Master Solute Potential: Guide + Calculator for Perfect Data
Understanding plant physiology requires grasping the concept of solute potential, a crucial factor in water movement within plants. Solute potential, heavily influenced by dissolved substances, affects how plants absorb water from the soil, which agricultural organizations like the USDA closely monitor for optimal crop yields. Calculating this potential can be complex, but luckily, a solute potential calculator simplifies this process and provides accurate values. Mastering these calculations is key to understanding the work of plant scientists like Dr. Melvin Tyree, whose research significantly advanced our understanding of water relations in plants, and allows us to better understand the influence of factors such as osmotic pressure.
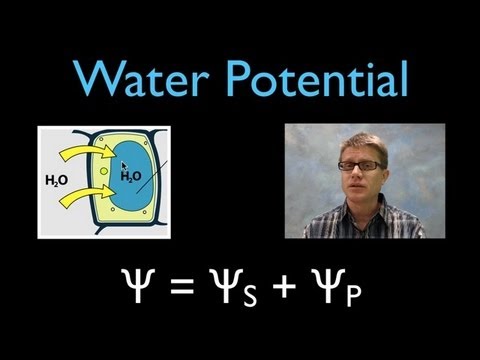
Image taken from the YouTube channel Bozeman Science , from the video titled Water Potential .
In the intricate dance of life, water plays a pivotal role. Its movement in and out of cells, across membranes, and throughout organisms is governed by a fundamental principle: water potential.
One of the key drivers of water potential is solute potential, a concept that unlocks a deeper understanding of biological and chemical processes.
This exploration delves into the heart of solute potential, unraveling its significance and providing the knowledge needed to understand this critical concept.
Defining Solute Potential: The Force of Dissolved Substances
Solute potential, often denoted by the symbol Ψs, is defined as the reduction in water potential due to the presence of solute molecules.
In simpler terms, it's a measure of how much dissolved substances lower the free energy of water in a system.
Pure water, under standard conditions, has a solute potential of zero. However, the introduction of solutes invariably results in a negative solute potential.
This negativity arises because solute molecules bind to water molecules, reducing the number of water molecules available to do work (like moving across a membrane).
The more solute present, the more negative the solute potential, and the greater the tendency for water to move towards that solution.
This principle has far-reaching implications in various fields, from plant physiology to environmental science.
Understanding solute potential provides a framework for understanding phenomena such as osmosis, turgor pressure, and the overall health of living organisms.
The Utility of a Solute Potential Calculator
Calculating solute potential manually can be cumbersome, requiring careful attention to units and formulas. Thankfully, a solute potential calculator offers a streamlined solution.
These calculators provide accurate and rapid determination of solute potential based on user-supplied inputs.
The most common inputs are concentration (molarity), temperature, and the van't Hoff factor, which accounts for the dissociation of solutes in solution.
By automating the calculations, these tools minimize the risk of human error and save valuable time.
The utility of a solute potential calculator extends beyond simple calculations. It is an indispensable tool for:
- Data Analysis: Researchers can quickly analyze experimental data and draw meaningful conclusions about the effects of solutes on water potential.
- Predictive Modeling: Scientists can use solute potential calculators to predict the movement of water in different environments.
- Educational Purposes: Students can use these calculators to reinforce their understanding of solute potential and its applications.
Water Potential: A Broader Perspective
While solute potential focuses on the impact of dissolved substances, it's important to remember that it's just one component of the larger concept of water potential (Ψ).
Water potential represents the potential energy of water per unit volume relative to pure water at atmospheric pressure and sea level.
It dictates the direction of water movement in biological systems.
Water potential is influenced by several factors, including:
- Solute Potential (Ψs): As previously discussed, the presence of solutes lowers water potential.
- Pressure Potential (Ψp): This reflects the physical pressure exerted on water, such as turgor pressure in plant cells. It can be positive or negative.
- Matric Potential (Ψm): This component accounts for the adhesion of water molecules to surfaces, such as soil particles. It's usually negative.
The relationship between these components can be expressed as:
Ψ = Ψs + Ψp + Ψm
In most biological contexts, matric potential is negligible, simplifying the equation to:
Ψ = Ψs + Ψp
Understanding the interplay between these different components is crucial for comprehending water movement in complex biological systems.
The Utility of a Solute Potential Calculator serves as an efficient means to derive understanding, it’s crucial to first appreciate the underlying scientific principles that govern solute potential itself.
The Science Behind Solute Potential: A Deeper Dive
Let's embark on a more thorough investigation of the science that underpins solute potential.
This exploration will clarify the relationship between solute potential, osmosis, and osmotic pressure.
It will also elaborate on the complex interplay between water potential and solute potential.
Unpacking Solute Potential: A Detailed Definition
At its core, solute potential (Ψs) is a measure of how the presence of solutes reduces the water potential of a solution.
More technically, it's the potential energy of water per unit volume relative to pure water, when both are at atmospheric pressure and the same temperature.
Pure water, by definition, has a solute potential of zero.
The addition of any solute will invariably decrease the water potential, resulting in a negative value for Ψs.
This is because the solute molecules bind to water molecules, which reduces the freedom of water molecules to move and do work.
The Van't Hoff Equation
The most common formula used to calculate solute potential is the Van't Hoff equation:
Ψs = -iMRT
Where:
- Ψs is the solute potential (measured in bars or Pascals).
- i is the Van't Hoff factor (number of ions or particles per molecule of solute).
- M is the molar concentration of the solute (mol/L).
- R is the ideal gas constant (0.00831 L MPa/mol K or 0.0831 L bar/mol K).
- T is the temperature in Kelvin (K).
The negative sign indicates that the solute potential is always zero or negative.
Note the importance of the Van’t Hoff Factor (i).
This factor corrects for solutes that dissociate in water, such as NaCl, which splits into Na+ and Cl- ions, effectively doubling the number of particles.
Units are critical to ensure accurate calculation. Molarity should be in mol/L, and temperature must be converted to Kelvin.
Solute Potential, Osmosis, and Osmotic Pressure: An Intertwined Relationship
Solute potential is intrinsically linked to osmosis and osmotic pressure.
Osmosis is the movement of water across a semipermeable membrane from an area of high water potential to an area of low water potential.
This gradient of water potential is often driven by differences in solute concentration.
The area with higher solute concentration has a lower (more negative) solute potential.
Therefore, water will move towards that area, attempting to equalize the water potential across the membrane.
Osmotic pressure is the pressure required to prevent the flow of water across a semipermeable membrane due to osmosis.
It is directly proportional to the solute concentration.
A solution with a higher solute concentration will have a higher osmotic pressure, meaning it requires more pressure to prevent water from flowing into it.
Solute potential contributes directly to osmotic pressure; the more negative the solute potential, the higher the osmotic pressure.
This is because a more negative solute potential indicates a greater tendency for water to move into that solution.
Water Potential: The Sum of Its Parts
Water potential (Ψ) represents the potential energy of water per unit volume relative to pure water at atmospheric pressure and temperature.
It determines the direction of water movement in plants, animals, and other systems.
Water potential is influenced by several factors.
The primary factors are solute potential (Ψs) and pressure potential (Ψp).
The relationship is expressed as:
Ψ = Ψs + Ψp
Where:
- Ψ is the water potential.
- Ψs is the solute potential.
- Ψp is the pressure potential.
Pressure potential refers to the physical pressure on a solution.
In plant cells, this is often turgor pressure, the pressure exerted by the cell membrane against the cell wall.
In summary, solute potential is a key component of water potential, influencing the movement of water in biological systems.
While solute potential is the reduction in water potential due to solutes, pressure potential can be positive or negative and reflects the physical pressure on the water.
Understanding how these components interact is fundamental to comprehending water relations in living organisms.
The Utility of a Solute Potential Calculator serves as an efficient means to derive understanding, it’s crucial to first appreciate the underlying scientific principles that govern solute potential itself.
Key Factors Influencing Solute Potential: Concentration, Temperature, and the Van't Hoff Factor
Solute potential isn't a fixed property; rather, it's a dynamic value influenced by several key environmental and solute-specific factors. Understanding these factors is essential for both accurate calculations and insightful interpretations. The primary determinants of solute potential are the concentration of the solute, the temperature of the system, and the Van't Hoff factor, each playing a critical role and working in concert via the Van't Hoff equation.
The Influence of Concentration (Molarity)
Concentration, expressed as molarity (M), has a direct and proportional effect on solute potential. As the concentration of a solute increases, the solute potential becomes more negative.
This inverse relationship arises because a higher solute concentration implies more solute molecules binding to water molecules. This, in turn, reduces the water's freedom to move and, consequently, its potential energy.
Imagine adding salt to water: the more salt you add (increasing the molarity), the lower the water potential becomes. The water is "less free" to perform work, due to being bound by the solute.
The Role of Temperature (Kelvin/Celsius)
Temperature also plays a significant role in modulating solute potential. An increase in temperature generally leads to a less negative (or more positive) solute potential. This occurs because higher temperatures increase the kinetic energy of the water molecules, counteracting the binding effect of the solute.
However, it's crucial to note that temperature in the Van't Hoff equation must be expressed in Kelvin (K). To convert Celsius (°C) to Kelvin, use the following formula: K = °C + 273.15.
Using the correct temperature unit is vital for accurate solute potential calculations. For instance, calculations involving biological systems often need adjustment to reflect physiological temperatures.
Understanding the Van't Hoff Factor
The Van't Hoff factor (i) is a dimensionless number that represents the number of ions or particles formed when a solute dissolves in a solution. This factor accounts for the dissociation of solutes into multiple particles.
For example, NaCl (sodium chloride) dissolves into two ions (Na+ and Cl-) in water. Therefore, its Van't Hoff factor is 2. Glucose, on the other hand, does not dissociate and has a Van't Hoff factor of 1.
The Importance of Accurate Van't Hoff Factor Determination
The Van't Hoff factor is crucial for determining the effective concentration of solute particles in a solution. Failing to account for dissociation can lead to significant errors in solute potential calculations. For instance, a 1 M solution of NaCl effectively contributes twice the number of particles compared to a 1 M solution of glucose.
It's also important to consider that the Van't Hoff factor can be affected by concentration. In highly concentrated solutions, ion pairing can occur, leading to a reduction in the effective number of particles.
Applying the Van't Hoff Equation
The Van't Hoff equation (Ψs = -iMRT) integrates these key factors to provide a quantitative measure of solute potential. To accurately calculate Ψs, it's essential to:
- Determine the correct Van't Hoff factor (i) for the solute.
- Measure or obtain the molar concentration (M) of the solute.
- Ensure temperature (T) is expressed in Kelvin.
- Use the appropriate value for the ideal gas constant (R), depending on the units desired for Ψs (e.g., 0.00831 L MPa/mol K).
By carefully considering these factors and applying the Van't Hoff equation correctly, one can accurately calculate and interpret solute potential in various biological and chemical contexts. This provides valuable insights into water movement, osmotic processes, and overall system behavior.
Temperature also plays a significant role in modulating solute potential. An increase in temperature generally leads to a less negative (or more positive) solute potential. This occurs because higher temperatures increase the kinetic energy of the water molecules, counteracting the constraints imposed by the solute. With a firm grasp of these factors, we can now turn our attention to the practical application of this knowledge through a solute potential calculator.
The Solute Potential Calculator: A Step-by-Step Guide
A solute potential calculator is an indispensable tool for scientists, students, and anyone working with solutions, especially in fields like biology, agriculture, and chemistry. It streamlines the process of calculating solute potential, saving time and reducing the likelihood of manual calculation errors. Here's a comprehensive guide on how to effectively use a solute potential calculator.
Understanding the Calculator's Interface
Most solute potential calculators feature a user-friendly interface with input fields for the necessary variables: concentration (Molarity), temperature (Kelvin or Celsius), and the Van't Hoff factor. Some calculators may also include a field for the pressure potential if you're calculating total water potential. Familiarize yourself with the layout before proceeding.
Inputting Values: A Detailed Walkthrough
Accurate input is paramount for obtaining reliable results. Let's break down the process for each variable:
Entering the Concentration (Molarity)
The concentration, typically expressed as molarity (M), represents the number of moles of solute per liter of solution. Ensure that your concentration value is in the correct units. Some calculators might offer unit conversion tools, but it's best practice to double-check beforehand.
For example, if you have a solution with 0.5 moles of NaCl dissolved in 1 liter of water, the molarity is 0.5 M. Enter this value directly into the designated field.
Specifying the Temperature (Kelvin/Celsius)
Temperature plays a crucial role, and solute potential calculators usually accept temperature in either Kelvin (K) or Celsius (°C). If your initial temperature is in Celsius, and the calculator requires Kelvin, remember to convert it using the formula: K = °C + 273.15.
For instance, if the temperature is 25°C, convert it to Kelvin: K = 25 + 273.15 = 298.15 K. Input this value accurately.
Determining and Applying the Van't Hoff Factor
The Van't Hoff factor (i) accounts for the number of particles a solute dissociates into when dissolved in a solvent. For non-ionic solutes like glucose, i is typically 1, as they do not dissociate. However, for ionic compounds like NaCl, i is approximately 2 because it dissociates into Na+ and Cl- ions.
For more complex compounds, the Van't Hoff factor might be different and should be determined experimentally or consulted from a reliable source. Input the correct Van't Hoff factor for your specific solute.
Running the Calculation and Understanding the Output
Once you've entered all the necessary values, click the "Calculate" button. The calculator will then compute the solute potential using the Van't Hoff equation:
Ψs = -iMRT
Where:
- Ψs is the solute potential
- i is the Van't Hoff factor
- M is the molar concentration
- R is the ideal gas constant (0.00831 L MPa/mol K)
- T is the temperature in Kelvin
The result will typically be displayed in units of pressure, such as megapascals (MPa) or bars.
Interpreting the Calculated Solute Potential Value
The calculated solute potential value provides critical information about the solution's properties. A more negative solute potential indicates a higher solute concentration and a greater tendency for water to move into the solution via osmosis. Conversely, a less negative (or more positive) solute potential suggests a lower solute concentration and a reduced osmotic pull.
Solute Potential and Water Movement
In biological systems, the solute potential helps determine the direction of water movement across cell membranes. Water will move from areas of higher water potential (less negative solute potential) to areas of lower water potential (more negative solute potential).
Significance in Plant Physiology
Understanding solute potential is particularly vital in plant physiology. Plant cells maintain turgor pressure, which is essential for rigidity and growth, by controlling solute concentrations.
By using a solute potential calculator, researchers and agricultural professionals can optimize irrigation strategies, fertilizer applications, and other practices to ensure plants thrive.
Temperature also plays a significant role in modulating solute potential. An increase in temperature generally leads to a less negative (or more positive) solute potential. This occurs because higher temperatures increase the kinetic energy of the water molecules, counteracting the constraints imposed by the solute. With a firm grasp of these factors, we can now turn our attention to the practical application of this knowledge through a solute potential calculator.
Solute Potential in Biological Systems: Plant Cells and Water Uptake
The principles of solute potential find perhaps their most compelling illustration within biological systems, especially in the intricate world of plant cells. The capacity of plants to thrive hinges significantly on their ability to manage water uptake and maintain cellular integrity. This is where solute potential steps into the limelight, acting as a key player in processes vital to plant life.
Turgor Pressure: The Rigidity of Life
Turgor pressure, the pressure exerted by the cell contents against the cell wall, is fundamental to plant structure and function. Solute potential directly influences turgor pressure by affecting the movement of water into and out of the cell. A more negative solute potential within the cell, relative to its surroundings, draws water in via osmosis.
This influx of water inflates the cell, pushing the plasma membrane against the cell wall and creating turgor. This pressure provides rigidity to non-woody plants, allowing them to stand upright. Without sufficient turgor pressure, plants wilt, losing their structural integrity.
Plasmolysis: When Cells Shrink
Plasmolysis occurs when a plant cell is placed in a hypertonic environment, meaning the water potential outside the cell is lower (more negative) than inside.
In this situation, water moves out of the cell via osmosis, causing the protoplast (the cell's contents inside the plasma membrane) to shrink and pull away from the cell wall.
Solute potential plays a crucial role in preventing plasmolysis. By maintaining a sufficiently negative solute potential within the cell, water is retained, counteracting the osmotic pressure exerted by the external environment.
This preventative action ensures that the cell remains turgid and functional.
Water Uptake: The Root of Plant Life
Plants obtain water from the soil through their roots, a process heavily reliant on water potential gradients. Solute potential within root cells is deliberately maintained at a lower (more negative) level than that of the surrounding soil water. This difference in water potential creates a driving force, pulling water into the root cells via osmosis.
The water then moves from cell to cell towards the xylem, the plant's vascular tissue responsible for water transport throughout the plant.
The ability of plants to regulate their solute potential in root cells is therefore essential for efficient water uptake from the soil.
Water Potential and Overall Plant Health
Ultimately, the interplay between solute potential, pressure potential, and the resulting water potential dictates the overall health and function of plant cells. Water potential affects numerous processes, including:
- Nutrient uptake: Water acts as a solvent for essential nutrients, facilitating their transport into and throughout the plant.
- Photosynthesis: Water is a key reactant in photosynthesis, the process by which plants convert light energy into chemical energy.
- Transpiration: The evaporation of water from plant leaves helps regulate temperature and drives the movement of water from the roots to the shoots.
By understanding and managing the solute potential within plant cells, we gain valuable insights into optimizing plant growth, improving crop yields, and developing strategies for drought resistance.
Real-World Applications and Examples: Optimizing Plant Growth
Having explored the theoretical underpinnings of solute potential and its significance within biological systems, particularly concerning plant cells and water uptake, it's time to shift our focus to the practical implications of this knowledge. How does solute potential actually impact plant growth and survival in the diverse environments they inhabit? And, crucially, how can we leverage our understanding of solute potential, and tools like solute potential calculators, to optimize plant growth, especially in controlled environments?
Case Studies: Solute Potential in Action
The influence of solute potential manifests differently across various ecosystems, each presenting unique challenges and opportunities for plant life. Let's examine a few illustrative case studies.
Saline Environments: The Struggle for Water
Consider plants thriving in saline soils, such as coastal marshes or arid regions affected by saltwater intrusion. These environments are characterized by high concentrations of dissolved salts, resulting in a highly negative solute potential in the soil water.
For plants to survive, they must maintain a solute potential even more negative than that of their surroundings. This allows them to draw water into their roots against the osmotic gradient. Halophytes, or salt-tolerant plants, achieve this through various mechanisms, including accumulating compatible solutes (organic compounds that do not interfere with cellular processes) in their cells.
By measuring the solute potential of both the soil and the plant tissues, scientists can assess the plant's ability to cope with saline stress and identify strategies for improving its salt tolerance, such as selective breeding or the application of soil amendments.
Drought Conditions: Managing Water Loss
In arid and semi-arid environments, water scarcity is the primary constraint on plant growth. Plants must employ strategies to minimize water loss through transpiration and maximize water uptake from the soil.
The solute potential of the soil water plays a critical role in determining the availability of water to plants. During prolonged drought, the soil solute potential can become increasingly negative as water evaporates and salts accumulate.
Plants adapted to drought conditions, known as xerophytes, often exhibit a range of adaptations to maintain turgor pressure and prevent water loss, including deep root systems, reduced leaf surface area, and the ability to accumulate solutes in their cells.
Understanding the relationship between soil solute potential, plant solute potential, and transpiration rates is crucial for developing effective irrigation strategies and selecting drought-tolerant plant species.
The Impact of Fertilizers on Solute Potential
Even in managed agricultural systems, solute potential can significantly impact plant health. Over-fertilization, for instance, can dramatically lower the solute potential of the soil solution. This makes it difficult for plants to absorb water, leading to fertilizer burn – a condition where leaves brown and die due to dehydration.
Careful monitoring of fertilizer application rates and soil solute potential is crucial for preventing this issue and ensuring optimal plant growth.
Optimizing Plant Growth in Controlled Environments
Solute potential calculators prove invaluable in controlled environments like greenhouses and hydroponic systems, where environmental factors can be precisely manipulated.
Hydroponics: Precision Nutrient Management
In hydroponic systems, plants are grown without soil, relying solely on nutrient solutions for their water and mineral requirements. The solute potential of the nutrient solution directly influences water uptake by the plants.
By using a solute potential calculator, growers can fine-tune the concentration of nutrients in the solution to optimize water uptake and promote healthy growth.
Maintaining the ideal solute potential prevents both water stress (from too high a concentration) and nutrient deficiencies (from too low a concentration), maximizing yield and quality.
Greenhouses: Controlling Environmental Stress
Greenhouses provide a controlled environment where temperature, humidity, and light levels can be carefully regulated. However, even in greenhouses, solute potential can be a limiting factor.
For example, during periods of high temperature or low humidity, plants may experience water stress due to increased transpiration rates. By monitoring the solute potential of the growing medium and adjusting irrigation practices accordingly, growers can mitigate the effects of environmental stress and maintain optimal plant growth.
Furthermore, solute potential calculators can assist in selecting appropriate fertilizer formulations and application rates to meet the specific needs of different plant species.
In conclusion, a thorough understanding of solute potential and its influence on plant water relations is essential for optimizing plant growth and survival in both natural and managed environments. By leveraging tools like solute potential calculators, scientists and growers can make informed decisions that promote plant health, increase crop yields, and ensure sustainable agricultural practices.
Real-world applications highlight the power of understanding solute potential, but it's equally crucial to acknowledge the potential pitfalls that can arise during calculations. Inaccurate solute potential values can lead to flawed interpretations and ultimately, misguided conclusions about plant health and growth strategies. Therefore, let’s address common mistakes and provide troubleshooting tips to ensure accurate results when working with solute potential and solute potential calculators.
Common Mistakes and Troubleshooting: Ensuring Accurate Calculations
Calculating solute potential, while seemingly straightforward with the right tools, is prone to errors if attention isn't paid to detail. These errors can stem from incorrect unit conversions, misunderstandings of the Van't Hoff factor, or simply misinterpreting the calculator's output. Let's examine these common pitfalls and learn how to avoid them, ensuring the reliability of your solute potential calculations.
The Unit Conversion Conundrum
One of the most frequent sources of error in solute potential calculations lies in the incorrect use of units. The solute potential equation relies on specific units for concentration and temperature, and deviations from these can lead to significant inaccuracies.
Molarity (Concentration): Molarity, represented as moles of solute per liter of solution (mol/L), is the standard unit for concentration in the solute potential equation. Always verify that your concentration values are expressed in molarity. If given in grams per liter (g/L) or parts per million (ppm), conversion to molarity is essential before proceeding with the calculation. Neglecting this step will undoubtedly lead to an incorrect result.
Temperature (Kelvin): Temperature must be expressed in Kelvin (K) for the solute potential equation to be valid. Celsius (°C) is a common temperature scale, but it needs conversion to Kelvin. The conversion formula is simple: K = °C + 273.15. Failing to convert Celsius to Kelvin will introduce a substantial error into the calculated solute potential, as temperature directly influences molecular movement and osmotic pressure.
Demystifying the Van't Hoff Factor
The Van't Hoff factor (i) accounts for the dissociation of solutes in a solution. It represents the number of particles into which a solute dissociates. For example, NaCl (sodium chloride) dissociates into two ions (Na+ and Cl-) in water, so its Van't Hoff factor is ideally 2. However, not all compounds dissociate completely.
Understanding Dissociation: Substances like glucose or sucrose do not dissociate in water; their Van't Hoff factor is 1. Strong electrolytes like NaCl, KCl, and MgCl2 will have Van't Hoff factors close to their theoretical values (2, 2, and 3, respectively). Weak electrolytes will have values between 1 and their theoretical maximum, depending on the degree of dissociation.
Experimental Determination vs. Theoretical Values: In real-world scenarios, the actual Van't Hoff factor may deviate slightly from its theoretical value due to ion pairing and other interactions in the solution. For precise calculations, especially in research settings, it's best to determine the Van't Hoff factor experimentally using techniques like colligative property measurements. However, for many practical applications, using the theoretical value is sufficiently accurate.
Applying the Van't Hoff Factor Correctly: When using a solute potential calculator, ensure that you're using the correct Van't Hoff factor for the specific solute you are working with. Incorrect application of the Van't Hoff factor can lead to a significant underestimation or overestimation of the solute potential.
Interpreting the Solute Potential Calculator Output
A solute potential calculator can provide a numerical value, but understanding what that value means is crucial. Solute potential is always a negative value (or zero for pure water), reflecting the decrease in water potential due to the presence of solutes.
Magnitude Matters: The more negative the solute potential, the lower the water potential and the greater the tendency of water to move into that solution. A plant cell with a very negative solute potential will readily draw water from its surroundings.
Context is Key: The calculated solute potential value should always be interpreted in the context of the environment being studied. For example, a plant cell in a saline environment needs to have a more negative solute potential than its surroundings to maintain water uptake. Comparing the solute potential of the plant tissue to the solute potential of the soil is essential for understanding the plant's water relations.
Troubleshooting Unexpected Results: If the calculated solute potential value seems unusual or doesn't align with your expectations, re-examine your input values. Double-check the units, the Van't Hoff factor, and the concentration. A small error in any of these parameters can propagate through the calculation and lead to a misleading result. Additionally, consider the limitations of the solute potential equation, which assumes ideal conditions. In complex biological systems, other factors like pressure potential and matric potential can also influence water potential.
Real-world applications highlight the power of understanding solute potential, but it's equally crucial to acknowledge the potential pitfalls that can arise during calculations. Inaccurate solute potential values can lead to flawed interpretations and ultimately, misguided conclusions about plant health and growth strategies. Therefore, let’s address common mistakes and provide troubleshooting tips to ensure accurate results when working with solute potential and solute potential calculators.
Advanced Topics: The Interplay of Water Potential, Solute Potential, and Osmosis
Beyond the basics, lies a deeper understanding of solute potential and its far-reaching implications. Solute potential doesn't exist in isolation. It's intimately connected to water potential and osmosis, driving critical biological processes. Let’s explore these advanced concepts.
Water Potential: A Holistic View of Water Movement
Water potential is the grand overseer of water movement in biological systems. It's the sum of all factors influencing water's tendency to move from one area to another. Solute potential is a key component.
Other factors, such as pressure potential (physical pressure on the solution) and matric potential (water's attraction to surfaces), also play significant roles.
The equation for water potential (Ψ) is:
Ψ = Ψs + Ψp + Ψm
Where:
- Ψs = Solute potential
- Ψp = Pressure potential
- Ψm = Matric potential
In most plant physiology scenarios, matric potential is often negligible, so the equation simplifies to:
Ψ = Ψs + Ψp
Understanding this relationship is key to predicting water's direction. Water always moves from areas of high water potential to areas of low water potential.
Solute Potential’s Influence on Water Potential
A lower (more negative) solute potential decreases the overall water potential. This creates a gradient that encourages water to move into the area with the lower water potential. This is what happens when water is absorbed by plant roots from the soil. The higher solute concentration in the root cells, due to dissolved solutes, drives water uptake.
Pressure Potential: Turgor Pressure and Beyond
Pressure potential can be positive or negative. Positive pressure potential, also known as turgor pressure in plant cells, helps maintain cell rigidity. In contrast, negative pressure potential (tension) can occur in the xylem of plants, aiding in water transport to the leaves.
The interplay between solute potential and pressure potential determines the direction and rate of water movement, profoundly impacting plant physiology.
Osmosis in Complex Biological Systems
Osmosis, driven by water potential differences, is fundamental to many biological processes. Its influence extends far beyond simple diffusion across a membrane.
Osmoregulation in Aquatic Organisms
Aquatic organisms face the constant challenge of maintaining internal water balance. Osmoregulation, the active regulation of osmotic pressure, is crucial for their survival.
Freshwater fish, for example, live in a hypoosmotic environment (lower solute concentration than their internal fluids). They constantly gain water and lose solutes. To counteract this, they excrete large amounts of dilute urine and actively absorb ions from the water.
Marine fish, on the other hand, live in a hyperosmotic environment (higher solute concentration than their internal fluids). They tend to lose water and gain solutes. To compensate, they drink seawater, excrete excess salt through their gills, and produce small amounts of concentrated urine.
Kidney Function and Water Reabsorption
The human kidney provides another excellent example of osmosis in a complex system. Water reabsorption in the kidneys is heavily reliant on osmotic gradients created by the movement of ions (solutes).
The loop of Henle, a key structure in the nephron, establishes a concentration gradient in the kidney medulla. This gradient draws water out of the collecting duct, concentrating the urine and conserving water. The hormone ADH (antidiuretic hormone) regulates the permeability of the collecting duct to water. This fine-tunes water reabsorption based on the body's hydration status.
Guttation
Guttation is another interesting biological phenomenon. This is when xylem sap is exuded through pores on the edges of leaves, typically at night when transpiration is low.
This occurs when root pressure, generated by solute accumulation in the roots, forces water upwards. When transpiration is minimal, this pressure can become so great that water is pushed out of the leaves through specialized structures called hydathodes.
Guttation showcases the power of solute potential and root pressure in driving water movement, even against the force of gravity.
Video: Master Solute Potential: Guide + Calculator for Perfect Data
FAQs About Mastering Solute Potential
Here are some frequently asked questions about solute potential and how to best use it for accurate data analysis.
What exactly is solute potential?
Solute potential, also known as osmotic potential, is the reduction in water potential due to the presence of solutes. Basically, the more dissolved substances in a solution, the more negative the solute potential, and the more likely water will move into that solution.
How does the solute potential calculator help me?
The solute potential calculator simplifies the process of determining the solute potential of a solution. By inputting the necessary variables (ionization constant, molar concentration, and pressure constant) along with the temperature, it provides an accurate solute potential value, saving you time and reducing the chance of calculation errors.
Why is accurately determining solute potential important?
Accurate solute potential calculations are critical for understanding water movement in biological systems, especially in plants. Inaccurate data can lead to misinterpretations of experimental results, impacting research in fields like agriculture and plant physiology.
What units should I use with the solute potential calculator?
To ensure accuracy with the solute potential calculator, use the following units: Molar concentration should be in mol/L, temperature in Kelvin (K), and the pressure constant is typically 0.00831 L MPa/mol K. Remember to convert values to these units before using the calculator.