of chemical bonding.
Chemical Bonding: The Forces That Unite Atoms
Chemical bonding arises from the attractive forces between atoms, resulting in the formation of molecules and compounds. Several types of bonding exist, each with distinct characteristics.
Ionic bonding involves the transfer of electrons between atoms, creating ions that are held together by electrostatic forces.
Covalent bonding involves the sharing of electrons between atoms to achieve a stable electron configuration.
Coordinate covalent bonding is a special type of covalent bonding where one atom donates both electrons to the shared pair.
Xenon's chemistry involves primarily covalent and coordinate covalent bonds, formed to increase stability by sharing electrons with other atoms.
Lewis Structures: Visualizing Valence Electrons and Bonds
Lewis structures are diagrams that represent the valence electrons of atoms within a molecule or ion. They show how these electrons are arranged and how they participate in chemical bonds.
Dots represent non-bonding electrons (lone pairs), while lines represent shared electron pairs (covalent bonds).
Constructing Lewis structures for Xenon compounds helps visualize the bonding arrangement and electron distribution.
This also reveals whether Xenon violates the octet rule by accommodating more than eight electrons in its valence shell.
Molecular Geometry (VSEPR Theory): Predicting Molecular Shapes
Valence Shell Electron Pair Repulsion (VSEPR) theory predicts the three-dimensional shapes of molecules based on the repulsion between electron pairs surrounding a central atom.
Electron pairs, both bonding and non-bonding, arrange themselves to minimize repulsion, dictating the molecule's geometry.
Applying VSEPR theory to Xenon compounds allows us to predict their shapes. This includes linear, square planar, and distorted octahedral geometries. It also considers the influence of lone pairs on molecular shape.
Understanding molecular geometry is crucial for comprehending a molecule's physical and chemical properties, including its reactivity and polarity.
Reactivity and Bonding Partners: Why Xenon Forms Compounds
To reconcile Xenon's classification as a noble gas with its demonstrated ability to form compounds, we must examine the factors influencing its reactivity and the nature of its bonding partners. While the octet rule suggests inertness, specific conditions and elemental properties permit Xenon to participate in chemical bonding.
The Key to Xenon's Reactivity: Ionization Energy
The ability of Xenon to form compounds hinges on its ionization energy, which is the energy required to remove an electron from a neutral atom in its gaseous phase. Although Xenon possesses a filled valence shell, its ionization energy is significantly lower than that of other noble gases like Helium or Neon.
This relatively lower ionization energy means that, under the right circumstances, Xenon can lose one or more electrons. This allows it to form positive ions and engage in chemical bonding with highly electronegative elements.
The larger atomic size of Xenon, compared to lighter noble gases, also contributes to this effect. As the outermost electrons are further from the nucleus, they are less tightly held.
Electronegativity and Bonding: Common Partners
When Xenon does react, it almost exclusively bonds with elements of high electronegativity. Electronegativity is a measure of an atom's ability to attract electrons in a chemical bond.
The greater the difference in electronegativity between two atoms, the more polar the resulting bond, and the greater the driving force for electron sharing or transfer. The most common bonding partners for Xenon are Fluorine and Oxygen, owing to their exceptionally high electronegativity values.
Fluorine: The Prime Reactant
Fluorine stands out as the most reactive element and a frequent bonding partner for Xenon. Its extremely high electronegativity allows it to readily pull electron density away from Xenon. This forms strong, relatively stable bonds.
The reaction between Xenon and Fluorine, under controlled conditions of temperature and pressure, leads to the formation of a series of fluorides, including Xenon difluoride (XeF2), Xenon tetrafluoride (XeF4), and Xenon hexafluoride (XeF6). These fluorides are the most well-characterized Xenon compounds, due to the strength of the Xenon-Fluorine bond.
Oxygen: Forming Oxides and Oxyfluorides
Oxygen, the second most electronegative element, also forms compounds with Xenon. While not as straightforward as fluoride synthesis, Xenon oxides and oxyfluorides are known.
These compounds, such as Xenon trioxide (XeO3) and Xenon oxytetrafluoride (XeOF4), often require more complex synthetic routes. The Xenon-Oxygen bond, though strong, is generally less stable than the Xenon-Fluorine bond, making these compounds more susceptible to decomposition or explosive behavior.
In essence, the formation of Xenon compounds is a testament to the interplay between ionization energy and electronegativity. While Xenon's noble gas status implies inertness, these factors allow it to engage in chemical bonding with highly electronegative elements, expanding the boundaries of chemical understanding.
Xenon Compounds: A Gallery of Unique Molecules
Having established the fundamental principles that govern Xenon's reactivity, it is now pertinent to explore specific examples of Xenon compounds. These molecules showcase the diverse bonding arrangements and properties that arise when a noble gas defies expectations. This section will detail the synthesis, structure, bonding characteristics, and unique properties of key Xenon compounds, including fluorides, oxides, and the historically significant first noble gas compound.
Xenon Fluorides: A Study in Bonding
Xenon fluorides represent the most well-characterized class of Xenon compounds, owing to the high electronegativity of Fluorine and its propensity to form stable bonds. These compounds, formed under varying conditions of temperature, pressure, and stoichiometric ratios, exhibit a range of geometries dictated by the principles of VSEPR theory.
Xenon Difluoride (XeF2)
Xenon difluoride (XeF2) is synthesized by directly reacting Xenon and Fluorine gas at elevated temperatures or through photochemical methods.
This compound adopts a simple linear structure, with the Xenon atom at the center and two Fluorine atoms positioned on either side.
The bonding in XeF2 can be described using a three-center four-electron bond model, which accounts for the hypervalent nature of Xenon. This model explains how Xenon can accommodate more than eight electrons in its valence shell.
Xenon Tetrafluoride (XeF4)
Xenon tetrafluoride (XeF4) is formed by reacting Xenon and Fluorine in a 1:5 ratio at higher temperatures and pressures than required for XeF2 synthesis.
XeF4 possesses a square planar structure, a geometry readily predicted by VSEPR theory. The Xenon atom is at the center of the square, with four Fluorine atoms occupying the corners. Two lone pairs of electrons reside above and below the plane.
This arrangement minimizes electron repulsion, resulting in a stable, symmetrical molecule.
Xenon Hexafluoride (XeF6)
Xenon hexafluoride (XeF6) is synthesized under more extreme conditions, typically requiring high temperatures and pressures with an excess of Fluorine.
Unlike the other fluorides, XeF6 presents a significant challenge in predicting its molecular geometry. VSEPR theory suggests an octahedral arrangement with a lone pair influencing the shape; however, experimental evidence indicates a distorted octahedral structure.
This distortion arises from the complex interplay of lone pair repulsion and the flexibility of the Xe-F bonds. The precise geometry is still debated, with various models proposing different arrangements to account for the observed properties.
Xenon Oxides and Oxyfluorides: Introducing Oxygen
While Fluorine is the most common bonding partner for Xenon, Oxygen can also participate in compound formation, leading to the creation of Xenon oxides and oxyfluorides. These compounds generally require more complex synthetic routes and often exhibit higher reactivity than the fluorides.
Xenon Oxytetrafluoride (XeOF4)
Xenon oxytetrafluoride (XeOF4) can be synthesized by reacting XeF6 with silica or by partial hydrolysis of XeF6.
XeOF4 adopts a square pyramidal structure, with the Oxygen atom occupying the apical position and four Fluorine atoms forming the base of the pyramid. A lone pair resides on the Xenon atom, influencing the molecular geometry.
This compound is highly reactive and readily hydrolyzes, underscoring the strong affinity of Xenon for Oxygen and Fluorine.
Xenon Trioxide (XeO3)
Xenon trioxide (XeO3) is synthesized by the hydrolysis of XeF6 or XeF4. This process involves dissolving the Xenon fluoride in water, resulting in the formation of XeO3 and hydrofluoric acid.
XeO3 is an extremely dangerous explosive. In its solid state, it detonates spontaneously, even at room temperature, due to its high positive enthalpy of formation and rapid decomposition into Xenon and Oxygen.
The molecule adopts a pyramidal structure, with Xenon at the apex and three Oxygen atoms at the base. The presence of a lone pair on the Xenon atom contributes to its instability and explosive nature.
Xenon Hexafluoroplatinate: A Historic Milestone
The First Noble Gas Compound
Xenon hexafluoroplatinate (Xe+[PtF6]-) holds a unique place in chemical history as the first synthesized noble gas compound.
Neil Bartlett's groundbreaking synthesis of this compound in 1962 revolutionized the understanding of noble gas chemistry.
Bartlett observed the reaction of Platinum hexafluoride (PtF6), a powerful oxidizing agent, with Xenon. This reaction resulted in the formation of a yellow-orange solid, initially believed to be Xe+[PtF6]-.
The exact nature of the product is still debated, with some evidence suggesting a more complex composition. Nevertheless, its synthesis demonstrated that noble gases are not entirely inert and can participate in chemical bonding under specific conditions. The discovery of Xenon hexafluoroplatinate spurred a flurry of research into noble gas chemistry. It led to the synthesis of numerous other Xenon compounds, expanding the horizons of chemical knowledge and challenging long-held beliefs about the inertness of noble gases.
Advanced Concepts: Delving Deeper into Xenon's Electronic Structure
Beyond the basic principles of valence electrons and VSEPR theory, a deeper understanding of Xenon's electronic configuration provides crucial insights into its bonding behavior. By examining the arrangement of electrons within its atomic orbitals, we can better explain why Xenon, despite its noble gas status, participates in chemical bonding.
Unveiling Xenon's Electronic Configuration
Xenon (Xe), with an atomic number of 54, possesses a relatively complex electron configuration: 1s² 2s² 2p⁶ 3s² 3p⁶ 4s² 3d¹⁰ 4p⁶ 5s² 4d¹⁰ 5p⁶. This configuration reveals that Xenon has a filled outermost shell (5s² 5p⁶), which is characteristic of noble gases and typically associated with chemical inertness.
However, the key to Xenon's reactivity lies in the accessibility of its higher energy levels.
While the 5s and 5p orbitals are filled, the 5d orbitals are relatively close in energy.
This proximity allows for the possibility of promoting electrons from the filled 5p orbitals to the empty 5d orbitals, a process that requires energy input but ultimately enables Xenon to form bonds.
The Influence of Electron Configuration on Bonding
The promotion of electrons to the 5d orbitals creates unpaired electrons, which are necessary for covalent bond formation. This process directly influences Xenon's bonding capabilities. The number of electrons promoted dictates the number of bonds Xenon can form, explaining the existence of compounds such as XeF₂, XeF₄, and XeF₆.
Hybridization and Molecular Geometry
The promotion of electrons is usually followed by hybridization, where atomic orbitals mix to form new hybrid orbitals with different shapes and energies.
For example, in XeF₂, Xenon undergoes sp³d hybridization, resulting in five hybrid orbitals arranged in a trigonal bipyramidal geometry.
Two of these orbitals form sigma bonds with Fluorine atoms, while the remaining three contain lone pairs, leading to the observed linear structure.
Similarly, in XeF₄, Xenon undergoes sp³d² hybridization, generating six hybrid orbitals in an octahedral arrangement. Four of these orbitals bond with Fluorine atoms, and the remaining two hold lone pairs, resulting in the square planar geometry.
Energetic Considerations
It's important to note that the formation of Xenon compounds is driven by the energy released during bond formation, which compensates for the energy required to promote electrons. Highly electronegative elements like Fluorine and Oxygen form strong bonds with Xenon, providing the necessary energetic driving force.
The electronic configuration of Xenon, particularly the accessibility of its 5d orbitals, is paramount in understanding its ability to defy the traditional notion of noble gas inertness. By delving into these advanced concepts, we gain a more nuanced appreciation for the complex and fascinating chemistry of Xenon.
Video: Xenon Valence Electrons: Bonding Explained Simply
Frequently Asked Questions
Why is Xenon typically considered an inert gas?
Xenon is considered inert because it has a full outer shell of 8 electrons. These 8 electrons are the xenon valence electrons, meaning it has a stable electron configuration and doesn't readily react with other elements.
How can Xenon form bonds if it's supposed to be inert?
While usually inert, under extreme conditions, Xenon can form compounds. This happens because the xenon valence electrons, though stable, can be forced to participate in bonding with highly electronegative elements like fluorine.
What type of bonding does Xenon typically participate in?
Xenon typically participates in covalent bonding when it forms compounds. This means it shares its xenon valence electrons with another atom, rather than transferring them completely.
What makes Fluorine a common element that bonds with Xenon?
Fluorine is a common element that bonds with xenon due to its extremely high electronegativity. This means fluorine has a strong pull on electrons, strong enough to coax the xenon valence electrons into sharing, forming covalent bonds.
So, there you have it! Hopefully, this clears up some of the mystery surrounding xenon valence electrons and how this noble gas can actually form bonds. It might seem counterintuitive at first, but with a little understanding of electron configurations, it becomes surprisingly straightforward. Now you can impress your friends with your knowledge of xenon valence electrons!