Law of Superposition: Quantum Physics for Beginners
In the captivating realm of quantum physics, the law of superposition emerges as a cornerstone, challenging our classical intuitions about reality. This principle suggests that a quantum system, much like the electrons studied at CERN, can exist in multiple states simultaneously. The double-slit experiment, a classic demonstration often explored with tools like Quantum Inspire, beautifully illustrates this concept by showing particles passing through both slits at once. Groundbreaking physicists like Richard Feynman have significantly contributed to our understanding of superposition, emphasizing its pivotal role in quantum mechanics and its potential applications in quantum computing.
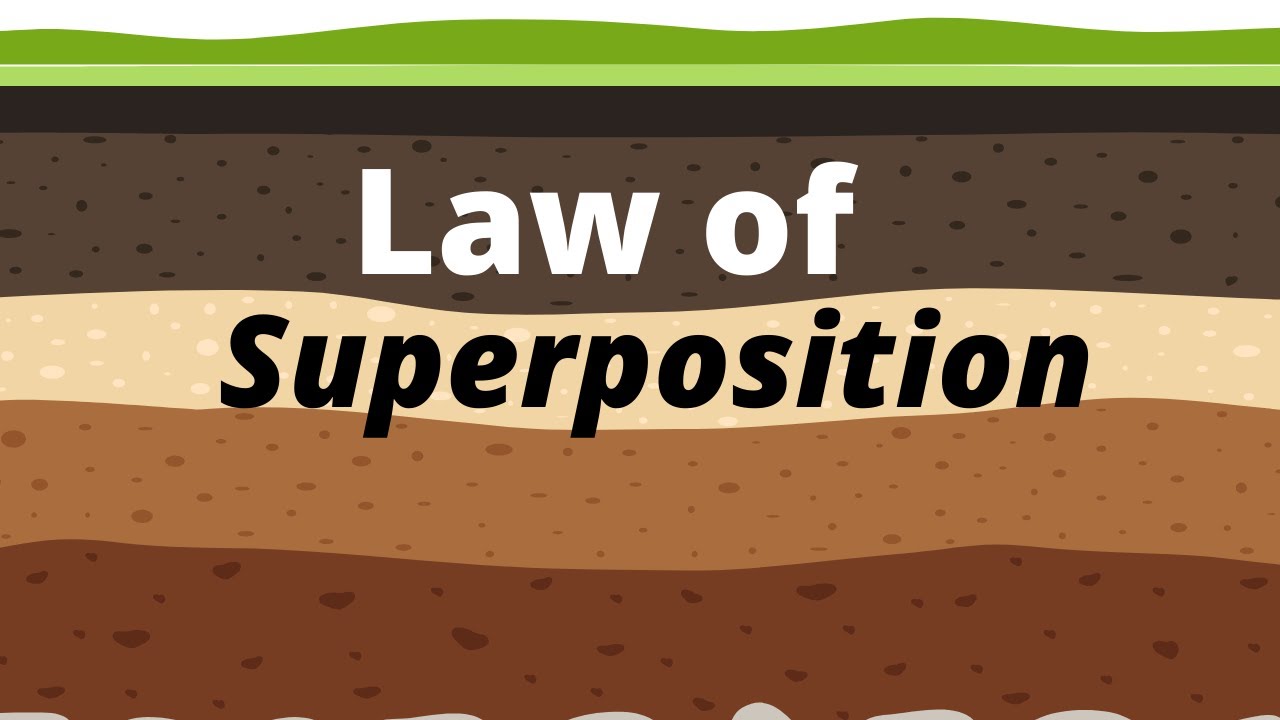
Image taken from the YouTube channel MooMooMath and Science , from the video titled Law of Superposition .
Diving into the Quantum Realm: A Beginner's Guide
Welcome to the wild and wonderful world of quantum physics! It's a realm where the rules of classical physics bend, break, and sometimes seem to stand on their head.
Even if you're new to this fascinating subject, trust us, there's plenty to get excited about. Prepare to have your mind expanded!
What is Quantum Physics Anyway?
Quantum physics, at its heart, is the study of the incredibly small. We're talking atoms, electrons, and the other fundamental particles that make up everything around us.
But here's the catch: the behavior of these tiny particles doesn't always line up with what we see in our everyday lives.
Counter-intuitiveness is practically a badge of honor in the quantum world. Particles can act like waves, and waves can act like particles. It's a bit mind-bending, but that's precisely what makes it so intriguing.
Why Should Beginners Care?
You might be thinking, "Okay, that sounds interesting, but why should I bother learning about something so abstract?"
The truth is, quantum mechanics isn't just some theoretical exercise. It's the foundation for much of the technology we rely on today.
Think about it: lasers, transistors, medical imaging, and even the smartphone in your pocket all owe their existence to our understanding of quantum principles.
Moreover, quantum physics is revolutionizing our understanding of the universe itself.
From the Big Bang to the mysteries of dark matter and dark energy, quantum mechanics offers critical insights into the very fabric of reality.
Superposition: A Quantum Leap in Understanding
Now, let's dive into one of the most mind-blowing concepts in quantum physics: superposition.
Defining Superposition Simply
Imagine a coin spinning in the air. Before it lands, it's neither heads nor tails, it's both at the same time in a probabilistic state. Superposition is somewhat like that.
In the quantum world, a particle can exist in multiple states simultaneously. It's not just that we don't know which state it's in; it's genuinely in all possible states at once!
Only when we measure or "look" at the particle does it "choose" one state or another.
Everyday Analogies (With Caveats!)
While superposition is a truly quantum phenomenon, we can try to grasp it with some relatable (though imperfect) analogies.
Think of a light switch that could be both on and off at the same time, or a musical note that could be both A and B simultaneously.
These aren't perfect comparisons, but they can help you visualize the idea of multiple possibilities existing at once.
Superposition: A Fundamental Principle
Superposition isn't just some weird quirk of quantum mechanics; it's a fundamental principle.
It's a cornerstone of quantum computing, quantum cryptography, and many other emerging technologies. Understanding superposition is key to unlocking the full potential of the quantum world.
It's a quantum leap in how we understand the universe, and it's just the beginning of an incredible journey.
Pioneers of Superposition: Meet the Scientists
Now that we've dipped our toes into the quantum realm, let's meet some of the brilliant minds who paved the way for our understanding of superposition. These scientists weren't just theorists; they were revolutionaries, challenging our fundamental understanding of reality itself.
Erwin Schrödinger: The Cat and the Equation
Erwin Schrödinger (1887-1961) was an Austrian-Irish physicist who made ground-breaking contributions to quantum theory. He is best known for the Schrödinger Equation and the famous Schrödinger's Cat thought experiment.
The Schrödinger Equation: A Quantum Cornerstone
The Schrödinger Equation is a mathematical equation that describes the time evolution of quantum mechanical systems. It's a cornerstone of quantum mechanics, much like Newton's laws are to classical mechanics.
It allows us to calculate the probability of finding a particle in a specific state at a given time.
Schrödinger's Cat: Alive and Dead?
Schrödinger's Cat is perhaps his most famous contribution to popular science, a thought experiment designed to illustrate the paradoxes of applying quantum superposition to everyday objects.
Imagine a cat in a sealed box with a radioactive atom, a Geiger counter, a hammer, and a vial of poison. If the atom decays, the Geiger counter triggers the hammer, which breaks the vial, killing the cat.
The quantum paradox arises because, until the box is opened, the atom exists in a superposition of both decayed and undecayed states. According to quantum mechanics, the cat is simultaneously alive and dead until we open the box and "observe" its state.
This thought experiment highlights the counterintuitive nature of superposition and how it challenges our classical intuitions about reality.
Werner Heisenberg: Uncertainty and the Quantum Dance
Werner Heisenberg (1901-1976) was a German physicist and one of the key founders of quantum mechanics. He is most famously known for formulating the Uncertainty Principle.
The Uncertainty Principle: Limits to Knowledge
The Uncertainty Principle states that there is a fundamental limit to the precision with which certain pairs of physical properties of a particle, such as position and momentum, can be known simultaneously.
The more accurately we know the position of a particle, the less accurately we can know its momentum, and vice versa.
How does this relate to superposition? The Uncertainty Principle reinforces the idea that quantum particles do not have definite properties until measured. Their existence is described by probabilities until observation forces them into a particular state. In a sense, the particle exists in a superposition of possible positions and momentums.
Max Born: Probability and the Quantum Dice
Max Born (1882-1970) was a German physicist and mathematician who played a crucial role in the development of quantum mechanics. He is celebrated for his probabilistic interpretation of the wave function.
Born's Rule: Interpreting Quantum Possibilities
Born proposed that the square of the magnitude of the wave function represents the probability density of finding a particle at a particular location.
This interpretation was a radical departure from classical physics, which assumed that physical properties could be precisely determined.
Born's rule tells us that quantum mechanics doesn't predict definite outcomes, but rather the probabilities of different outcomes. This probabilistic nature is intimately linked to superposition because the wave function describes the superposition of possible states.
Paul Dirac: Bridging Classical and Quantum
Paul Dirac (1902-1984) was a British theoretical physicist who made seminal contributions to both quantum mechanics and quantum electrodynamics. He is renowned for his elegant mathematical formalism and for predicting the existence of antimatter.
Bra-Ket Notation: A Quantum Language
Dirac introduced the bra-ket notation (⟨ | ⟩), a powerful and concise way to represent quantum states. This notation simplifies the mathematical manipulation of quantum states and is widely used in modern quantum mechanics.
The "ket" (|ψ⟩) represents a quantum state vector, while the "bra" (⟨φ|) represents its conjugate transpose.
This notation is crucial for describing and manipulating superposition states, allowing physicists to elegantly express the combination of multiple quantum states into a single, coherent superposition.
Richard Feynman: Paths to Understanding
Richard Feynman (1918-1988) was an American theoretical physicist known for his contributions to quantum electrodynamics, path integral formulation of quantum mechanics, and particle physics.
He was also a gifted communicator, known for his ability to explain complex ideas in an accessible way.
The Path Integral Formulation: Exploring Every Possibility
Feynman developed the path integral formulation of quantum mechanics, which provides an alternative way to calculate the probability of a particle transitioning from one point to another.
Instead of focusing on a single trajectory, the path integral sums over all possible paths the particle could take, each weighted by a phase factor.
This approach directly reflects the principle of superposition. The particle doesn't choose a single path; it explores all possible paths simultaneously, and the final probability is determined by the interference of all these paths.
Understanding Superposition: Core Quantum Concepts
To truly grasp the seemingly bizarre nature of superposition, we need to unpack some core quantum concepts. These concepts, while mathematically rigorous, can be understood conceptually with the right approach. They form the foundation upon which our understanding of superposition, and indeed, the entire quantum world, is built. Let's begin!
Quantum State: The Foundation
The quantum state is perhaps the most fundamental concept. It represents the complete description of a quantum system at any given time. Think of it as the "settings" of a quantum system, defining all its properties. Unlike classical physics where a system's state can be precisely defined (e.g., position and velocity), a quantum state can be a combination of possibilities.
Superposition is inherently linked to the quantum state because it describes the possibility of a quantum system existing in multiple states simultaneously. It's not that the system is randomly jumping between states, but rather exists in a blended combination of all the possible states available to it. This is a significant departure from our everyday experience.
Wave Function: The Quantum Code
But how do we represent this "blended combination" mathematically? Enter the wave function. The wave function, denoted typically by the Greek letter psi (Ψ), is a mathematical function that describes the quantum state of a system. It contains all the information we can possibly know about that system.
Think of the wave function as a quantum code. It tells us about the probabilities of finding the system in different states when we perform a measurement. The wave function doesn't tell us definitively what state the system is in; instead, it provides the probabilities of finding it in each possible state. This probabilistic nature is a key element of quantum mechanics.
Probability Amplitude: Decoding the Possibilities
Within the wave function reside probability amplitudes. These are complex numbers that, when squared, give us the probability of finding the system in a specific state. These amplitudes are crucial for understanding superposition.
A higher amplitude for a particular state means a higher probability of finding the system in that state upon measurement. These amplitudes, due to their complex nature, can interfere with each other, leading to the fascinating phenomenon of quantum interference.
Quantum Measurement: The Act of Observation
Now, what happens when we try to measure a quantum system in superposition? This is where things get even stranger. The act of measurement forces the system to "choose" one specific state. This is often referred to as superposition collapse.
Before measurement, the system exists in a superposition of states. After measurement, it exists in only one definite state. The probability of ending up in each state is determined by the square of the probability amplitude associated with that state, as defined by the wave function.
The Observer Effect and the Measurement Problem
It's crucial to understand the measurement process. Many interpret it that the act of measurement collapses the superposition. This leads to the infamous "measurement problem". What constitutes a measurement? Does a conscious observer need to be involved? These questions are still debated among physicists. The key takeaway is that measurement fundamentally alters the quantum system.
Qubit: Quantum Bit
Finally, let's introduce the qubit. It's the basic unit of quantum information, analogous to the bit in classical computing. However, unlike a classical bit which can only be 0 or 1, a qubit can exist in a superposition of 0 and 1.
This ability to be in a superposition of states is what gives quantum computers their potential for incredible processing power. A qubit can represent a vast number of possibilities simultaneously, opening doors to solving problems that are intractable for classical computers.
Visualizing Superposition: The Double-Slit Experiment
Understanding Superposition: Core Quantum Concepts To truly grasp the seemingly bizarre nature of superposition, we need to unpack some core quantum concepts. These concepts, while mathematically rigorous, can be understood conceptually with the right approach. They form the foundation upon which our understanding of superposition, and indeed, the entire quantum world, rests. Now, let's journey into one of the most visually compelling demonstrations of superposition: the double-slit experiment.
Setting the Stage: The Experiment Unveiled
Imagine a wall with two slits cut into it.
Now, picture firing tiny particles, like electrons, at this wall.
What do you expect to see on a screen behind the wall?
Classically, we'd anticipate two distinct bands, corresponding to the particles that passed through each slit.
But quantum mechanics throws a wrench into this intuitive picture.
Instead of two bands, we observe an interference pattern – a series of alternating high and low intensity regions.
This pattern is characteristic of waves, not particles.
Wave-Particle Duality: A Quantum Cornerstone
The double-slit experiment is a powerful demonstration of wave-particle duality, a central tenet of quantum mechanics.
It shows that particles, which we typically think of as localized objects, can also exhibit wave-like behavior.
But it doesn't mean an electron is both a wave and a particle.
The experiment reveals that the electron propagates as a wave, but interacts (detects) as a particle.
This seemingly paradoxical behavior is not a limitation, but actually the fundamental attribute of quantum objects.
How can a single particle go through both slits simultaneously to create the interference?
That's where superposition comes in.
Superposition in Action: Through Both Slits at Once
Before measurement (before the electron hits the screen), the electron exists in a superposition of states.
It's not going through one slit or the other; it's in a combination of both possibilities at the same time.
The wave function describes this superposition, with each slit having an associated probability amplitude.
These probability amplitudes interfere, just like water waves, creating the interference pattern.
This interference shows how probability amplitude is distributed.
Superposition is the key to understanding how the electron seems to be in multiple places at once.
It highlights the probabilistic nature of quantum mechanics before a measurement collapses the wave function.
The Act of Observation: Collapsing Superposition
Now, what happens if we try to observe which slit the electron goes through?
If we place a detector at one of the slits, the interference pattern disappears.
The electron now behaves as a classical particle, going through either one slit or the other.
This is because the act of measurement collapses the superposition.
The electron is forced to "choose" a definite state, and its wave-like behavior vanishes.
The observer effect demonstrates that measurement inevitably influences the quantum phenomenon.
It plays a key role in quantum measurement theory and philosophical interpretation.
The double-slit experiment vividly illustrates the strangeness and beauty of superposition.
It provides an accessible way to visualize a concept that lies at the heart of quantum mechanics.
Superposition in Action: Quantum Technologies
Visualizing superposition through experiments like the double-slit is fascinating.
But the true excitement lies in how we can harness this quantum weirdness.
We're on the cusp of a technological revolution, with superposition as a driving force.
Let's explore the groundbreaking applications of superposition in quantum computing and cryptography.
Quantum Computing: Unleashing Quantum Power
Classical computers store information as bits.
Each bit is either a 0 or a 1.
Quantum computers, however, use qubits.
Qubits can be in a superposition of both 0 and 1 simultaneously.
The Power of Parallelism
This "both-at-once" capability is what gives quantum computers their phenomenal power.
They can explore many possibilities in parallel.
This unlocks the ability to solve problems that are intractable for even the most powerful classical computers.
Applications on the Horizon
The potential applications are staggering:
- Drug Discovery: Simulating molecular interactions to design new drugs and therapies.
- Materials Science: Discovering new materials with specific properties for various applications.
- Financial Modeling: Optimizing investment strategies and managing risk more effectively.
- Artificial Intelligence: Accelerating machine learning algorithms and creating more intelligent systems.
The development of fault-tolerant quantum computers is still an ongoing challenge.
But the progress is accelerating rapidly.
It holds the promise to transform industries and redefine what's computationally possible.
Quantum Cryptography: Secure Communication
Imagine a world where your communications are completely secure from eavesdropping.
Quantum cryptography, also known as quantum key distribution (QKD), brings us closer to that reality.
It uses the principles of superposition and quantum measurement to create unbreakable encryption keys.
The Unbreakable Code
The security of QKD relies on the fact that any attempt to observe a quantum system collapses its superposition.
If an eavesdropper tries to intercept the key exchange, they will inevitably disturb the quantum states.
This disturbance is detectable by the sender and receiver.
They'll be alerted to the presence of an intruder and can discard the compromised key.
Securing the Future
QKD is being deployed in various applications, from securing government communications to protecting financial transactions.
While still a relatively young technology, it offers a path toward truly secure communication in an increasingly interconnected world.
Superposition makes Quantum Cryptography unique and ensures that sensitive information remains confidential.
Video: Law of Superposition: Quantum Physics for Beginners
FAQs: Law of Superposition
What does the law of superposition mean in simple terms?
The law of superposition states that a quantum system, like an electron, can exist in multiple states at the same time until measured. It's like being in multiple places at once, until you "look" at it, forcing it to choose one location.
Isn't superposition just uncertainty?
Not quite. Superposition is more than just not knowing the state. It means the system is in multiple states simultaneously, existing as a combination of possibilities. Uncertainty arises because we can’t predict which state we'll find when we measure it. This fundamental difference is crucial to understanding the law of superposition.
Can I see superposition in everyday life?
No, superposition is a quantum phenomenon primarily observed with extremely small particles like atoms and electrons. The effects of the law of superposition are not noticeable in macroscopic objects due to decoherence, a process where interactions with the environment cause the superposition to collapse.
If something is in superposition, does it have all possibilities equally?
Not necessarily. The law of superposition doesn't imply equal probabilities for each state. Some states might be more likely than others. These probabilities are determined by the quantum system's wave function, which describes the likelihood of finding the system in a particular state upon measurement.
So, there you have it – a whirlwind tour of the law of superposition! It might sound a bit mind-bending, and honestly, it is! But hopefully, you now have a better grasp of this fundamental concept in quantum physics. Keep exploring, keep questioning, and who knows, maybe you'll be the one to unlock even more of the universe's quantum secrets!