Unlock the Atom: Decoding the Charge of an Electron!
The National Institute of Standards and Technology (NIST) establishes fundamental constants, and the charge of an electron, a core property, is crucial for accurate calculations. Understanding this minuscule charge of an electron involves employing tools like the Millikan oil drop experiment. The Standard Model of particle physics provides a theoretical framework, defining the charge of an electron as a fundamental unit of negative charge. Scientists like Robert Millikan dedicated their work to accurately measuring the charge of an electron, revolutionizing our understanding of atomic structure.
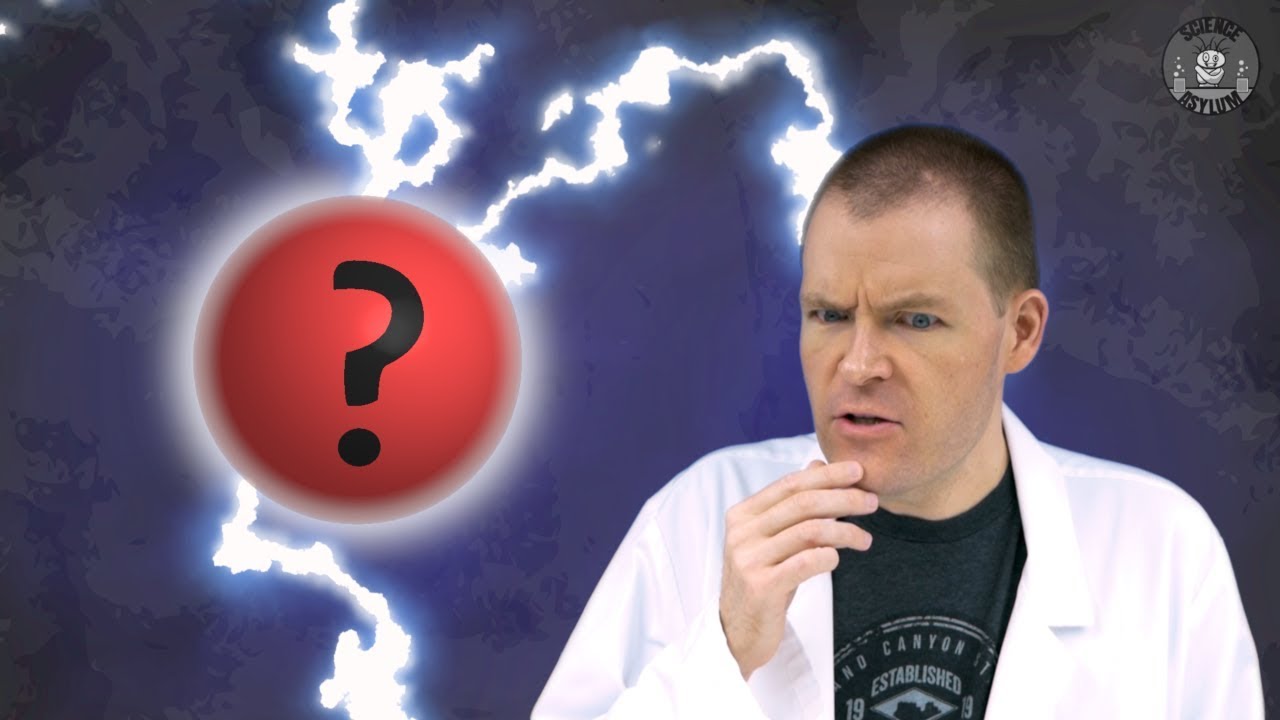
Image taken from the YouTube channel The Science Asylum , from the video titled What is Electric Charge? (Electrodynamics) .
The electron, an almost ethereal speck of matter, is the cornerstone of our understanding of the physical world. This seemingly simple particle, residing within the atom, dictates the behavior of matter at its most fundamental level. Its influence stretches from the smallest chemical reactions to the grandest cosmic events.
The Electron: A Fundamental Building Block
At the heart of every atom lies the electron, orbiting the nucleus and defining the atom's chemical properties. Electrons are fundamental particles, meaning they are not composed of anything smaller. They are the quintessential units of negative charge, and their interactions govern how atoms bond to form molecules and materials. Without electrons, matter as we know it could not exist.
The Charge Conundrum: Why It Matters
Understanding the electron's electric charge is not merely an academic exercise. It is a critical key that unlocks the secrets of countless scientific disciplines. From the intricate workings of semiconductors in our computers to the complex interactions within living cells, the electron's charge plays a pivotal role.
The properties of materials, the nature of chemical bonds, and the flow of electricity – all are ultimately determined by the behavior of electrons and their inherent charge. A precise knowledge of this fundamental property is therefore essential for technological advancements and scientific breakthroughs.
Exploring the Electron's Charge: A Journey of Discovery
This article embarks on a journey to explore the multifaceted nature of the electron's charge. We will delve into its discovery, measurement, and significance, uncovering the vital role it plays in shaping our understanding of the universe. We will discuss its impact across various scientific fields, illustrating why the seemingly minuscule charge of an electron holds such immense importance.
Demystifying Electric Charge: A Fundamental Force
Having established the electron's central role, it's time to delve into the very essence of what makes it so influential: its electric charge. Electric charge isn't just a property; it's a fundamental force that shapes the universe at its most basic level.
What is Electric Charge?
Electric charge is an intrinsic property of matter that causes it to experience a force when placed in an electromagnetic field. It is a fundamental property, like mass or spin, and it dictates how particles interact with each other through the electromagnetic force.
Think of it as the "source" of electromagnetism. Without electric charge, there would be no electromagnetic force, and the universe would be a very different place.
Positive and Negative: The Duality of Charge
Electric charge comes in two varieties: positive and negative. These aren't just arbitrary labels; they represent fundamentally different behaviors. Objects with the same type of charge repel each other, while objects with opposite charges attract.
This attraction and repulsion is what drives the formation of atoms, molecules, and all the structures we see around us.
The conventional assignment of positive and negative charge stems from historical convention, but its impact is profound. It allows us to understand and predict the behavior of charged particles in electric and magnetic fields.
The Elementary Charge: The Quantum of Electricity
The elementary charge (denoted by e) is the smallest unit of electric charge that can exist freely. It is the magnitude of the electric charge carried by a single proton or electron.
All observable charges are integer multiples of this fundamental unit. This is a cornerstone of our understanding of electricity, demonstrating that charge is quantized, meaning it comes in discrete packets rather than a continuous range.
The concept of the elementary charge is essential for understanding the structure of matter at the atomic and subatomic levels. It explains why we never observe fractions of an electron's charge in isolation.
Therefore, comprehending electric charge, its positive/negative duality, and the elementary charge is critical for unraveling more complex phenomena. These are the building blocks upon which our understanding of electricity and electromagnetism is built.
Having explored the fundamental nature of electric charge and its presence in both positive and negative forms, we now turn our attention to the groundbreaking journey that led to the discovery of the electron itself. This wasn't an overnight revelation but rather the culmination of decades of scientific curiosity, ingenious experimentation, and a gradual shift in our understanding of the building blocks of matter.
A Glimpse into the Past: Unveiling the Electron's Discovery
The story of the electron begins long before the particle was definitively identified. It's a narrative woven with the threads of 19th-century physics, a time when scientists were beginning to probe the mysteries of electricity and matter with increasing precision.
The Cathode Ray Tube: A Window into the Subatomic World
Central to the discovery of the electron was the Cathode Ray Tube (CRT). This seemingly simple device, a glass tube with electrodes at either end, became a crucial tool for exploring the nature of electricity.
When a high voltage was applied across the electrodes, a stream of particles, dubbed cathode rays, was emitted from the negatively charged electrode (the cathode).
These rays traveled towards the positively charged electrode (the anode), causing the glass at the end of the tube to glow.
Early experiments with CRTs revealed that these rays could be deflected by magnetic fields, suggesting that they were composed of charged particles.
However, the exact nature of these particles remained a mystery. Were they waves, particles, or something else entirely?
J. Thomson: The Pioneer Who Identified the Electron
The scientist who ultimately unlocked the secret of the cathode rays was J.J. Thomson, a British physicist working at the Cavendish Laboratory in Cambridge.
Through a series of ingenious experiments in 1897, Thomson demonstrated conclusively that cathode rays were, in fact, streams of negatively charged particles, each with a fixed mass.
Thomson's experiments involved carefully measuring the deflection of cathode rays in both electric and magnetic fields.
By analyzing these deflections, he was able to determine the charge-to-mass ratio of the particles.
This ratio turned out to be remarkably consistent, regardless of the material used to construct the cathode.
This suggested that these particles were a universal constituent of matter, not just a byproduct of the specific experimental setup.
Thomson initially called these particles "corpuscles," but they would later be known as electrons.
Redefining Matter: The Emergence of Subatomic Particles
Thomson's discovery had profound implications for our understanding of matter.
Before his work, atoms were considered to be the smallest, indivisible units of matter.
Thomson's experiments demonstrated that atoms were, in fact, composed of even smaller subatomic particles.
The discovery of the electron shattered the long-held belief in the indivisibility of the atom and ushered in a new era of particle physics.
The electron became the first subatomic particle to be identified, paving the way for the discovery of protons, neutrons, and a whole zoo of other elementary particles in the decades that followed.
Unveiling the Negative Charge of the Electron
One of the key properties of the electron, as revealed by Thomson's experiments, was its negative charge.
The deflection of cathode rays in electric and magnetic fields clearly indicated that the particles were repelled by negative charges and attracted to positive charges.
This inherent negative charge is a fundamental characteristic of the electron, and it plays a crucial role in its interactions with other particles and in the formation of atoms and molecules.
The negative charge of the electron is balanced by the positive charge of the protons in the atom's nucleus, resulting in electrically neutral atoms.
The discovery of the electron's negative charge not only unveiled a fundamental property of the universe, but also gave rise to new technologies we now use today.
Having painstakingly traced the electron's initial identification, the scientific community still faced a crucial question: what exactly was the magnitude of this fundamental charge? It was one thing to know that electrons carried a negative charge, but quite another to precisely quantify that charge. This challenge was met head-on by Robert Millikan, whose ingenious experiment provided the definitive measurement that cemented our understanding of the electron.
Millikan's Triumph: Quantifying the Elusive Charge
Robert Millikan's Oil Drop Experiment stands as a monumental achievement in the history of physics, a testament to scientific ingenuity and meticulous execution. This section explores the historical context, methodology, and lasting impact of Millikan's work, which provided the first accurate measurement of the elementary charge, e.
The Scientific Landscape Before Millikan
At the turn of the 20th century, the existence of the electron had been confirmed, but its charge remained a mystery. Scientists knew that cathode rays were composed of negatively charged particles, and J.J. Thomson had even estimated the charge-to-mass ratio of these particles.
However, determining the absolute value of the electron's charge proved to be a formidable challenge. Various researchers attempted to measure this fundamental constant, but their results were often inconsistent and unreliable.
It was in this context that Robert Millikan embarked on his groundbreaking experiment, driven by a desire to provide a definitive answer to this fundamental question.
The Genius of the Oil Drop Experiment
The Millikan Oil Drop Experiment, conducted between 1909 and 1913, was deceptively simple in its concept, yet remarkably sophisticated in its execution.
Experimental Setup and Procedure
The experiment involved observing tiny oil droplets suspended between two parallel metal plates. These droplets were sprayed into the chamber and allowed to fall under the influence of gravity.
Millikan used X-rays to ionize the air within the chamber, causing the oil droplets to pick up one or more electrons, thus acquiring a negative charge.
By applying a voltage across the plates, Millikan could create an electric field that opposed the force of gravity.
By carefully adjusting the voltage, he could suspend individual droplets in mid-air or even cause them to move upwards against gravity.
Measuring the Elementary Charge
The key to Millikan's experiment lay in his ability to precisely measure the forces acting on the oil droplets. By equating the electric force with the gravitational force, he could determine the charge on each droplet.
Crucially, Millikan found that the charge on each droplet was always a multiple of a fundamental unit of charge. This led him to conclude that this fundamental unit was the charge of a single electron, the elementary charge (e).
Through meticulous measurements and careful analysis, Millikan determined the value of e to be approximately 1.5924 × 10−19 coulombs, remarkably close to the currently accepted value.
The Enduring Impact of Millikan's Discovery
Millikan's Oil Drop Experiment had a profound impact on our understanding of the electron and the nature of electric charge.
Confirmation of Charge Quantization
One of the most significant results of Millikan's experiment was the confirmation that electric charge is quantized. This means that charge exists only in discrete units, multiples of the elementary charge e.
This discovery was a major step forward in our understanding of the fundamental nature of matter and paved the way for the development of quantum mechanics.
Accurate Determination of the Elementary Charge
Millikan's experiment provided the first accurate measurement of the elementary charge, a fundamental constant of nature. This value is essential for many calculations in physics and chemistry, and it continues to be used by scientists around the world.
A Legacy of Precision and Ingenuity
The Millikan Oil Drop Experiment is not only a scientific triumph but also a testament to the power of careful experimentation and meticulous data analysis. Millikan's work serves as an inspiration to scientists and a reminder of the importance of precision in scientific measurements.
Millikan's oil drop experiment provided a groundbreaking method for determining the fundamental unit of electrical charge, but to truly understand its significance, we must delve into the precise numerical value and units used to express this quantity. This translation of experimental results into a standardized language of measurement is crucial for consistent scientific communication and application.
The Language of Charge: Numerical Value and Units
The Coulomb and the Electron's Charge
The standard unit of electric charge in the International System of Units (SI) is the Coulomb (C). It's a derived unit, defined in terms of other base units like the ampere (unit of electric current) and the second (unit of time).
Through Millikan's experiment and subsequent refinements, the accepted numerical value for the charge of a single electron is approximately -1.602176634 × 10⁻¹⁹ Coulombs.
The negative sign signifies that the electron carries a negative charge, as established by earlier experiments with cathode rays. This incredibly small value underscores the subatomic nature of the electron and the vast number of electrons required to produce even a modest amount of macroscopic charge.
Elementary Charge and its Relation to the Coulomb
The concept of the elementary charge (e) represents the magnitude of the electric charge carried by a single proton or electron. It's a fundamental constant of nature.
While the electron's charge is negative, the elementary charge is typically referred to as its absolute value, 1.602176634 × 10⁻¹⁹ C.
This means that one Coulomb is equivalent to the charge of approximately 6.241509074 × 10¹⁸ elementary charges.
Understanding this relationship is vital for converting between the microscopic world of individual particles and the macroscopic world of measurable currents and charges.
The Importance of SI Units
The use of SI units is paramount in scientific research and technological development. These standardized units provide a common language for scientists and engineers worldwide, ensuring consistency and comparability of measurements.
Without a universally accepted system of units, replicating experiments, sharing data, and collaborating across disciplines would be nearly impossible. The Coulomb, as an SI unit, plays a vital role in maintaining this global standard.
The Electron Volt: An Energy Unit for the Quantum Realm
While the Coulomb measures charge, the electron volt (eV) is a unit of energy particularly relevant in atomic and particle physics. One electron volt is defined as the amount of energy gained (or lost) by a single electron when it moves through an electric potential difference of one volt.
The relationship between electron volts and Joules (the SI unit of energy) is: 1 eV = 1.602176634 × 10⁻¹⁹ Joules.
The electron volt is a convenient unit for expressing the energies of atomic and subatomic particles because it avoids the use of extremely small numbers that would be required if Joules were used directly. It also provides a direct link between energy and electrical potential, simplifying calculations in many areas of physics.
The Ripple Effect: Significance Across Physics and Beyond
Having established the numerical value and units for the electron's charge, it's time to explore the profound implications of this seemingly minuscule quantity. The significance of the electron's charge extends far beyond the confines of the laboratory, permeating various branches of physics and shaping our understanding of the world around us.
Electron Charge in Fundamental Physics
The electron's charge plays a pivotal role in two cornerstone areas of physics: electromagnetism and quantum mechanics.
In electromagnetism, the electron's charge is the linchpin for understanding the interactions between electric and magnetic fields. These interactions are responsible for a vast array of phenomena, from the operation of electric motors and generators to the propagation of light.
The force experienced by a charged particle in an electric or magnetic field is directly proportional to its charge, making the electron's charge a fundamental parameter in calculating these forces.
Quantum Choreography: Electrons in Quantum Mechanics
In quantum mechanics, the electron's charge dictates its behavior at the atomic and subatomic levels. The Schrödinger equation, a cornerstone of quantum mechanics, incorporates the electron's charge to predict its energy levels and probability distributions within an atom.
This understanding is critical for explaining the structure of atoms, their interactions with light, and the formation of chemical bonds. The quantization of energy levels, a direct consequence of quantum mechanics, is intimately linked to the electron's charge and its interaction with the atomic nucleus.
Beyond Physics: Electron Charge in Other Disciplines
The influence of the electron's charge extends far beyond the realm of physics, playing a crucial role in other scientific disciplines, most notably chemistry, materials science, and electronics.
Chemical Reactions: The Dance of Electrons
In chemistry, the electron's charge is the key to understanding chemical bonding and reactions. Atoms form bonds by sharing or transferring electrons to achieve a stable electron configuration. The strength and nature of these bonds are directly related to the electron's charge and its distribution within the molecule.
Chemical reactions involve the rearrangement of electrons between atoms, leading to the formation of new molecules. Understanding the electron's charge and its role in chemical bonding is essential for predicting the outcome and kinetics of chemical reactions.
Materials Science: Properties from Electron Interactions
In materials science, the electron's charge determines the electrical, optical, and magnetic properties of materials. The movement of electrons through a material gives rise to electrical conductivity, while the interaction of electrons with light determines its color and transparency.
The magnetic properties of materials arise from the intrinsic angular momentum of electrons, known as spin, which is also associated with a magnetic dipole moment proportional to the electron's charge.
Electronics: Harnessing the Electron's Flow
In electronics, the electron's charge is the foundation upon which all electronic devices are built. Transistors, diodes, and integrated circuits rely on the controlled flow of electrons to perform their functions.
The ability to manipulate and control the electron's charge is essential for creating electronic devices that can amplify signals, switch currents, and store information. The development of new electronic technologies is often driven by advances in our understanding of the electron's charge and its behavior in different materials.
The Foundation of Matter: Atoms and Molecules
Ultimately, the electron's charge is fundamental to the behavior of atoms and molecules, underpinning all chemical reactions and the properties of materials. It is the glue that holds atoms together, allowing them to form molecules and create the diverse array of substances that make up our world.
Without the electron's charge, matter as we know it would not exist. The intricate dance of electrons, governed by the laws of quantum mechanics and electromagnetism, is the foundation upon which all of chemistry and materials science are built. It is a testament to the power of a single fundamental constant to shape the structure and behavior of the universe.
Having seen the electron's charge at work across diverse scientific disciplines, from electromagnetism to quantum mechanics, it's natural to wonder about the frontiers of research. How is our understanding of this fundamental property evolving, and what new technological marvels are being born from this knowledge?
The Cutting Edge: Modern Research and Technological Applications
The study of the electron and its charge is far from a closed book. Contemporary research continues to probe the electron's properties with ever-increasing precision, seeking to refine our understanding and uncover new phenomena. This pursuit not only deepens our grasp of fundamental physics but also fuels innovation in a wide range of technologies.
Exploring the Electron's Intrinsic Properties
Scientists are actively engaged in experiments designed to test the Standard Model of particle physics, which predicts the electron's properties with remarkable accuracy. Deviations from these predictions could hint at new physics beyond our current understanding.
High-precision measurements of the electron's magnetic dipole moment, for instance, provide stringent tests of the Standard Model and search for potential new particles or interactions. Researchers are also exploring the possibility that the electron may have a non-zero electric dipole moment, which would violate time-reversal symmetry and have profound implications for our understanding of the universe.
Furthermore, the electron’s charge is being examined within exotic materials and under extreme conditions to observe novel quantum phenomena. This research has implications for areas like quantum computing and high-energy physics.
Nanotechnology and Single-Electron Devices
The precise control and manipulation of individual electrons are at the heart of many emerging technologies. Nanotechnology, in particular, relies heavily on our understanding of the electron's charge to create nanoscale devices with unprecedented functionalities.
Single-electron transistors (SETs), for example, exploit the discrete nature of the electron's charge to achieve extremely low power consumption and high sensitivity. These devices hold promise for applications in sensors, memory devices, and quantum computing.
Furthermore, researchers are developing novel materials and structures that allow for the precise control of electron transport at the nanoscale. This includes exploring the use of quantum dots, nanowires, and two-dimensional materials like graphene to create new electronic devices with tailored properties.
Advanced Materials and Energy Applications
The behavior of electrons within materials is crucial for determining their electrical, optical, and magnetic properties. A deeper understanding of the electron's charge and its interactions within materials is enabling the design of new materials with enhanced performance for a variety of applications.
For example, researchers are developing new battery technologies that rely on the efficient transport of electrons and ions within the battery materials. Similarly, the development of more efficient solar cells hinges on our ability to control the flow of electrons generated by sunlight.
The field of spintronics, which exploits the electron's spin in addition to its charge, is also gaining momentum. Spintronic devices have the potential to be faster, smaller, and more energy-efficient than traditional electronic devices. Advances in understanding and controlling the electron’s properties offer vast potential across multiple industries.
Video: Unlock the Atom: Decoding the Charge of an Electron!
Frequently Asked Questions: Decoding the Charge of an Electron
These FAQs will help clarify the concept of the charge of an electron and its significance.
What exactly is electric charge?
Electric charge is a fundamental property of matter that causes it to experience a force when placed in an electromagnetic field. It can be either positive or negative. The charge of an electron is negative.
How was the charge of an electron discovered?
Robert Millikan's oil drop experiment in the early 20th century was pivotal. By carefully observing the movement of charged oil droplets in an electric field, he was able to accurately determine the fundamental unit of electric charge, revealing the charge of an electron.
Why is understanding the charge of an electron important?
The charge of an electron governs how atoms interact with each other. This interaction underlies all chemical bonding and reactions. Without understanding the charge of an electron, we couldn't explain the behavior of matter.
What is the current accepted value for the charge of an electron?
The accepted value for the elementary charge, which is the magnitude of the charge of an electron, is approximately -1.602 × 10^-19 coulombs. This tiny value is fundamental to many calculations in physics and chemistry.
So, there you have it! Hopefully, you now have a better grasp on the charge of an electron. Keep exploring, and remember, even the tiniest things like the charge of an electron can unlock huge discoveries!