Water's Hidden Property: Thermal Conductivity Explained!
Understanding the thermal behavior of water is crucial in various scientific and engineering applications. Heat transfer, a fundamental concept, significantly influences the thermal conductivity of water. For instance, research conducted at the National Institute of Standards and Technology (NIST) provides precise measurements, which are essential for validating theoretical models. Computational Fluid Dynamics (CFD) simulations rely on accurate thermal conductivity of water values to predict the performance of heat exchangers. The thermal properties also play a vital role in the study of aquatic ecosystems, where temperature gradients affect biological processes.
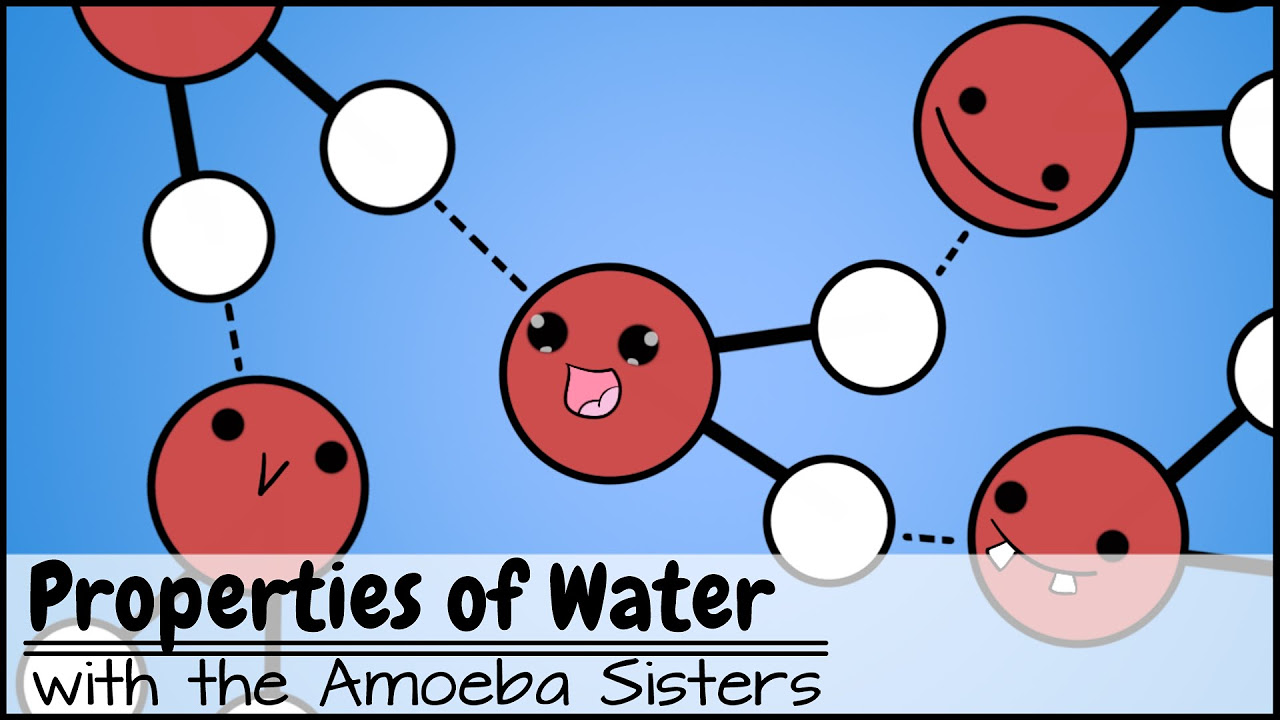
Image taken from the YouTube channel Amoeba Sisters , from the video titled Properties of Water .
Water, a ubiquitous substance that blankets our planet and sustains life, possesses a multitude of fascinating properties.
Among these, its ability to conduct heat, known as thermal conductivity, stands out as a critical parameter influencing a wide array of natural and engineered systems.
Understanding this property is not merely an academic exercise; it is essential for addressing challenges in fields ranging from climate science to advanced engineering.
Thermal Conductivity: A Concise Definition
At its core, thermal conductivity is a material property that quantifies the ability of a substance to transfer heat.
It represents the rate at which heat flows through a material, driven by a temperature difference.
Materials with high thermal conductivity, like metals, readily transmit heat, while those with low thermal conductivity, like insulators, resist heat flow.
Water, while not as conductive as metals, occupies a unique position due to its prevalence and importance in various processes.
Why Water's Thermal Conductivity Matters
The significance of understanding water's thermal conductivity stems from its central role in numerous natural and technological processes.
In the environment, it influences ocean currents, lake stratification, and the global climate.
Variations in water temperature, driven by its thermal properties, impact marine ecosystems and weather patterns.
In engineering, water's thermal conductivity is crucial for designing efficient heat exchangers, cooling systems for power plants, and thermal management strategies in various industries.
The effectiveness of these applications hinges on a precise understanding of how water conducts heat under different conditions.
Scope and Objectives
This article aims to provide a comprehensive exploration of the thermal conductivity of water.
We will delve into the molecular mechanisms governing heat transfer in water, examine the factors that influence its thermal conductivity, and explore the methods used to measure this property accurately.
Furthermore, we will highlight the diverse applications of water's thermal conductivity in various fields, emphasizing its importance in both natural and engineered systems.
By providing a thorough understanding of this fundamental property, we hope to foster further research and innovation in areas that rely on the unique thermal characteristics of water.
Defining Thermal Conductivity: The Science of Heat Flow
Water's thermal conductivity is a specific aspect of a much broader field: the study of heat transfer. Before we can fully appreciate how water conducts heat, it's crucial to understand the fundamental mechanisms by which heat moves from one place to another.
These mechanisms are conduction, convection, and radiation. Each plays a distinct role, and their relative importance depends on the specific circumstances.
The Three Pillars of Heat Transfer
Conduction: Heat Through Direct Contact
Conduction is the transfer of heat through a material without any bulk movement of the material itself. It occurs when a temperature difference exists within a substance.
Energetic particles (atoms or molecules) collide with their less energetic neighbors, transferring kinetic energy.
This process is most efficient in solids, where particles are tightly packed, but it also occurs in liquids and gases, albeit less effectively.
Convection: Heat Carried by Moving Fluids
Convection involves heat transfer through the movement of fluids (liquids or gases). When a fluid is heated, it expands and becomes less dense.
This less dense, warmer fluid rises, while cooler, denser fluid sinks to take its place, creating a circulating current.
This movement carries heat along with it. Convection can be natural, driven by density differences, or forced, driven by external means like a pump or fan.
Radiation: Heat Transfer by Electromagnetic Waves
Radiation is the transfer of heat through electromagnetic waves. Unlike conduction and convection, radiation does not require a medium to propagate.
This is how the Sun's energy reaches the Earth. All objects emit thermal radiation, and the amount of radiation emitted depends on the object's temperature and surface properties.
Conduction: The Dominant Mode in Water
While all three modes of heat transfer can occur in water, conduction is often the most important, especially in quiescent (non-moving) water bodies.
Convection can play a significant role when there are temperature gradients that induce fluid motion.
However, in many situations, particularly when considering heat transfer at a molecular level or within relatively small volumes of water, conduction is the primary mechanism.
Radiation plays a minor role unless considering direct exposure to solar radiation.
Defining Thermal Conductivity: A Material's Intrinsic Ability
Thermal conductivity is an intrinsic property of a material that describes its ability to conduct heat. It's a measure of how readily a substance allows heat to flow through it when a temperature difference is present.
A material with high thermal conductivity will quickly transfer heat, while a material with low thermal conductivity will resist heat flow.
Mathematically, thermal conductivity (often denoted by the symbol k) is defined as the amount of heat (Q) that flows through a unit area (A) of a material with a unit thickness (L) per unit time (t) for a unit temperature difference (ΔT).
This relationship is formalized in Fourier's Law of Heat Conduction:
Q = -k A (ΔT/L) * t
The negative sign indicates that heat flows from a region of higher temperature to a region of lower temperature.
Understanding the Units of Thermal Conductivity
The standard unit of thermal conductivity is Watts per meter per Kelvin (W/mK). Let's break down what this unit signifies.
- Watts (W): A unit of power, representing the rate at which energy (in this case, heat) is transferred.
- Meters (m): A unit of length, representing the distance over which the heat is transferred.
- Kelvin (K): A unit of temperature, representing the temperature difference driving the heat transfer.
Therefore, W/mK signifies the amount of power (in Watts) that is transferred through a material that is one meter thick, per square meter of area, for every one-Kelvin difference in temperature.
A higher value of thermal conductivity in W/mK indicates that the material is more effective at conducting heat.
For example, copper has a thermal conductivity of around 400 W/mK, meaning it is an excellent heat conductor. Water, on the other hand, has a thermal conductivity of around 0.6 W/mK, making it a relatively poor conductor compared to metals but still significant in many thermal processes.
Molecular Basis: How Water Conducts Heat on a Microscopic Level
Having explored the macroscopic properties of thermal conductivity, it’s time to zoom in and examine the molecular mechanisms that govern heat transfer in water. The unique structure of water, particularly its extensive hydrogen bonding network, plays a critical role in its ability to conduct heat. Understanding these microscopic interactions is key to unlocking a deeper understanding of water’s thermal behavior.
The Dance of Water Molecules: Hydrogen Bonding and Structure
Water's exceptional properties stem from its bent molecular geometry and the resulting polarity. The oxygen atom attracts electrons more strongly than the hydrogen atoms, creating a partial negative charge on the oxygen and partial positive charges on the hydrogens.
This charge distribution allows water molecules to form hydrogen bonds with each other. A hydrogen bond is a relatively weak electrostatic attraction between the partially positive hydrogen of one water molecule and the partially negative oxygen of another.
These bonds are not static; they are constantly forming and breaking, creating a dynamic network. This network is what gives water its unique structure and many of its unusual properties.
Hydrogen Bonds: The Highways for Energy Transfer
The hydrogen bonding network is not just structural; it also provides a pathway for efficient energy transfer. When one water molecule gains kinetic energy (i.e., heats up), it begins to vibrate more vigorously.
These vibrations are transmitted to neighboring molecules through the hydrogen bonds. This transfer occurs much faster than it would through simple collisions, which are the primary mechanism of heat transfer in many other liquids.
Think of it like a chain of dominoes. When the first domino falls, it quickly triggers a chain reaction, transferring energy down the line.
Similarly, the hydrogen bonding network in water allows for rapid propagation of vibrational energy, effectively increasing its thermal conductivity.
Temperature's Influence: Agitation and Disruption
Temperature plays a crucial role in modulating the strength and stability of the hydrogen bonding network. As temperature increases, water molecules gain kinetic energy.
This increased energy leads to more vigorous vibrations, which can disrupt the hydrogen bonds. At higher temperatures, the hydrogen bonds become weaker and break more frequently.
This disruption of the hydrogen bonding network has a complex effect on thermal conductivity. Initially, increased molecular vibration can enhance energy transfer.
However, at higher temperatures, the weakening of the hydrogen bonds reduces the efficiency of this transfer. The balance between these two opposing effects results in a non-linear relationship between temperature and thermal conductivity.
Density's Role: Proximity and Interaction
Density, the mass per unit volume, also significantly influences water's thermal conductivity. Higher density means that more water molecules are packed into a given space.
This closer proximity leads to a greater number of hydrogen bonds per unit volume. With more hydrogen bonds available, the efficiency of energy transfer increases, leading to higher thermal conductivity.
However, the relationship between density and thermal conductivity is not always straightforward. Other factors, such as temperature and pressure, can also affect density and, consequently, thermal conductivity.
Factors Influencing Water's Thermal Conductivity: A Delicate Balance
The thermal conductivity of water, while seemingly straightforward, is a complex property influenced by a multitude of interacting factors. Temperature, specific heat capacity, convection, and the presence of dissolved substances each play a significant role in determining how efficiently water conducts heat. Understanding these influences is crucial for accurately predicting and manipulating water's thermal behavior in various applications.
Temperature Dependence: A Non-Linear Relationship
The relationship between temperature and thermal conductivity in water is not linear. Generally, the thermal conductivity of water increases with temperature up to a certain point, typically around 120°C (248°F). Beyond this, it begins to decrease.
This behavior is linked to the disruption of the hydrogen bonding network as temperature rises.
Initially, increased temperature leads to more vigorous molecular vibrations, facilitating faster energy transfer through the hydrogen bonds. However, at higher temperatures, the hydrogen bonds begin to break down more rapidly, diminishing their effectiveness as conduits for heat. This delicate balance results in the observed peak in thermal conductivity.
Specific Heat Capacity: The Energy Reservoir
Specific heat capacity, the amount of energy required to raise the temperature of a substance by a certain amount, is intimately connected to thermal conductivity. Water's remarkably high specific heat capacity (4.186 J/g°C) means it can absorb a considerable amount of heat without undergoing drastic temperature changes.
While specific heat capacity doesn't directly increase thermal conductivity, it influences the rate at which heat diffuses through the water.
A higher specific heat capacity means that more energy needs to be transferred to raise the temperature of adjacent molecules, potentially slowing down the overall conductive process.
However, water's exceptional ability to store thermal energy also allows it to act as a significant heat sink, which is essential in applications like cooling systems.
Convection: A Disturbance to Pure Conduction
Convection, the transfer of heat through the movement of fluids, often interferes with pure conductive heat transfer in water.
When water is heated, density differences arise, causing warmer, less dense water to rise, and cooler, denser water to sink. This creates convection currents that rapidly redistribute heat throughout the fluid.
Convection dominates heat transfer when significant temperature gradients are present.
This process effectively increases the apparent thermal conductivity of the water, as heat is transported much faster than it would be through conduction alone. In experiments or applications where precise control of thermal conductivity is required, it is crucial to minimize or eliminate convection, often through careful design of the experimental setup or the use of stabilizing agents.
Influence of Dissolved Substances: Impurities and Conductivity
The presence of dissolved substances, such as salts or minerals, can significantly alter water's thermal conductivity. Generally, the addition of dissolved solids decreases the thermal conductivity of water.
This reduction occurs because the dissolved ions disrupt the hydrogen bonding network, hindering the efficient transfer of vibrational energy between water molecules.
The magnitude of the effect depends on the type and concentration of the dissolved substance. For instance, seawater, with its high salt content, has a lower thermal conductivity than pure water.
This factor is critical to consider in applications involving natural water sources or industrial processes where water contains dissolved impurities. Understanding how these substances influence thermal conductivity is necessary for accurate thermal modeling and control.
Specific heat capacity provides insight into water’s ability to store heat, convection describes heat transfer through movement, and dissolved substances can alter thermal properties. To fully understand water's thermal behavior, we need not only to consider these factors, but also the methods we use to quantify its thermal conductivity in the first place.
Measurement Techniques: Quantifying Water's Thermal Conductivity
Accurately determining the thermal conductivity of water is crucial for both scientific research and engineering applications. Several techniques have been developed for this purpose, each with its own strengths and limitations. These methods rely on carefully controlled experiments and precise measurements to extract the thermal conductivity value.
Thermal Conductivity Meters: A Window into Measurement
Thermal conductivity meters are instruments designed specifically to measure the thermal conductivity of materials, including liquids like water. These meters employ various techniques to establish a controlled temperature gradient within the sample and then measure the heat flux required to maintain this gradient. The thermal conductivity can then be calculated using Fourier's Law of heat conduction.
Fourier's Law states that the heat flux is proportional to the temperature gradient and the thermal conductivity of the material. Different types of thermal conductivity meters exist, each based on slightly different principles.
Transient Hot Wire Method
One of the most widely used methods is the transient hot wire method. This technique involves immersing a thin, electrically heated wire into the water sample. A constant current is passed through the wire, causing its temperature to increase.
The temperature rise of the wire is monitored over time. By analyzing the rate of temperature increase, the thermal conductivity of the surrounding water can be determined. The transient hot wire method is known for its accuracy and relatively simple setup.
Guarded Hot Plate Method
Another common technique is the guarded hot plate method. This method is more suitable for measuring the thermal conductivity of solids, but it can also be adapted for liquids. It involves placing the water sample between two plates, one heated and the other cooled.
A guard heater surrounds the central heating plate to ensure that all the heat flows vertically through the sample. By measuring the temperature difference between the plates and the heat flux, the thermal conductivity can be calculated.
Laser Flash Analysis
Laser flash analysis is a transient method that involves irradiating one side of the water sample with a short laser pulse. The temperature rise on the opposite side of the sample is then measured. The thermal diffusivity, which is related to the thermal conductivity, can be determined from the temperature profile.
While laser flash analysis is primarily used for solids, it can be adapted for liquid samples using appropriate sample holders.
Challenges in Accurate Measurement
Despite the advancements in measurement techniques, accurately determining the thermal conductivity of water presents several challenges. These challenges stem from the complex nature of water itself, as well as the limitations of the measurement techniques.
Convection: The Unwanted Heat Transfer
One of the main challenges is convection. Water is prone to convective heat transfer, which can interfere with the measurement of conductive heat transfer. Convection occurs when temperature differences within the water cause fluid motion, leading to the transport of heat.
This can result in an overestimation of the thermal conductivity. To minimize convection, careful experimental design is necessary. This includes using small sample sizes, maintaining stable temperature gradients, and employing techniques to suppress fluid motion.
Temperature Control and Stability
Maintaining precise temperature control during the measurement is also crucial. The thermal conductivity of water is temperature-dependent, so even small temperature fluctuations can affect the results. Highly stable temperature baths and sensitive temperature sensors are essential for accurate measurements.
Purity and Dissolved Gases
The purity of the water sample can also influence the measured thermal conductivity. Dissolved impurities, such as salts or gases, can alter the thermal properties of water. Therefore, it is important to use high-purity water and to degas the sample before measurement.
Interfacial Resistance
Interfacial thermal resistance between the sensor and the water sample can also introduce errors. This resistance arises from the imperfect thermal contact between the sensor and the water. It can lead to an underestimation of the thermal conductivity.
To minimize interfacial resistance, it is important to ensure good thermal contact between the sensor and the sample. This can be achieved by using appropriate thermal interface materials or by carefully designing the sensor geometry.
Measurement techniques provide a way to peek behind the curtain to see how we have come to our current understanding. This provides the groundwork that allows us to harness the remarkable thermal properties of water across an astonishingly wide range of applications, a testament to its unique characteristics.
Applications: Harnessing Water's Thermal Conductivity
Water's thermal conductivity, while seemingly a simple property, underpins a vast array of applications spanning engineering, industry, and the natural world.
Its ability to efficiently transfer heat, coupled with its abundance and relative safety, makes it an indispensable fluid for countless processes. From the intricate designs of heat exchangers to the delicate balance of aquatic ecosystems, water's thermal behavior plays a critical role.
Heat Exchangers: Optimizing Thermal Transfer
Heat exchangers are essential components in numerous industrial and engineering systems. They facilitate the transfer of heat between two fluids without allowing them to mix. Water's high thermal conductivity is a key reason why it is frequently employed as the working fluid in these devices.
Its efficiency in absorbing and dissipating heat enables the effective regulation of temperature in various applications. Consider power plants, where water is used to cool steam after it has driven turbines. The rapid heat transfer ensures optimal efficiency of the power generation cycle.
Similarly, in chemical processing plants, heat exchangers using water help to maintain reaction temperatures, ensuring product quality and safety. The widespread use of water in these applications underscores its value as a reliable and efficient heat transfer medium.
Cooling Systems: Maintaining Operational Integrity
The need for efficient cooling is paramount in many engineering applications, especially where heat generation is a limiting factor. Water's thermal conductivity, combined with its high specific heat capacity, makes it an ideal coolant.
Internal combustion engines rely on water-based cooling systems to prevent overheating, thus maintaining optimal operating temperatures and preventing catastrophic failures. Similarly, data centers, with their dense concentration of heat-producing servers, depend on sophisticated water cooling systems.
These systems ensure the reliable and continuous operation of critical infrastructure. The effectiveness of water as a coolant stems not only from its ability to absorb heat, but also from its capacity to transport it away from sensitive components.
Natural Processes: Lake Temperature Stratification
Water's thermal properties are not just exploited in engineered systems; they also govern many natural processes. One prominent example is the phenomenon of lake temperature stratification.
In temperate climates, lakes typically exhibit distinct temperature layers during the summer months. The surface water warms up due to solar radiation, while the deeper water remains cooler.
This temperature difference creates density gradients, preventing the mixing of the layers. Water's thermal conductivity plays a crucial role in this stratification. It influences the rate at which heat is transferred from the surface to the deeper layers.
This stratification affects nutrient distribution, oxygen levels, and the overall ecology of the lake. Understanding the thermal dynamics of lakes is crucial for managing water resources and protecting aquatic ecosystems.
Environmental Thermal Management: Ice and Steam
Water exists in multiple phases, each with distinct thermal properties. Ice and steam, the solid and gaseous forms of water, respectively, play significant roles in environmental thermal management.
Ice, with its high latent heat of fusion, absorbs a considerable amount of energy as it melts, providing a cooling effect. This is vital in regulating temperatures in polar regions and high-altitude environments.
The melting of glaciers and ice sheets has profound implications for global climate patterns and sea levels. Steam, on the other hand, carries a large amount of thermal energy.
Its release into the atmosphere can influence weather patterns and contribute to cloud formation. Understanding the interplay between water's different phases and their thermal properties is crucial for addressing climate change and managing our environment.
Video: Water's Hidden Property: Thermal Conductivity Explained!
FAQs About Water's Thermal Conductivity
This section answers common questions about water's thermal conductivity and its implications.
Why is water considered to have relatively high thermal conductivity?
Compared to other common liquids, water conducts heat surprisingly well. While not as conductive as metals, the thermal conductivity of water is significantly higher than many organic liquids. This impacts how quickly heat moves through it.
How does the thermal conductivity of water affect aquatic life?
Water's thermal conductivity helps distribute heat evenly in aquatic environments. This prevents extreme temperature fluctuations that could harm fish and other organisms.
Does the thermal conductivity of water change with temperature?
Yes, the thermal conductivity of water is temperature-dependent. Generally, as water temperature increases, its thermal conductivity also slightly increases, allowing it to transfer heat more efficiently.
How is the thermal conductivity of water used in cooling systems?
Water's ability to absorb and transfer heat efficiently makes it an ideal coolant in many industrial and domestic applications. Systems like car radiators and power plants utilize the thermal conductivity of water to dissipate excess heat.
Hopefully, you now have a better grasp of the thermal conductivity of water! Go forth and impress your friends with your newfound knowledge. Now, go experiment and thanks for reading!