Temporal Summation: Hack Your Nervous System! [Explained]
The human nervous system, a complex communication network, relies on processes like temporal summation to efficiently transmit information. Neurotransmitters, key chemical messengers, play a crucial role in this process, affecting how signals are integrated by neurons. Understanding Hebbian learning, which posits that neurons that fire together wire together, is vital to appreciating how repeated stimuli can lead to action potentials through temporal summation. Moreover, researchers at the Salk Institute continue to investigate the intricacies of neural plasticity and how techniques to harness processes like temporal summation might lead to new therapeutic interventions.
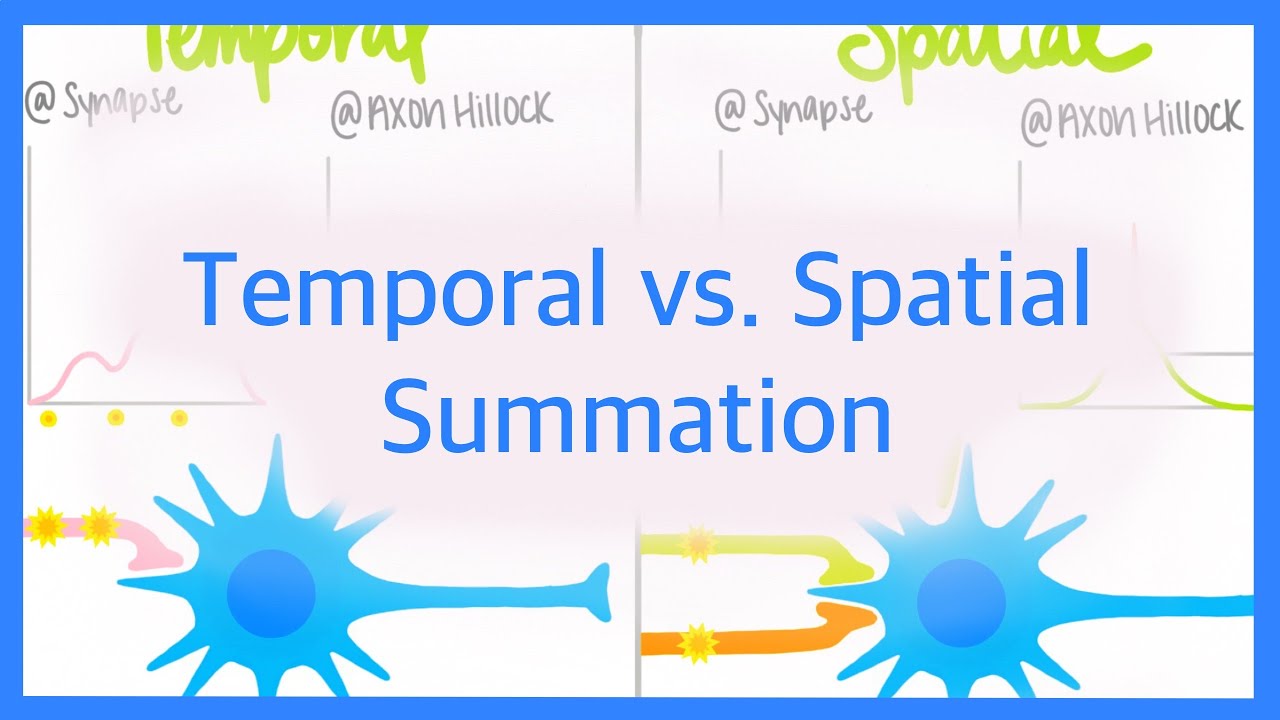
Image taken from the YouTube channel BioME , from the video titled Temporal vs. Spatial Summation .
Unlocking the Secrets of Temporal Summation
Imagine you're a musician learning a complex riff. Initially, each note feels distinct and separate. With practice, however, those individual notes begin to blur together, forming a seamless and fluid melody. This transformation, this integration of discrete events into a cohesive whole, mirrors a fundamental process occurring within your nervous system: temporal summation.
Temporal Summation: A Primer
Temporal summation is a crucial mechanism by which neurons integrate synaptic inputs that occur closely together in time.
Instead of firing an action potential in response to a single, strong stimulus, a neuron can combine multiple, weaker stimuli that arrive in rapid succession.
This allows the neuron to reach the threshold needed to fire, even if each individual stimulus would have been insufficient on its own.
This seemingly simple process underlies a vast array of experiences, from our perception of pain to our ability to learn and adapt.
The Significance of Temporal Summation in Neural Communication
At its core, the nervous system operates through a complex network of interconnected neurons.
These neurons communicate with each other via electrical and chemical signals.
Temporal summation plays a vital role in this communication by allowing neurons to process and integrate information over time.
It's not merely about adding up signals; it's about the precise timing of those signals.
Think of it as a carefully orchestrated symphony, where each instrument (neuron) must play its part at the right moment to create a harmonious whole.
Without temporal summation, our nervous system would be a chaotic mess of disconnected signals, unable to perform even the simplest tasks.
Thesis Statement: Unveiling the Mechanism, Harnessing the Potential
This article aims to delve into the intricate world of temporal summation, exploring its underlying mechanisms and its profound impact on nervous system function.
We will unpack how temporal summation relates to the generation of action potentials, the fundamental units of neural communication.
Ultimately, we'll consider the potential for leveraging our understanding of temporal summation to "hack" the nervous system, influencing everything from pain management to cognitive enhancement.
The Foundation: How Neurons Communicate
Before we can truly understand the elegant dance of temporal summation, we must first lay the foundation by exploring the fundamental mechanisms of neural communication. This involves understanding the key players: neurons, synapses, and action potentials, and how they interact to transmit information throughout the nervous system.
The Neuron: The Building Block
The neuron, or nerve cell, is the fundamental unit of the nervous system. It's a specialized cell designed to transmit information, both electrical and chemical, to other cells in the body.
Think of neurons as the "wires" of the nervous system, forming a vast, interconnected network that allows different parts of the body to communicate with each other.
Structure of a Neuron
A neuron consists of several key components, each playing a vital role in its function:
-
Dendrites: These branch-like extensions receive signals from other neurons. They act like antennae, collecting incoming messages.
-
Cell Body (Soma): This is the central part of the neuron, containing the nucleus and other essential cellular machinery. It integrates the signals received from the dendrites.
-
Axon: A long, slender projection that transmits signals away from the cell body to other neurons, muscles, or glands. Think of it as a one-way street for electrical signals.
-
Axon Terminals: The branching ends of the axon that form connections with other neurons at synapses. These terminals are the delivery points for neurotransmitters.
Neurons as Transmitters of Information
Neurons communicate through a combination of electrical and chemical signals. Electrical signals, in the form of action potentials, travel along the axon.
When these electrical signals reach the axon terminals, they trigger the release of chemical signals called neurotransmitters. These neurotransmitters then carry the message to the next neuron in the chain.
This intricate interplay of electrical and chemical signaling allows for rapid and precise communication throughout the nervous system.
Synapses: The Communication Junction
Synapses are the critical junctions where neurons communicate with each other. They are not direct physical connections, but rather tiny gaps across which signals are transmitted.
These gaps ensure that communication occurs in a controlled and specific manner.
Synaptic Transmission: A Step-by-Step Process
The process of synaptic transmission involves several key steps:
-
Neurotransmitter Release: When an action potential reaches the axon terminal, it triggers the influx of calcium ions, which causes vesicles containing neurotransmitters to fuse with the presynaptic membrane. This fusion releases the neurotransmitters into the synaptic cleft.
-
Receptor Binding: The released neurotransmitters diffuse across the synaptic cleft and bind to specific receptors on the postsynaptic membrane of the receiving neuron. These receptors are like locks that only certain neurotransmitter keys can open.
-
Postsynaptic Potential: The binding of neurotransmitters to receptors causes a change in the electrical potential of the postsynaptic neuron. This change can be either excitatory (depolarizing), making the neuron more likely to fire an action potential, or inhibitory (hyperpolarizing), making it less likely to fire.
This carefully orchestrated sequence of events allows neurons to pass information to each other, enabling the complex computations that underlie all brain functions.
Action Potentials: The All-or-None Signal
Action potentials are rapid, temporary changes in the electrical potential of a neuron's membrane. They serve as the primary means of long-distance communication within the nervous system.
Generation of Action Potentials
Action potentials are generated when the membrane potential of a neuron reaches a certain threshold. This threshold is typically around -55 mV.
When the membrane potential reaches this point, voltage-gated sodium channels open, allowing a rapid influx of sodium ions into the cell. This influx of positive charge causes the membrane potential to rapidly depolarize, creating the action potential.
After a brief period, the sodium channels close and voltage-gated potassium channels open, allowing potassium ions to flow out of the cell. This outflow of positive charge causes the membrane potential to repolarize, returning to its resting state.
The "All-or-None" Principle
Action potentials are governed by the "all-or-none" principle. This means that an action potential either fires completely or it doesn't fire at all.
There is no such thing as a "partial" action potential.
The strength of the stimulus does not affect the size or duration of the action potential, but rather the frequency at which action potentials are fired.
Long-Distance Signal Transmission
Action potentials are essential for transmitting information over long distances because they are self-regenerating. As an action potential travels down the axon, it triggers the opening of voltage-gated sodium channels in the adjacent region of the membrane, propagating the signal without any loss of strength.
This allows signals to travel from one end of a neuron to the other, even if the neuron is several feet long.
The dance of neural communication is intricate, a symphony of signals constantly being processed and interpreted. Having explored the individual instruments—the neurons, synapses, and action potentials—it's time to delve into how these elements work together to create complex neural responses. This leads us to the fascinating phenomenon of temporal summation, a key mechanism by which our nervous system integrates information over time, enabling nuanced and adaptive responses to the world around us.
Decoding Temporal Summation: Integrating Signals Over Time
Temporal summation is a fundamental process in neural communication that allows our nervous system to make sense of the world around us. It is the mechanism by which our brains integrate synaptic inputs that occur closely together in time. Unlike simply reacting to individual stimuli, temporal summation allows for a graded response, where the strength of the neural signal depends on the timing and frequency of incoming signals. This is crucial for everything from perceiving subtle changes in temperature to making split-second decisions in a fast-paced environment.
Defining Temporal Summation
At its core, temporal summation is the process where multiple subthreshold stimuli, arriving at a synapse in quick succession, are added together. This summation increases the postsynaptic potential. If this combined potential reaches the threshold for an action potential, the neuron will fire, transmitting the signal onward.
In simpler terms, imagine a neuron receiving a series of small "pushes" from another neuron. If these pushes are spaced far apart, the neuron will simply absorb them and nothing will happen. However, if these pushes come rapidly, one after the other, they will build upon each other. They increase the likelihood that the receiving neuron will "fire" and send its own signal.
The key here is timing. The stimuli must occur close enough in time for the effects of one stimulus to overlap with the effects of the next. If the time interval between stimuli is too long, the postsynaptic potential will decay before the next stimulus arrives, and summation will not occur.
How Temporal Summation Works
The mechanism behind temporal summation involves the integration of Excitatory Postsynaptic Potentials (EPSPs) and Inhibitory Postsynaptic Potentials (IPSPs).
When a presynaptic neuron releases neurotransmitters that bind to receptors on the postsynaptic neuron, it can cause a change in the membrane potential of the postsynaptic neuron. This change can either be a depolarization (making the neuron more likely to fire) or a hyperpolarization (making the neuron less likely to fire). Depolarizations are called EPSPs, while hyperpolarizations are called IPSPs.
EPSPs are like excitatory signals that push the neuron closer to its firing threshold. When several EPSPs arrive at a synapse in rapid succession, they can build upon each other, causing the membrane potential to gradually increase.
IPSPs, on the other hand, are inhibitory signals that push the neuron further away from its firing threshold. They counteract the effects of EPSPs, making it more difficult for the neuron to fire.
The interplay between EPSPs and IPSPs determines whether or not temporal summation will occur. If the sum of the EPSPs is greater than the sum of the IPSPs, and the combined potential reaches the threshold, the neuron will fire. However, if the sum of the IPSPs is greater than the sum of the EPSPs, the neuron will not fire.
Visualizing Membrane Potential Changes
Imagine a graph where the x-axis represents time and the y-axis represents membrane potential. A flat line represents the resting membrane potential of the neuron.
- Each EPSP would cause a small upward deflection in the line, representing a depolarization.
- If these deflections occur close enough together in time, they would build upon each other, creating a larger upward deflection.
- An IPSP would cause a downward deflection, counteracting the effects of the EPSPs.
- If the combined upward deflection reaches a certain threshold, an action potential will be triggered, represented by a sharp spike in the line.
Spatial Summation vs. Temporal Summation
It's important to distinguish temporal summation from spatial summation. While both are mechanisms of neural integration, they differ in how they combine signals.
-
Spatial summation occurs when multiple presynaptic neurons release neurotransmitters onto the same postsynaptic neuron at the same time. The EPSPs and IPSPs from these different synapses are then summed together to determine whether or not the postsynaptic neuron will fire. Spatial summation is about integrating signals from different locations simultaneously.
-
Temporal summation, as we've discussed, involves the summation of signals from a single presynaptic neuron over time. It's about integrating signals from the same location over time.
Both spatial and temporal summation contribute to the complex process of neural integration. They allow neurons to process information from multiple sources and respond in a nuanced and adaptive way. In many cases, both spatial and temporal summation occur simultaneously, allowing for even more sophisticated information processing. They are both key components of how our nervous system makes sense of the world and orchestrates our responses to it.
The dance of neural communication is intricate, a symphony of signals constantly being processed and interpreted. Having explored the individual instruments—the neurons, synapses, and action potentials—it's time to delve into how these elements work together to create complex neural responses. This leads us to the fascinating phenomenon of temporal summation, a key mechanism by which our nervous system integrates information over time, enabling nuanced and adaptive responses to the world around us.
Temporal Summation's Role in the Nervous System
Temporal summation isn't just a theoretical concept; it's a critical player in the everyday functioning of our nervous system. From the simplest reflexes to the most complex cognitive processes, temporal summation is constantly at work, shaping our perceptions, actions, and thoughts. Let's explore how this integration process manifests within the brain and spinal cord, how it's modulated by neurotransmitters and receptors, and what happens when this delicate system goes awry.
Temporal Summation in the Brain and Spinal Cord
The brain, the central hub of information processing, relies heavily on temporal summation. Consider sensory perception: a faint sound might not trigger a response if heard only momentarily. However, if that sound repeats rapidly, the temporal summation of EPSPs in auditory neurons can push the signal past the threshold, allowing us to perceive it.
This principle extends to higher-order cognitive functions. Complex computations and decision-making processes depend on the brain's ability to integrate information over time. Neurons in decision-making circuits might receive a series of inputs, each representing a piece of evidence. Temporal summation allows these inputs to be weighed and accumulated, ultimately leading to a decision when the threshold is reached.
The spinal cord, responsible for reflexes and motor control, also benefits from temporal summation. Think of a doctor tapping your knee to test your reflexes. The single tap might not be enough to elicit a response. However, in individuals with heightened reflexes, even a slight stimulus can result in a response due to the efficient temporal summation of signals in the spinal cord.
In motor control, temporal summation is crucial for smooth, coordinated movements. Motor neurons receive a barrage of synaptic inputs, and the precise timing of these inputs determines the strength and duration of muscle contractions.
Integration of Sensory Information
Temporal summation plays a crucial role in how we perceive the world around us. This process allows us to detect subtle changes in our environment. It also helps to distinguish between important stimuli and background noise.
Cognitive Processes and Decision-Making
The brain's capacity for complex computations and informed decisions depends significantly on its ability to integrate signals over time.
Role in Spinal Cord Reflexes
In the spinal cord, temporal summation contributes to the speed and strength of our reflexes. This ensures rapid responses to potential threats.
Role of Neurotransmitters and Receptors
The effectiveness of temporal summation isn't fixed; it's dynamically regulated by a variety of factors, including the types of neurotransmitters and receptors involved.
Different neurotransmitters have different effects on postsynaptic potentials. For example, glutamate, a primary excitatory neurotransmitter, typically elicits fast EPSPs, while other neurotransmitters may produce slower, longer-lasting EPSPs. The duration and amplitude of these EPSPs directly impact how effectively signals can summate over time.
Similarly, the type of receptor activated by a neurotransmitter plays a crucial role. Some receptors are coupled to ion channels, leading to rapid changes in membrane potential, while others activate intracellular signaling cascades that modulate neuronal excitability over longer timescales.
Furthermore, inhibitory neurotransmitters like GABA can counteract the effects of temporal summation. By eliciting IPSPs, GABA can effectively reduce the likelihood that a neuron will reach the threshold for an action potential, even if it receives a series of excitatory inputs.
Modulation by Neurotransmitters
Different neurotransmitters can influence the strength and duration of EPSPs and IPSPs, thereby affecting the overall integration process.
Influence of Receptor Types
The specific receptors activated by neurotransmitters can modulate the time course of postsynaptic potentials, further shaping the integration of signals.
Implications for Nervous System Function
When temporal summation malfunctions, the consequences can be significant. Disruptions in this process have been linked to a variety of neurological disorders, including chronic pain, epilepsy, and certain psychiatric conditions.
In chronic pain, for example, the temporal summation of pain signals can become amplified, leading to a heightened sensitivity to pain and a persistent feeling of discomfort. This phenomenon, known as "wind-up," occurs when repeated pain stimuli cause a progressive increase in the excitability of spinal cord neurons, making them more likely to fire in response to subsequent stimuli.
Epilepsy, characterized by abnormal and excessive neuronal activity, can also be influenced by dysfunctional temporal summation. In some forms of epilepsy, neurons may have a lower threshold for firing, making them more susceptible to temporal summation and increasing the likelihood of seizures.
The understanding of temporal summation mechanisms opens avenues for therapeutic interventions. By targeting specific neurotransmitter systems or receptors involved in temporal summation, it may be possible to restore normal neuronal function and alleviate the symptoms of these disorders.
For example, some pain medications work by reducing the excitability of neurons in the spinal cord, thereby decreasing the temporal summation of pain signals. Similarly, anticonvulsant drugs can help to stabilize neuronal membranes and prevent the excessive temporal summation that can lead to seizures.
Neurological Disorders
Dysfunctional temporal summation can contribute to conditions like chronic pain and epilepsy, underscoring its importance in maintaining neural stability.
Potential Therapeutic Interventions
Targeting temporal summation mechanisms offers promising avenues for developing new treatments for neurological disorders. These interventions aim to restore normal neuronal function. They also alleviate symptoms associated with these conditions.
"Hacking" Your Nervous System: Practical Applications of Temporal Summation
The dance of neural communication is intricate, a symphony of signals constantly being processed and interpreted. Having explored the individual instruments—the neurons, synapses, and action potentials—it's time to delve into how these elements work together to create complex neural responses. This leads us to the fascinating phenomenon of temporal summation, a key mechanism by which our nervous system integrates information over time, enabling nuanced and adaptive responses to the world around us.
Temporal summation isn't just a theoretical concept; it's a critical player in the everyday functioning of our nervous system. From the simplest reflexes to the most complex cognitive processes, temporal summation is constantly at work, shaping our perceptions, actions, and thoughts. This section delves into practical applications, exploring how an understanding of temporal summation can be leveraged to improve pain management, enhance learning and memory, and optimize overall performance.
Taming the Pain Signals: Temporal Summation in Pain Management
Pain, often perceived as an immediate sensation, is in reality a complex process influenced by the temporal integration of signals. Temporal summation plays a significant role in how we perceive and react to pain. Understanding this mechanism offers potential strategies for pain management.
Consider chronic pain conditions, where persistent, repeated stimuli can lead to a heightened sensitivity. This phenomenon, known as wind-up, is essentially an exaggerated form of temporal summation in pain pathways.
By understanding wind-up, we can explore techniques to disrupt or reduce the temporal summation of pain signals.
Strategies for Reducing Pain Perception
One approach involves employing techniques that introduce periods of rest or distraction between painful stimuli. This prevents the consecutive build-up of EPSPs, making it less likely that pain signals will reach the threshold for action potential firing.
For example, mindfulness and meditation practices can help individuals to shift their focus away from pain signals. These techniques can effectively break the cycle of temporal summation, reducing overall pain perception.
Another promising area lies in the development of pharmacological interventions. Certain drugs can target specific receptors involved in pain transmission.
These drugs reduce the excitability of neurons, thereby dampening the effects of temporal summation. This can lead to significant pain relief.
Boosting Brainpower: Temporal Summation, Learning, and Memory
Temporal summation isn't just about managing pain. It also plays a crucial role in shaping our ability to learn and remember. Synaptic plasticity, the brain's capacity to strengthen or weaken connections between neurons, is fundamental to learning and memory formation. Temporal summation directly influences synaptic plasticity.
When neurons are repeatedly activated in close succession, the temporal summation of EPSPs can trigger long-term potentiation (LTP). LTP is a process that strengthens the synaptic connections, making it easier for those neurons to communicate in the future. This is a critical mechanism for forming new memories and acquiring new skills.
Harnessing Temporal Summation for Enhanced Learning
By understanding how temporal summation contributes to LTP, we can devise strategies to optimize learning. Spaced repetition, a learning technique that involves reviewing information at increasing intervals, aligns perfectly with the principles of temporal summation.
By spacing out learning sessions, we allow for repeated activation of the relevant neural pathways, promoting synaptic strengthening through temporal summation.
Furthermore, techniques like interleaving, where different subjects or skills are practiced in an alternating fashion, can also enhance learning by promoting the formation of distinct neural representations through temporal summation.
This approach encourages the brain to actively differentiate between concepts, leading to more robust and flexible learning outcomes.
Peak Performance: Optimizing Cognitive and Athletic Abilities
The applications of temporal summation extend beyond pain management and learning. It can also be leveraged to optimize both cognitive and athletic performance. The ability to rapidly integrate sensory information and execute precise motor commands is essential for success in many domains.
Temporal summation plays a critical role in these processes.
In sports, for example, the ability to anticipate and react to an opponent's movements often depends on the rapid temporal integration of visual and auditory cues. Athletes train their nervous systems to become more efficient at summing up these signals. This allows them to respond more quickly and effectively.
Enhancing Performance Through Temporal Summation
Cognitive training exercises can also be designed to enhance temporal summation abilities. Tasks that require rapid decision-making or sustained attention may benefit from strategies that improve the brain's capacity to integrate information over time.
For example, practicing tasks that demand quick responses to rapidly changing stimuli can sharpen temporal processing skills. This leads to improved cognitive agility and enhanced performance in various cognitive domains.
Furthermore, understanding the role of neurotransmitters in modulating temporal summation can lead to targeted interventions for optimizing performance. Manipulating levels of certain neurotransmitters, through diet, supplements, or pharmacological means, may enhance focus, attention, and motor coordination. This, in turn, can lead to improvements in both athletic and cognitive performance.
Video: Temporal Summation: Hack Your Nervous System! [Explained]
FAQs: Temporal Summation
Got questions about temporal summation? We've compiled answers to some common inquiries to help you better understand this fascinating neurological process.
What exactly is temporal summation?
Temporal summation is how your nervous system combines multiple signals received over a short period of time to create a stronger response. Essentially, it's rapid-fire signaling that, when combined, triggers a neuron to fire that otherwise wouldn't have.
How does temporal summation relate to feeling pain?
Pain receptors can fire repeatedly in response to a persistent stimulus. If these signals arrive quickly enough, temporal summation occurs, amplifying the pain signal and making you perceive the pain as more intense than it might otherwise be.
Can I really "hack" temporal summation?
While "hack" might be a strong word, understanding temporal summation allows you to influence your nervous system. For example, by consciously relaxing muscles and breathing deeply, you can reduce the frequency of pain signals reaching your brain, lessening the impact of temporal summation on your pain perception.
What's the difference between temporal and spatial summation?
Temporal summation deals with signals arriving at different times at the same location on a neuron. Spatial summation, on the other hand, involves signals arriving at the same time but at different locations on the neuron. Both are mechanisms for neurons to integrate multiple inputs.
So, that's the gist of temporal summation! Now you have a better understanding of how your nervous system adds things up. Go forth and experiment (safely, of course!) to see how you can use this knowledge to better understand your own body and mind. Until next time!