Wave Energy: Harnessing Nature's Power Source
Oceans, vast and dynamic, hold immense potential for renewable energy production; specifically, waves are carriers of energy, a principle that wave energy converters (WECs) are designed to exploit. The European Marine Energy Centre (EMEC), located in the Orkney Islands, serves as a key testing site for these WECs, assessing their ability to transform wave motion into usable electricity. Companies such as Ocean Power Technologies are at the forefront of developing innovative technologies that capture and convert this kinetic energy. Gabriel Lippmann's early work on capturing solar energy indirectly through wave power laid some of the conceptual groundwork for these modern advancements, highlighting the long-standing scientific interest in harnessing the power of the sea.
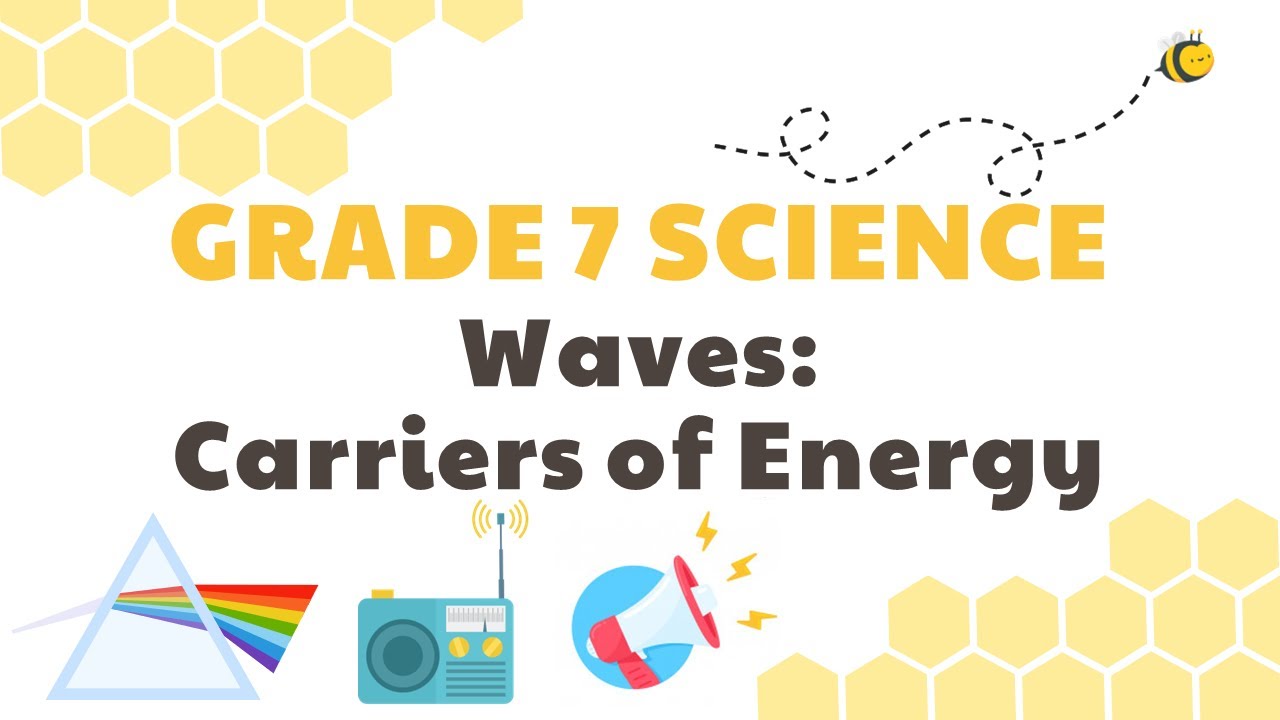
Image taken from the YouTube channel The Learning Bees , from the video titled Waves: Carriers of Energy | Grade 7 Science DepEd MELC Quarter 3 Module 3 .
Unveiling the Untapped Potential of Wave Energy
Wave energy, a burgeoning frontier in renewable energy, holds immense promise for reshaping our global energy landscape. As we grapple with the urgent need to transition away from fossil fuels, wave energy stands out as a compelling, largely untapped resource. Harnessing the power of the ocean's perpetual motion offers a pathway to a cleaner, more sustainable energy future.
Defining Wave Energy and its Vast Potential
Wave energy refers to the energy derived from ocean surface waves. It represents a conversion of the kinetic and potential energy inherent in these waves into usable electricity. The theoretical global wave energy resource is substantial, potentially exceeding the world's current electricity needs.
However, only a tiny fraction of this potential has been realized to date. Technological advancements and strategic investments are crucial to unlock the full potential of this renewable energy source.
Advantages of Wave Energy: Reliability, Predictability, and Energy Density
Wave energy offers several key advantages over other renewable energy sources:
- Reliability: Waves are persistent and less intermittent than solar or wind. Ocean waves are always present.
- Predictability: Wave patterns are relatively predictable, allowing for better grid integration. Wave forecasting is increasingly accurate.
- High Energy Density: Water is denser than air. Wave energy boasts a significantly higher energy density compared to wind or solar. This leads to smaller infrastructure footprints for equivalent power output.
The Global Imperative for Clean Energy
The escalating global demand for clean energy is undeniable. Climate change, air pollution, and energy security concerns are driving a rapid transition towards renewable energy sources.
Wave energy can play a vital role in meeting this demand. It reduces our reliance on fossil fuels. Also, it contributes to a diversified and resilient energy portfolio. By harnessing the ocean's power, we can mitigate the environmental impacts of energy production. We will also create a more sustainable future for generations to come.
Understanding Wave Mechanics: The Physics Behind the Power
To truly harness the potential of wave energy, we must first delve into the fundamental physics that governs wave behavior. This section explores how waves are formed, how they store and transmit energy, and the key principles that influence our ability to extract that energy efficiently. Understanding these mechanics is crucial for designing effective wave energy converters (WECs) and optimizing their performance.
Wave Formation: A Symphony of Forces
Waves are primarily generated by wind transferring energy to the water's surface. The stronger the wind and the longer the distance over which it blows (the fetch), the larger and more powerful the resulting waves.
Tides, caused by the gravitational pull of the moon and sun, also contribute to wave formation, although these are typically characterized by much longer wavelengths and periods than wind-generated waves.
Seismic activity, such as underwater earthquakes and volcanic eruptions, can also generate powerful waves known as tsunamis, which, while carrying immense energy, pose significant challenges for energy extraction due to their unpredictable nature and destructive potential.
Kinetic and Potential Energy: The Duo of Wave Power
Waves possess both kinetic and potential energy. Kinetic energy is associated with the motion of water particles as the wave propagates. As the water particles move in circular or elliptical paths, they carry momentum and contribute to the wave's overall energy.
Potential energy, on the other hand, is related to the wave's height or amplitude. The higher the wave crest, the greater the potential energy stored due to gravity.
The total energy of a wave is the sum of its kinetic and potential energy, and this energy is directly proportional to the square of the wave's amplitude. This relationship underscores the importance of accurately assessing wave height when evaluating the energy potential of a given location.
The Role of Buoyancy in Wave Energy Extraction
Buoyancy, the upward force exerted by a fluid that opposes the weight of an immersed object, plays a vital role in many WEC designs.
Devices that float on the surface of the water, such as point absorbers and oscillating water columns, utilize buoyancy to interact with the waves and extract energy from their motion.
The changing water level caused by passing waves causes these devices to rise and fall, driving mechanical or hydraulic systems that generate electricity.
Fluid Dynamics & Hydrodynamics: Key to Device Optimization
Fluid dynamics and hydrodynamics are essential for understanding how waves interact with WECs.
These disciplines provide the theoretical framework for analyzing wave forces, predicting device motion, and optimizing WEC design to maximize energy capture.
Computational Fluid Dynamics (CFD) software is often used to simulate wave-device interactions and identify potential areas for improvement.
Wave Propagation: How Waves Transfer Energy Over Distance
Wave propagation describes how waves move and transfer energy across the water's surface. As waves travel, they cause water particles to oscillate, but the particles themselves do not travel horizontally with the wave. Instead, the energy is transmitted through the water, allowing waves to travel vast distances with minimal energy loss.
Understanding wave propagation is crucial for predicting wave behavior and designing WECs that can effectively capture energy from incoming waves.
Wave Spectrum: Deciphering Ocean Wave Composition
The wave spectrum describes the distribution of wave energy across different frequencies and wavelengths in a given sea state.
Ocean waves are typically a complex mixture of waves with varying heights, periods, and directions. Analyzing the wave spectrum provides valuable insights into the energy content of the sea and helps engineers design WECs that are optimally tuned to capture the available energy.
Quantifying the Resource: Measuring Wave Characteristics
To truly unlock the immense power of ocean waves, a fundamental understanding of how to quantify this resource is paramount. This involves identifying and measuring the key characteristics that define a wave's energy potential, allowing us to effectively assess wave energy sites and design efficient wave energy converters (WECs). Accurate measurement of these parameters is not merely an academic exercise, but a crucial step towards realizing a wave-powered future.
The Importance of Wave Measurement
Precise wave measurement forms the bedrock of successful wave energy projects. It allows for accurate resource assessment, informing decisions on the suitability of a particular site for wave energy extraction. This data is also critical for the design and optimization of WECs, ensuring that they can effectively capture and convert wave energy into usable electricity.
Furthermore, understanding wave characteristics is essential for predicting the performance and reliability of WECs, enabling efficient grid integration and energy management strategies.
Key Wave Parameters: A Detailed Look
Amplitude: The Wave's Height
Amplitude is defined as the maximum displacement of a wave from its resting position. In simpler terms, it's the height of the wave crest (the highest point) or the depth of the wave trough (the lowest point) relative to the undisturbed water level.
Amplitude is a direct indicator of wave energy. Larger amplitude waves carry significantly more energy. Consequently, regions with consistently high wave amplitudes are highly sought after for wave energy development.
Wavelength: Measuring the Distance
Wavelength is the distance between two corresponding points on consecutive waves, such as the distance between two crests or two troughs.
It's typically measured in meters and is inversely related to wave frequency. Wavelength is an important parameter for WEC design, influencing the size and spacing of devices.
Frequency: The Rate of Oscillation
Frequency represents the number of wave cycles that pass a fixed point in a given amount of time, usually measured in Hertz (Hz), where 1 Hz equals one cycle per second. Wave frequency is related to the wave period, which is the time it takes for one complete wave cycle to pass a fixed point.
Higher frequency waves indicate shorter wave periods and, generally, higher energy transfer rates. Understanding wave frequency is critical for tuning WEC systems to maximize energy capture.
Period: The Time for a Cycle
Period, often denoted by 'T', is defined as the time it takes for one complete wave cycle to pass a fixed point. It is measured in seconds.
The wave period is inversely proportional to the frequency (T = 1/f). Longer periods indicate slower-moving waves, while shorter periods signify faster, more frequent waves. This parameter is crucial in determining the resonance and efficiency of wave energy converters.
Wave Power Density: Quantifying Energy Flux
Wave Power Density (WPD), often measured in kilowatts per meter (kW/m), describes the amount of wave energy that passes through a line of a certain length that is perpendicular to the direction of wave propagation. It is a critical metric used to assess the wave energy potential of a given location.
WPD is calculated based on wave height, wave period, and water density. Areas with high WPD offer the greatest potential for wave energy extraction. Accurately determining WPD is essential for selecting appropriate sites for wave energy farms and estimating the electricity generation potential.
Understanding and accurately measuring wave characteristics is not just a theoretical exercise; it's the foundation upon which the entire wave energy industry is built. By carefully quantifying these parameters, we can unlock the vast potential of ocean waves and pave the way for a cleaner, more sustainable energy future.
Wave Energy Conversion Technologies: Harnessing the Motion
Quantifying the Resource: Measuring Wave Characteristics provides essential data, the next crucial step is understanding how to effectively capture and convert this energy. This is where Wave Energy Converters (WECs) come into play. These innovative devices employ diverse methods to transform the dynamic motion of ocean waves into usable electricity. Let's explore the primary types of WECs and the underlying principles that power them.
Power Take-Off (PTO) Systems: The Heart of Wave Energy Conversion
At the core of every WEC lies the Power Take-Off (PTO) system. The PTO is the mechanism responsible for converting the mechanical energy captured from the waves into electrical energy. The PTO is critical for how effectively the wave's power is transferred.
PTO systems vary widely in design, but their function remains consistent. They act as an intermediary, translating the often irregular and oscillating motion of waves into a smooth, continuous electrical output.
Hydraulic systems, pneumatic systems, and direct-drive generators are common PTO technologies. Each offers distinct advantages in terms of efficiency, reliability, and cost. The selection of a suitable PTO system is paramount to the overall performance and economic viability of a WEC.
Oscillating Water Column (OWC) Technology: Capturing Energy Through Air Compression
Oscillating Water Columns (OWCs) are among the most widely studied and deployed WEC technologies. They operate on the principle of capturing wave energy by using the wave motion to compress air.
An OWC typically consists of a partially submerged, hollow structure with an opening to the sea. As waves enter the chamber, the water level inside rises and falls, forcing air through a turbine.
The turbine, in turn, drives a generator to produce electricity.
OWCs are known for their relative simplicity and robustness, making them suitable for a variety of coastal environments. Both onshore and offshore applications are possible.
Point Absorber Technology: Buoys that Dance with the Waves
Point Absorbers are floating structures that generate electricity from their movement relative to the waves. These devices, often buoy-like in shape, are designed to efficiently absorb energy from all directions.
They typically consist of two main parts: a floating buoy and a submerged structure or a seabed anchor. As waves pass, the buoy moves up and down, driving a PTO system located within the device.
This PTO system converts the relative motion into electricity. Point Absorbers are particularly well-suited for deployment in deep-water locations, far from shore.
Overtopping Device Technology: Wave Reservoirs in the Sea
Overtopping devices mimic the natural process of waves breaking over a coastline. These devices feature a ramp or reservoir that allows incoming waves to surge upwards and overtop the structure.
The water collected in the reservoir is then channeled back to sea through hydro turbines, generating electricity. Overtopping devices can be constructed onshore or offshore, and their energy output is largely dependent on the height and frequency of incoming waves.
The size and design of the reservoir are crucial for optimizing energy capture and conversion.
Attenuator Technology: Riding the Waves for Power
Attenuators are long, floating structures that are oriented parallel to the direction of wave propagation. They flex and bend as waves pass, capturing energy from the relative motion between the segments.
Hydraulic cylinders or other PTO mechanisms are strategically placed between the segments. This converts the mechanical energy into electricity.
Attenuators are particularly effective in areas with consistent wave directionality, as they can efficiently extract energy from the undulating motion of the waves.
Submerged Pressure Differential (SPD) Technology: Harnessing the Deep
Submerged Pressure Differential (SPD) devices operate on the principle of capturing energy from the pressure variations caused by waves passing overhead. These devices are typically deployed on the seabed, where they are less susceptible to extreme weather conditions.
An SPD device consists of a flexible membrane or bladder that is connected to a PTO system. As waves pass, the pressure on the membrane fluctuates, causing it to expand and contract.
This movement drives the PTO system, generating electricity. SPD devices offer the advantage of being visually unobtrusive and having minimal impact on surface navigation.
Key Innovators: Organizations and Companies Pushing the Boundaries
The journey from theoretical potential to practical implementation requires visionaries – organizations and companies willing to invest resources, expertise, and unwavering dedication. Let’s examine some of the key players who are shaping the wave energy landscape.
International Energy Agency (IEA): A Global Catalyst
The International Energy Agency (IEA) plays a pivotal role in promoting international cooperation on energy issues.
Its involvement in wave energy stems from its commitment to diversifying energy sources and mitigating climate change.
The IEA provides valuable data, conducts in-depth analysis, and formulates policy recommendations related to ocean energy technologies.
This includes wave energy, offering guidance to governments and stakeholders on how to best support the sector's growth.
European Marine Energy Centre (EMEC): Real-World Testing and Validation
The European Marine Energy Centre (EMEC), based in Scotland, stands as a world-leading test center for wave and tidal energy technologies.
EMEC provides developers with access to grid-connected, full-scale testing facilities in real-sea conditions, enabling them to validate the performance and reliability of their devices.
This rigorous testing environment is crucial for accelerating the development and commercialization of wave energy technologies.
EMEC's role goes beyond simple testing; it fosters collaboration, shares knowledge, and helps establish industry standards.
Wave Energy Scotland (WES): Innovation and Technology Acceleration
Wave Energy Scotland (WES) is a government-funded organization focused on accelerating the development of wave energy technologies in Scotland.
WES takes a unique approach by funding targeted research and development projects aimed at addressing specific technical challenges.
This ensures that resources are directed towards the areas where they can have the greatest impact, such as improving device performance, reducing costs, and enhancing grid integration.
S. Department of Energy (DOE): Driving Innovation through Funding and Research
The U.S. Department of Energy (DOE) is a key player in advancing wave energy technologies within the United States.
The DOE provides funding for research, development, and demonstration projects.
This supports innovation across a wide range of areas, from fundamental research to the deployment of pilot-scale wave energy devices.
The DOE also supports national laboratories like NREL and PNNL, which conduct cutting-edge research and provide technical expertise to the wave energy industry.
National Renewable Energy Laboratory (NREL): Expertise and Collaboration
The National Renewable Energy Laboratory (NREL) serves as a crucial research hub, offering expertise in wave resource assessment, device modeling, and grid integration.
NREL's contributions extend to developing advanced control systems, optimizing device designs, and assessing the economic viability of wave energy projects.
Through collaborative partnerships with industry, academia, and other research institutions, NREL facilitates the exchange of knowledge and accelerates the pace of innovation.
Pacific Northwest National Laboratory (PNNL): Environmental Considerations and Monitoring
The Pacific Northwest National Laboratory (PNNL) brings a unique perspective to wave energy development by focusing on the potential environmental impacts of these technologies.
PNNL conducts research on the interaction between wave energy devices and marine ecosystems, helping to ensure that wave energy projects are developed in a sustainable and responsible manner.
This helps to minimize any negative impacts on marine life and habitats.
PNNL also develops innovative monitoring technologies to assess the performance and environmental effects of wave energy devices in real-time.
Ocean Power Technologies (OPT): PowerBuoy Pioneers
Ocean Power Technologies (OPT) is a leading company that has been developing its PowerBuoy technology for many years.
The PowerBuoy is a point absorber-type WEC that converts the motion of waves into electricity.
OPT has deployed PowerBuoys in various locations around the world, demonstrating the potential of its technology for powering remote locations, offshore facilities, and coastal communities.
They recently entered into a collaboration with 3Dent B.V. in preparation for the first deployment of a wave energy device in the Dutch North Sea.
Carnegie Clean Energy: CETO Technology and Integrated Solutions
Carnegie Clean Energy, based in Australia, is another prominent player in the wave energy sector.
They are known for their CETO technology, which utilizes submerged buoys to convert wave energy into electricity and desalinated water.
Carnegie focuses on delivering integrated wave energy solutions that can provide both power and freshwater to coastal communities.
CorPower Ocean: High Survivability and Performance
CorPower Ocean, a Swedish company, has developed a unique WEC design based on a wave spring technology.
This design allows their devices to be lightweight, robust, and highly efficient at capturing wave energy.
CorPower's WECs are designed to be highly survivable in harsh ocean conditions, making them suitable for deployment in a wide range of locations.
They are currently undertaking a demonstration project in Portugal.
These organizations and companies represent just a fraction of the innovation occurring within the wave energy sector. Their collective efforts are propelling the technology forward. They contribute to a more sustainable and secure energy future.
Global Hotspots: Locations with High Wave Energy Potential
The development of wave energy is not uniform across the globe. Certain regions, blessed with favorable wave climates and proactive policies, are emerging as hotspots for wave energy innovation and deployment. Let's explore some of these key locations and understand what makes them ideal for harnessing the ocean's power.
Scotland: Riding the Atlantic's Fury
Scotland's north and west coasts, exposed to the full force of the North Atlantic, possess some of the highest wave energy potential in Europe.
Its commitment to renewable energy targets and its strategic investments have positioned it as a leader in the sector.
Wave Energy Scotland (WES), a government-funded body, plays a crucial role in accelerating the development of wave energy technologies through funding calls, collaborative projects, and testing infrastructure.
The European Marine Energy Centre (EMEC) in Orkney provides world-leading testing facilities, allowing developers to deploy and validate their devices in real-sea conditions.
Scotland faces challenges, including grid capacity limitations and the high costs associated with offshore operations, but its ambition and existing infrastructure make it a frontrunner in the global wave energy race.
Oregon, USA: A Pacific Pioneer
Oregon, with its rugged coastline and abundant wave resources, is at the forefront of wave energy development in the United States.
The Pacific Northwest, in general, holds significant promise, but Oregon has been particularly proactive in exploring and supporting wave energy projects.
Oregon State University's Hinsdale Wave Research Laboratory is a significant asset, providing cutting-edge research and testing capabilities.
The planned PacWave South test site will offer developers a pre-permitted location to deploy and test their wave energy converters in open ocean conditions.
Navigating complex regulatory frameworks and securing community support remain key challenges for Oregon's wave energy ambitions, but its strong research base and commitment to sustainability make it a promising location for future deployments.
Coastal Regions with High Wave Energy Resources
Beyond Scotland and Oregon, numerous other coastal regions around the world boast significant wave energy potential:
Western Europe: A Continent of Opportunity
The Atlantic coasts of Portugal, Spain, and France also have substantial wave energy resources. These countries are actively exploring wave energy as part of their broader renewable energy strategies.
Australia and New Zealand: Southern Hemisphere Powerhouses
The southern coasts of Australia and New Zealand are exposed to powerful swells generated in the Southern Ocean. This creates a potentially significant resource.
These regions are attracting increasing interest for wave energy development.
South Africa: An Emerging Market
South Africa's long coastline also presents opportunities for wave energy. However, development is in the early stages compared to other regions.
Chile: Latin American Potential
The Chilean coast, bordering the Pacific Ocean, experiences consistent and energetic wave conditions, making it a promising region for harnessing wave energy.
The success of wave energy development in these global hotspots depends not only on the wave resource itself, but also on supportive government policies, robust grid infrastructure, and community acceptance. By overcoming the existing challenges and fully investing in R&D, we can accelerate the shift to a cleaner, wave-powered energy future.
Tools and Technologies for Wave Energy Development: Modeling, Testing, and Grid Integration
The path to harnessing the vast potential of wave energy is paved with innovative tools and technologies. From sophisticated computer simulations to rigorous physical testing and seamless grid integration, a multi-faceted approach is crucial for realizing successful wave energy projects. Let's explore the key elements that drive progress in this exciting field.
Computational Fluid Dynamics (CFD) in Wave Energy
CFD software has become an indispensable tool in the design and optimization of Wave Energy Converters (WECs). By simulating the complex interaction between waves and WECs, CFD allows engineers to:
- Predict performance under various wave conditions.
- Identify areas of stress and potential failure.
- Optimize the shape and configuration of WECs for maximum energy capture.
Advantages of CFD Modeling
CFD offers a cost-effective and time-efficient alternative to extensive physical testing. Through high-fidelity simulations, engineers can explore a wide range of design options and operating scenarios without the need for expensive prototypes.
This iterative process allows for rapid refinement of WEC designs, leading to improved performance and reduced development costs. By accurately modelling the hydrodynamic behaviour of the wave energy devices, we improve the efficiency of the development cycle.
Types of CFD Models Used
Different CFD models are suitable for different aspects of wave energy development. Some commonly used models include:
- Reynolds-Averaged Navier-Stokes (RANS): Provides a good balance between accuracy and computational cost for simulating turbulent flows.
- Large Eddy Simulation (LES): Captures more of the turbulent flow structures, leading to higher accuracy but also higher computational demands.
- Smoothed Particle Hydrodynamics (SPH): A mesh-free method that is well-suited for simulating highly complex wave phenomena, such as breaking waves.
Wave Tanks: Validating Designs in a Controlled Environment
While CFD provides valuable insights, physical testing remains essential for validating WEC designs and assessing their performance in realistic wave conditions. Wave tanks offer a controlled environment for conducting these tests, allowing engineers to precisely control wave parameters and measure the response of WECs.
Types of Wave Tanks
Several types of wave tanks exist, each designed to simulate different wave conditions:
- 2D Wave Flumes: Long, narrow tanks that generate unidirectional waves. Ideal for testing the basic hydrodynamic performance of WECs.
- 3D Wave Basins: Larger tanks that can generate multi-directional waves, simulating more realistic ocean conditions. Essential for testing the stability and survivability of WECs.
Instrumentation and Data Acquisition
Wave tanks are equipped with sophisticated instrumentation to measure wave characteristics, WEC motion, and power output. These instruments may include:
- Wave probes: Measure wave height and period.
- Load cells: Measure forces and moments on the WEC.
- Motion capture systems: Track the position and orientation of the WEC.
- Power meters: Measure the electrical power generated by the WEC.
The data collected from wave tank tests is used to validate CFD models, refine WEC designs, and assess the overall performance of wave energy devices.
Power Electronics: Bridging the Gap to the Grid
Once wave energy is captured and converted into mechanical energy, power electronics play a crucial role in converting it into a form that can be integrated into the electrical grid. This involves converting the variable voltage and frequency output of WECs into a stable, grid-compatible AC voltage.
Key Functions of Power Electronics
Power electronics systems for wave energy applications typically perform the following functions:
- Rectification: Converts AC power from the WEC into DC power.
- DC-DC Conversion: Regulates the DC voltage to match the input requirements of the inverter.
- Inversion: Converts DC power into AC power with the correct voltage and frequency for grid connection.
- Grid Synchronization: Ensures that the AC power from the WEC is synchronized with the grid voltage and frequency.
Advanced Power Electronics Technologies
Emerging power electronics technologies are further enhancing the efficiency and reliability of wave energy systems. These include advanced control algorithms, wide-bandgap semiconductors (e.g., silicon carbide and gallium nitride), and modular power converter designs.
-
Advanced control algorithms optimize the operation of the power electronics system, maximizing power output and improving grid stability.
-
Wide-bandgap semiconductors offer higher efficiency, higher switching frequencies, and better thermal performance compared to traditional silicon-based devices.
-
Modular power converter designs allow for scalability and redundancy, improving the overall reliability of the wave energy system.
By mastering these tools and technologies – CFD modeling, wave tank testing, and advanced power electronics – we can unlock the full potential of wave energy and pave the way for a sustainable energy future.
Video: Wave Energy: Harnessing Nature's Power Source
Frequently Asked Questions About Wave Energy
How does wave energy technology work?
Wave energy converters capture the power of ocean waves and transform it into electricity. Various technologies exist, but most use the motion of the waves to drive turbines or pumps. These mechanisms then generate electricity that can be transmitted to the shore. Waves are carriers of energy.
What are the main advantages of wave energy?
Wave energy is a renewable resource and offers a predictable energy source compared to wind or solar power. It also has a smaller environmental footprint than fossil fuels and contributes to reducing carbon emissions. Waves are carriers of energy.
Where can wave energy be practically implemented?
Wave energy is most viable in coastal regions with consistently strong wave activity. Suitable locations often include the western coasts of continents and islands exposed to open ocean waves. Feasibility studies are crucial to determine the best placement for wave energy farms. Waves are carriers of energy.
What are some challenges in developing wave energy technology?
Challenges include the high initial costs of construction and maintenance, the need for durable technologies that can withstand harsh marine environments, and the potential impacts on marine ecosystems. Efficiency improvements and cost reductions are crucial for wider adoption. Though, waves are carriers of energy.
So, the next time you're at the beach watching the waves roll in, remember they're not just a pretty sight – waves are carriers of energy, and we're getting closer to tapping into that incredible, sustainable power source to light up our lives. Exciting stuff, right?