Thylakoids Revealed! The Secret To Plant's Success?
Photosynthesis, the engine of nearly all life on Earth, relies heavily on specialized structures within plant cells. Chloroplasts, the organelles responsible for this vital process, contain stacks of thylakoids, often referred to as grana. These grana, essential for the light-dependent reactions, increase the surface area for light absorption. Professor Melvin Calvin, known for his groundbreaking work on the Calvin cycle, demonstrated the role of these light-dependent reactions in capturing energy. The efficiency of these stacks of thylakoids affects the overall productivity of ecosystems from sprawling agricultural lands to dense rainforests.
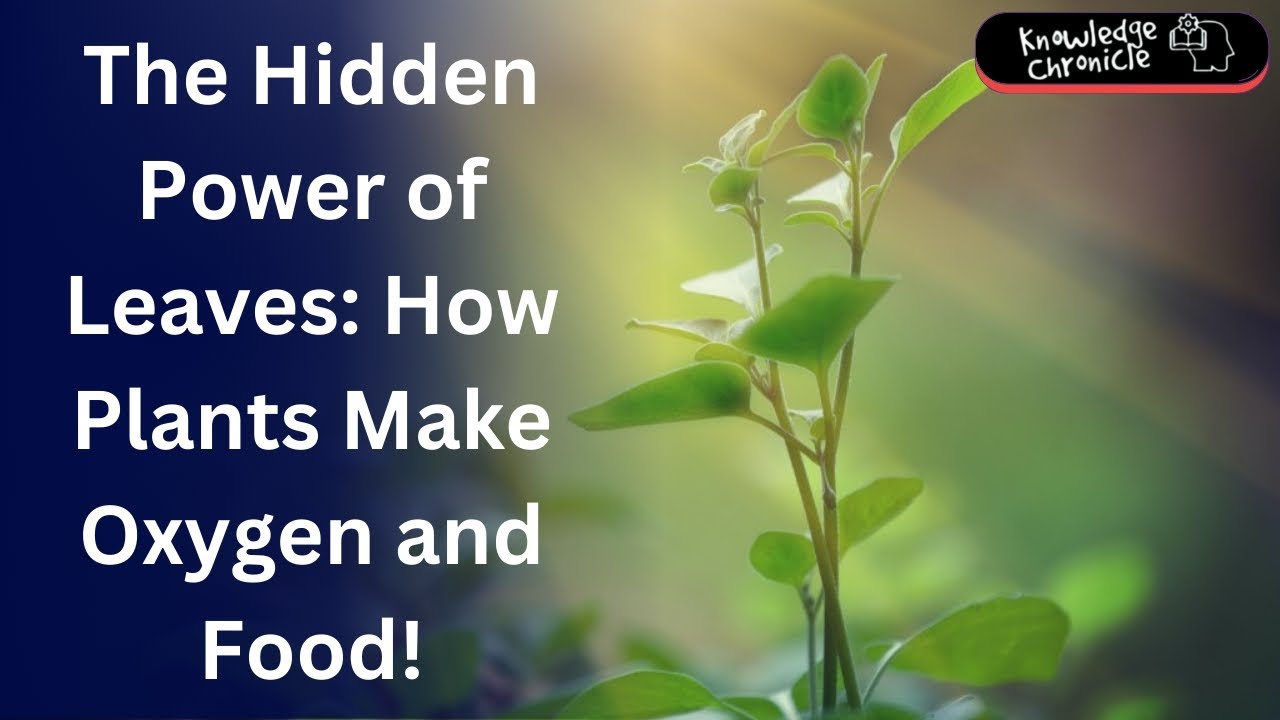
Image taken from the YouTube channel Knowledge Chronicle , from the video titled Photosynthesis Uncovered: The Secret Power of Plants. .
The Green Engine of Life: Unveiling Photosynthesis' Secret
Imagine a lush, vibrant forest, a field of swaying wheat, or even the humble houseplant on your windowsill. What unites these diverse forms of plant life?
The answer lies in a single, fundamental process: photosynthesis. It's the engine that drives nearly all life on Earth, converting light energy into the chemical energy that fuels plant growth and sustains ecosystems.
At the heart of this process lie tiny, yet incredibly important structures called thylakoids.
Thylakoids: The Architects of Photosynthesis
Within the cells of plants, particularly in the organelles known as chloroplasts, thylakoids are the key players. These internal membrane-bound compartments are where the magic of photosynthesis truly happens.
Think of them as miniature solar panels, expertly designed to capture and convert sunlight.
But what exactly are thylakoids, and why are they so crucial?
This article delves into the intricate world of these structures, exploring their form, function, and overall significance in plant life. We will focus particularly on how thylakoids stack together to form grana.
Exploring the Grana: Stacks of Efficiency
Grana are critical components within chloroplasts, working to optimize photosynthetic function. They maximize light absorption and facilitate the complex biochemical reactions that underpin plant life.
Unraveling the mysteries of thylakoids and grana is essential for understanding the very essence of plant biology.
This exploration offers potential avenues for improving sustainable energy solutions, and potentially even unlocking new ways to mimic nature's efficiency.
Thesis: The Critical Role of Thylakoids
This article explores the structure, function, and significance of thylakoids, particularly the "stacks of thylakoids" (grana) within chloroplasts, revealing their critical role in plant life.
Chloroplasts: The Cellular Home of Thylakoids
Photosynthesis, the process we've introduced as the cornerstone of plant life, doesn't happen in isolation. It unfolds within specialized compartments inside plant cells called chloroplasts. Understanding the structure of these organelles is crucial to appreciating the context in which thylakoids operate. Think of the chloroplast as the factory, and the thylakoids as the specific assembly lines dedicated to capturing light energy.
Chloroplasts Defined: Powerhouses of Photosynthesis
Chloroplasts are organelles, membrane-bound structures within plant cells (and algae), that are exclusively responsible for carrying out photosynthesis.
They are not merely passive containers; they are dynamic and highly organized structures, optimized for the complex biochemical reactions that convert light energy into chemical energy in the form of sugars.
Anatomy of a Chloroplast: A Multi-Layered Structure
The chloroplast's structure is key to its function. It's composed of several distinct components. Understanding these components is crucial to fully appreciate the location of thylakoids.
Let's examine the key features:
-
Outer Membrane: The outermost boundary of the chloroplast, relatively permeable to small molecules.
-
Inner Membrane: Located inside the outer membrane, the inner membrane is highly selective, regulating the passage of substances into and out of the chloroplast. The space between the outer and inner membranes is the intermembrane space.
-
Stroma: The fluid-filled space within the inner membrane. The stroma contains enzymes, DNA, and ribosomes necessary for various metabolic processes, including the Calvin cycle, where carbon dioxide is fixed and sugars are synthesized.
Thylakoids: Inhabitants of the Stroma
Crucially, suspended within the stroma are the thylakoids. These are internal, membrane-bound compartments that form a complex network within the chloroplast.
Think of thylakoids as flattened sacs or discs. They are often arranged in stacks, like pancakes, which we call grana (singular: granum).
These grana are interconnected by stroma lamellae, which are essentially thylakoids that extend through the stroma. This interconnected network maximizes the efficiency of photosynthesis.
Crucially, suspended within the stroma are the thylakoids – the very structures at the heart of photosynthesis. But what exactly are thylakoids, and how does their unique architecture contribute to their pivotal role in capturing light energy?
Deconstructing Thylakoid Structure: Form and Function
The intricate process of photosynthesis hinges on the specialized structure of thylakoids. These internal compartments, nestled within the chloroplast, are not simply amorphous sacs; their distinct form is inextricably linked to their function.
The Disc-Shaped Design: Maximizing Surface Area
An individual thylakoid resembles a flattened, disc-shaped sac. This morphology is no accident. The large surface area afforded by this shape is critical. It ensures maximal exposure to the stroma, and thus, to the incoming light.
This expanded surface is densely packed with photosynthetic pigments and proteins. They all work in concert to capture and convert light energy. Think of each thylakoid as a solar panel, meticulously designed for optimal energy absorption.
Grana: Stacks of Efficiency
Individual thylakoids rarely exist in isolation. Instead, they are typically stacked upon one another. This resembles neat piles of pancakes. These stacks are called grana (singular: granum).
The formation of grana represents a crucial adaptation. It further amplifies the efficiency of light capture. By stacking thylakoids, plants increase the concentration of photosynthetic machinery. They do this within a limited space.
Think of grana as highly organized photosynthetic batteries. They are ready to be discharged when light strikes. These stacks also facilitate the efficient transfer of energy between thylakoids. This ensures that no captured light goes to waste.
Grana: Hubs of Light-Dependent Reactions
Grana are not merely structural features. They are the primary sites of the light-dependent reactions of photosynthesis. This is where light energy is converted into chemical energy in the form of ATP and NADPH.
The close proximity of thylakoids within grana allows for efficient cooperation between different photosynthetic complexes. These interactions are essential for the smooth operation of the electron transport chain. It ultimately drives the synthesis of ATP and NADPH.
The Thylakoid Membrane: A Stage for Photosynthesis
Encasing each thylakoid is the thylakoid membrane. This is a highly specialized lipid bilayer. It is embedded with a diverse array of proteins and pigment molecules. This is like chlorophyll, the green pigment responsible for capturing light energy.
The thylakoid membrane serves as the stage upon which the light-dependent reactions unfold. It provides a scaffold for the precise arrangement of photosystems. These are protein complexes that orchestrate the capture and transfer of light energy. It also maintains the proton gradient. This is essential for ATP synthesis.
The strategic location of chlorophyll within the thylakoid membrane is particularly important. It allows these pigment molecules to efficiently capture photons of light. The energy is then transferred to the reaction centers of photosystems. This kicks off the chain of events that ultimately leads to the production of chemical energy.
Individual thylakoids rarely exist in isolation. Instead, they are typically stacked upon one another, resembling neat piles of pancakes. These stacks are called grana. The formation of grana represents a crucial adaptation, further amplifying the efficiency of light capture. Now, let's delve into the heart of their purpose: how these meticulously structured thylakoids, and the grana they form, actually capture light energy and drive the initial stages of photosynthesis.
Thylakoids and Photosynthesis: Capturing Light Energy
At its core, photosynthesis is the remarkable process by which plants convert light energy into chemical energy, fueling life on Earth. This transformation hinges on a series of intricate reactions, with the thylakoids playing a central and indispensable role.
Thylakoids are the sites of the light-dependent reactions of photosynthesis. These reactions represent the first phase of the overall photosynthetic process. They capture the sun's energy and transform it into chemical forms.
The Light-Dependent Reactions: A Thylakoid Symphony
The light-dependent reactions unfold within the thylakoid membrane, a highly organized structure housing a cast of essential players. Among these are the photosystems, protein complexes that orchestrate the capture and conversion of light energy.
There are two main types of photosystems, aptly named Photosystem I (PSI) and Photosystem II (PSII). They each play distinct but interconnected roles in the light-dependent reactions.
- Photosystem II (PSII): This photosystem initiates the process by capturing light energy. It uses this energy to split water molecules into electrons, protons, and oxygen. The released electrons are then passed along an electron transport chain.
- Photosystem I (PSI): Later in the chain, after the electrons have released energy in the creation of ATP, Photosystem I re-energizes them using light. These energized electrons are then used to produce NADPH, another energy-carrying molecule.
Harnessing Sunlight: The Role of Chlorophyll
The magic of light capture relies on chlorophyll, the pigment that gives plants their green color. Chlorophyll molecules are embedded within the photosystems. They are precisely positioned to absorb specific wavelengths of light.
When chlorophyll absorbs light energy, its electrons become excited. This excitation triggers a chain of events that ultimately leads to the generation of ATP (adenosine triphosphate) and NADPH (nicotinamide adenine dinucleotide phosphate).
These energy-rich molecules, ATP and NADPH, serve as the fuels for the next stage of photosynthesis: the Calvin Cycle.
From Thylakoid to Stroma: Bridging the Gap
While the light-dependent reactions occur within the thylakoids, the subsequent Calvin Cycle takes place in the stroma, the fluid-filled space surrounding the thylakoids within the chloroplast.
The ATP and NADPH produced during the light-dependent reactions in the thylakoids provide the energy and reducing power needed to convert carbon dioxide into glucose (sugar) during the Calvin Cycle.
This elegant interplay between the thylakoids and the stroma highlights the interconnectedness of the two phases of photosynthesis. It transforms light energy into the chemical energy that sustains plant life.
Photosystem II (PSII) sets the stage, initiating the capture of light and subsequent electron transport. Photosystem I (PSI) then steps in, energizing electrons for the final steps of generating NADPH. This interplay of two different photosystems working in series is what allows photosynthesis to be such a productive process. Now, let’s consider the arrangement of thylakoids into grana to see how they influence and boost this process.
The Power of Stacking: Grana and Photosynthetic Efficiency
The formation of grana, those neat stacks of thylakoids within chloroplasts, is more than just a structural curiosity. It's a critical adaptation that significantly enhances photosynthetic efficiency. The unique architecture of grana directly contributes to a plant's ability to thrive, especially in varying light conditions.
Maximizing Light Absorption Through Stacking
The stacked arrangement of thylakoids in grana serves to maximize the surface area available for light absorption. Imagine spreading a single pancake flat versus stacking several; the stacked configuration occupies less horizontal space while presenting more surface area to an incoming light source.
Similarly, grana pack a greater amount of light-harvesting chlorophyll into a smaller volume within the chloroplast. This increase in pigment density directly translates to more efficient capture of photons, the fundamental particles of light.
This maximized light absorption is particularly crucial in environments where light may be a limiting factor for plant growth.
Evolutionary Advantage of Grana Formation
The development of grana represents a significant evolutionary leap. By concentrating photosynthetic machinery, grana enable plants to perform photosynthesis more efficiently.
This efficiency translates into faster growth rates, improved resource utilization, and ultimately, a greater ability to compete for survival. Plants with well-developed grana structures are better equipped to thrive in diverse environments, from sun-drenched fields to shaded forest floors.
The evolutionary success of grana underscores the principle that structural optimization plays a key role in biological adaptation.
Optimizing Photosystem Interaction Within Grana
The spatial arrangement of thylakoids within grana is not random. It is highly organized to facilitate the efficient transfer of energy and electrons between Photosystem II (PSII) and Photosystem I (PSI).
PSII and PSI work in series during the light-dependent reactions. PSII primarily resides in the stacked grana regions, while PSI is more concentrated in the unstacked stroma lamellae. This physical separation, yet strategic proximity, allows for an optimized electron transport chain.
Specifically, after PSII splits water and generates electrons, these electrons travel via mobile carriers to PSI, which further energizes them. The grana structure ensures that the distance these electrons need to travel is minimized, reducing energy loss and increasing the overall efficiency of the process.
This efficient interaction, facilitated by the grana structure, allows plants to effectively convert light energy into chemical energy.
Adaptation to Light Conditions
Interestingly, the structure of grana is not fixed; plant species adapt it to varying light conditions. Plants grown in low-light environments often exhibit larger grana stacks to maximize light capture.
Conversely, plants in high-light environments may have smaller grana stacks to prevent photo-damage from excessive light absorption. This plasticity in grana structure highlights the remarkable adaptability of plants to their surroundings.
This adaption indicates that the dynamic regulation of grana structure is a key mechanism for plants to optimize their photosynthetic performance under different environmental conditions.
The development of grana represents a significant evolutionary leap. By concentrating photosynthetic machinery, grana enable plants to perform photosynthesis more efficiently. This efficiency translates to greater biomass production and a competitive edge in resource acquisition. Now, let's broaden our perspective and examine the presence of thylakoids in other organisms, as well as explore potential applications stemming from a deeper understanding of thylakoid function.
Beyond Plants: Thylakoids in Other Organisms and Potential Applications
While often associated with plants, thylakoids are not exclusive to the plant kingdom. Their presence in other organisms, particularly cyanobacteria, offers insights into the evolutionary origins of photosynthesis and hints at future technological applications.
Thylakoids in Cyanobacteria: Evolutionary Pioneers
Cyanobacteria, often referred to as blue-green algae, are a group of bacteria that perform oxygenic photosynthesis. They are believed to be among the first organisms on Earth to develop this ability.
Crucially, cyanobacteria possess thylakoids, internal membrane systems where photosynthesis takes place. These thylakoids are not organized into chloroplasts as they are in plant cells, since cyanobacteria are prokaryotes and lack membrane-bound organelles.
Nevertheless, the presence of thylakoids in cyanobacteria is a strong indicator that thylakoid-based photosynthesis predates the evolution of plants.
The endosymbiotic theory proposes that chloroplasts in plant cells originated from ancient cyanobacteria that were engulfed by eukaryotic cells. Over time, these cyanobacteria evolved into the chloroplasts we see today, retaining their thylakoid membranes as the site of light-dependent reactions.
Studying the structure and function of thylakoids in cyanobacteria provides valuable information about the early evolution of photosynthesis and the development of essential photosynthetic machinery.
Harnessing Thylakoids: Bioenergy and Artificial Photosynthesis
The efficiency of thylakoids in capturing light energy has attracted considerable attention from researchers seeking sustainable energy solutions.
Two promising avenues of research are bioenergy production and artificial photosynthesis.
Bioenergy Research
Thylakoids can be utilized in bioenergy production through various methods. One approach involves extracting photosynthetic components, including thylakoid membranes and their associated proteins, to create biohybrid solar cells.
These cells mimic the natural photosynthetic process to generate electricity.
Another method explores the use of genetically engineered cyanobacteria or algae to produce biofuels, such as ethanol or biodiesel. By optimizing the photosynthetic efficiency and lipid production pathways within these organisms, researchers aim to develop sustainable and renewable energy sources.
Artificial Photosynthesis
Artificial photosynthesis seeks to replicate the natural process of photosynthesis in an artificial system. The goal is to create a device that can capture sunlight, convert it into chemical energy, and store that energy in the form of fuels like hydrogen or organic molecules.
Thylakoid membranes and their associated proteins serve as inspiration for designing artificial photosynthetic systems. Researchers are studying the structure and function of photosystems I and II to develop artificial light-harvesting complexes and electron transfer chains.
By mimicking the natural efficiency of thylakoids, scientists hope to create artificial systems that can produce clean and sustainable energy on a large scale.
Thylakoid research is showing promise in various applications, from developing biohybrid solar cells to improving bioenergy production and creating artificial photosynthetic systems. These applications highlight the potential of these tiny structures to contribute to a more sustainable energy future.
Video: Thylakoids Revealed! The Secret To Plant's Success?
Thylakoids Revealed! FAQs: Understanding Plant Power
Have questions about thylakoids and their importance to plants? This FAQ aims to clarify the key concepts from our article, helping you grasp the secret to plant success.
What exactly is a thylakoid?
A thylakoid is a membrane-bound compartment inside chloroplasts, which are organelles found in plant cells. Think of them as flattened sacs within the chloroplast. They're the site of the light-dependent reactions of photosynthesis.
How are thylakoids arranged within the chloroplast?
Thylakoids are organized into stacks called grana (singular: granum). These stacks of thylakoids are interconnected by stroma lamellae, creating a complex network within the chloroplast.
Why are thylakoids so crucial for photosynthesis?
Thylakoid membranes contain chlorophyll and other pigments that capture sunlight. This captured light energy drives the initial stages of photosynthesis, converting light energy into chemical energy. Without the stacks of thylakoids, plants would not be able to efficiently perform photosynthesis.
Are thylakoids found in all photosynthetic organisms?
While most people think of plants first, thylakoids are also present in cyanobacteria (formerly known as blue-green algae), which are photosynthetic bacteria. Their presence enables these organisms to carry out oxygenic photosynthesis, much like plants, and their thylakoids perform essentially the same function in trapping light.