Irradiance Units: Solar Energy Guide & Conversion
Understanding solar energy systems necessitates a firm grasp of irradiance units, where the watt per square meter (W/m²) serves as the standard measurement within the International System of Units (SI). Accurately measuring this incident solar radiation is crucial, whether for optimizing photovoltaic panel placement or estimating the power output of concentrating solar power plants near locations like the Mojave Desert. Devices such as pyranometers, frequently employed by organizations like the National Renewable Energy Laboratory (NREL), facilitate the precise quantification of these irradiance units, aiding engineers and researchers in evaluating solar resource availability. These measurements ultimately inform the calculations performed using tools like PVsyst to predict energy production.
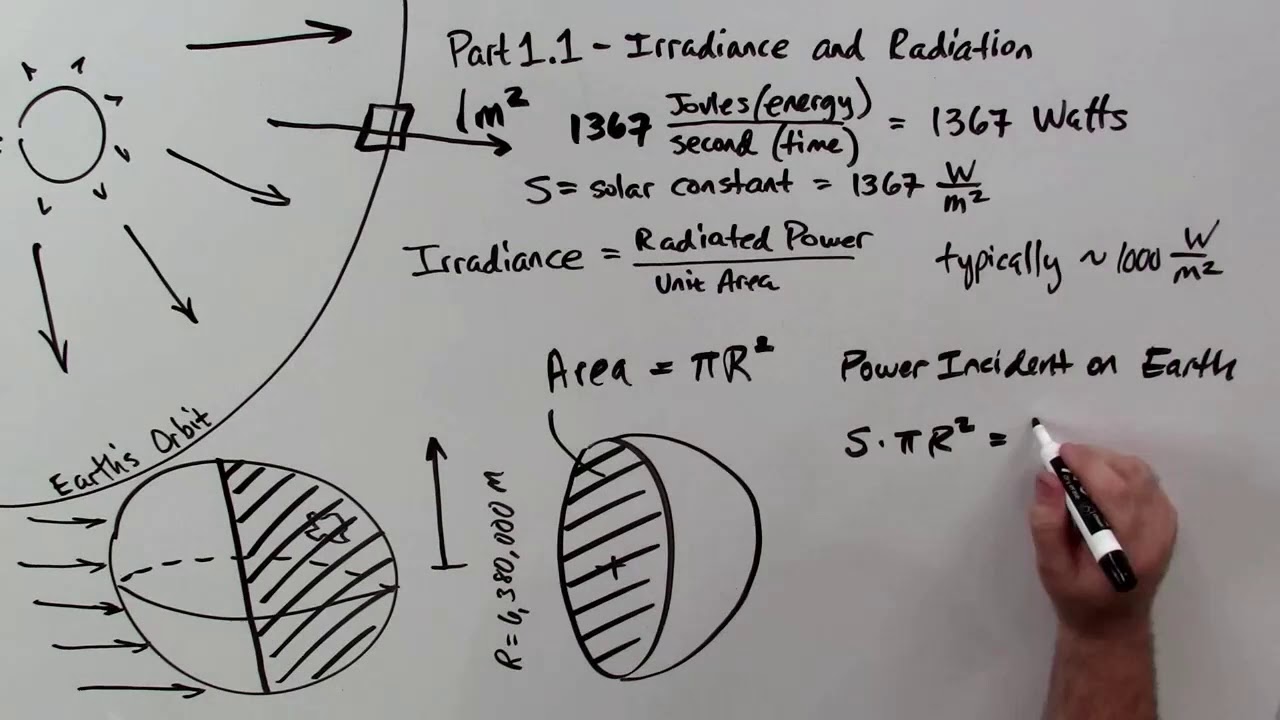
Image taken from the YouTube channel Justice and Mercy Energy Services , from the video titled Solar Radiance and Irradiance .
Irradiance, at its core, quantifies the power of light. It's a critical concept in understanding how energy, in the form of electromagnetic radiation, interacts with surfaces. This section will unpack its definition, relevance, and practical implications.
Defining Irradiance (E)
Irradiance (E) is formally defined as the radiant flux received by a surface per unit area. Radiant flux, measured in Watts (W), represents the total power of electromagnetic radiation. Therefore, irradiance is expressed in Watts per square meter (W/m²).
Think of it as the density of light power hitting a specific spot. A higher irradiance value means more light energy is concentrated on that surface.
The Broad Significance of Irradiance
The importance of irradiance spans numerous scientific and technological domains:
-
Solar Energy: Irradiance directly dictates the performance of solar panels, determining how much electricity they generate. Understanding solar irradiance patterns is key to optimizing the placement and efficiency of photovoltaic systems.
-
Climate Science: Variations in solar irradiance reaching Earth influence global temperatures and climate patterns. Measuring and modelling these variations are crucial for climate change research.
-
Agriculture: Light intensity, characterized by irradiance, is fundamental for photosynthesis. Farmers use irradiance data to optimize crop growth in greenhouses and open fields.
-
Material Science: Exposure to high irradiance levels, particularly ultraviolet (UV) radiation, can degrade materials over time. Understanding irradiance helps develop durable materials and protective coatings.
-
Human Health: UV irradiance impacts human skin, leading to vitamin D synthesis, sunburn, or skin cancer. Public health advisories regarding sun exposure rely on irradiance levels.
A Glimpse of What's to Come
Later sections will delve into the specific types of irradiance, such as Global Horizontal Irradiance (GHI), Direct Normal Irradiance (DNI), and Diffuse Horizontal Irradiance (DHI).
We'll also explore the fundamental physical quantities related to irradiance, including radiant flux, radiant energy, and the solar constant. Furthermore, we will examine the spectral dimension of irradiance and its relationship to wavelength.
Finally, we'll cover the units of measurement used to quantify irradiance and the instrumentation employed to measure it accurately.
Practical Implications: Solar Panel Efficiency
Consider a solar panel with a surface area of 1 square meter. If the irradiance on that panel is 1000 W/m², it means that 1000 Watts of solar power are striking that panel's surface.
However, the panel won't convert all that power into electricity. The efficiency of the panel, say 20%, determines how much of that 1000 W/m² is actually converted into usable electrical power. In this case, the panel would produce 200 Watts of electricity (1000 W/m² * 20% = 200 W).
Higher irradiance translates directly into higher power output, given a constant efficiency. Therefore, understanding and maximizing irradiance are crucial for optimizing solar energy generation.
Delving into Different Types of Irradiance
Irradiance, at its core, quantifies the power of light. It's a critical concept in understanding how energy, in the form of electromagnetic radiation, interacts with surfaces. This section will unpack its definition, relevance, and practical implications.
To effectively utilize solar energy and model atmospheric processes, it's essential to dissect irradiance into its fundamental components. Each component represents a distinct pathway by which solar radiation reaches the Earth's surface, influencing energy systems and environmental dynamics.
Solar Irradiance: The Source
Solar irradiance, fundamentally, is the irradiance that originates directly from the sun. It is the baseline energy input for our planet, driving weather patterns, sustaining life, and offering a vast potential source of renewable energy.
Without it, the other irradiance components would not exist. Understanding its magnitude and variations is paramount in any solar energy or climate-related analysis.
Global Horizontal Irradiance (GHI): Total Solar Input
Global Horizontal Irradiance (GHI) is a critical measurement in solar energy assessment. It represents the total solar irradiance received on a horizontal surface. This includes both the direct beam radiation from the sun and the diffuse radiation scattered by the atmosphere.
GHI is pivotal for assessing the potential of ground-mounted solar installations. Knowing the total energy available on a horizontal plane allows for informed decisions about the size and placement of photovoltaic (PV) arrays.
Factors Influencing GHI
Several factors dynamically influence GHI, leading to variations throughout the day and across seasons.
Cloud cover is a primary modulator, significantly reducing GHI by absorbing and scattering incoming solar radiation.
Atmospheric conditions, such as aerosols and humidity, also play a crucial role in attenuating solar radiation.
The time of day and season are also major determinants, affecting the angle of the sun and the length of daylight hours. These variations must be carefully considered for accurate solar energy predictions.
Direct Normal Irradiance (DNI): The Concentrated Power
Direct Normal Irradiance (DNI) refers to the amount of solar radiation received directly from the sun's disk. It is measured on a surface that is always perpendicular (normal) to the sun's rays.
DNI is particularly significant for Concentrated Solar Power (CSP) systems. These systems use mirrors or lenses to focus sunlight onto a receiver, generating heat for electricity production.
The Importance of Clear Skies for DNI
The effectiveness of CSP systems relies heavily on high DNI values.
Clear skies and minimal atmospheric scattering are essential for maximizing the amount of sunlight that can be focused. Cloud cover and aerosols can drastically reduce DNI, impairing the performance of CSP plants.
Locations with consistently high DNI, such as deserts, are therefore highly suitable for CSP deployment.
Diffuse Horizontal Irradiance (DHI): Scattered Light
Diffuse Horizontal Irradiance (DHI) is the component of solar radiation that reaches the Earth's surface after being scattered by the atmosphere. This scattering is caused by clouds, aerosols, air molecules, and other atmospheric particles.
Unlike DNI, which comes directly from the sun, DHI arrives from all directions in the sky.
The Path of DHI
The scattering process redirects sunlight in various directions. A portion of this scattered light eventually reaches the ground as DHI.
This component is crucial in situations where direct sunlight is blocked or limited.
DHI's Role in Shaded and Cloudy Conditions
DHI provides illumination in shaded areas and on cloudy days. It allows for some level of solar energy production even when direct sunlight is unavailable.
While DHI alone may not be sufficient to power high-energy applications, it contributes to the overall solar energy potential of a location. Understanding and quantifying DHI is vital for comprehensive solar resource assessment.
Fundamental Physical Quantities Related to Irradiance
Irradiance, at its core, quantifies the power of light. It's a critical concept in understanding how energy, in the form of electromagnetic radiation, interacts with surfaces. This section will unpack its definition, relevance, and practical implications.
To fully grasp the nuances of irradiance, it's essential to understand the underlying physical quantities that define and influence it. These fundamental concepts provide the theoretical bedrock upon which irradiance measurements and analyses are built.
Radiant Flux (Φ): The Power of Electromagnetic Radiation
Radiant flux (Φ), often referred to as radiant power, is the measure of the total power of electromagnetic radiation emitted, transmitted, or received. It's quantified in Watts (W), representing the energy transferred per unit time.
Think of it as the "flow rate" of light energy. Irradiance, then, is simply radiant flux distributed over a specific area.
The relationship is straightforward: irradiance (E) equals radiant flux (Φ) divided by area (A), expressed as E = Φ/A. Understanding radiant flux is crucial because it quantifies the total electromagnetic power involved, regardless of the area it illuminates.
Radiant Energy (Q): The Total Energy Carried by Light
Radiant energy (Q) represents the total energy of electromagnetic radiation. It's measured in Joules (J), the standard unit of energy. Radiant energy is directly related to radiant flux.
Specifically, radiant flux is the rate at which radiant energy is transferred or emitted. Mathematically, radiant flux (Φ) is the derivative of radiant energy (Q) with respect to time (t): Φ = dQ/dt.
Consider a light bulb: radiant energy is the total amount of light energy it emits, while radiant flux is how quickly it emits that energy (its power).
Solar Radiation: The Sun's Energy Spectrum
Solar radiation refers to the electromagnetic radiation emitted by the sun. This radiation spans a broad spectrum of wavelengths, ranging from high-energy ultraviolet (UV) and visible light to lower-energy infrared (IR) radiation.
Understanding the spectral distribution of solar radiation is critical for various applications, including solar energy conversion, climate modeling, and atmospheric studies. The intensity and composition of solar radiation vary depending on factors such as atmospheric conditions, solar angle, and time of year.
Solar Constant: Irradiance at the Edge of Our Atmosphere
The solar constant is defined as the average solar irradiance received at the top of Earth's atmosphere. Its value is approximately 1361 W/m².
This figure represents the maximum possible solar irradiance before atmospheric absorption and scattering occur. It's a vital parameter for climate models and calculations of solar energy potential.
Variability of the Solar Constant
It's important to note that the "constant" isn't perfectly constant.
Solar activity, such as sunspots and solar flares, can cause slight variations in the solar constant over time. These variations, while relatively small, can still have a noticeable impact on Earth's climate system.
Air Mass (AM): Atmospheric Path Length
Air mass (AM) represents the relative path length that sunlight travels through the atmosphere to reach a specific location on Earth's surface. It quantifies how much atmosphere the sunlight has to penetrate.
A higher air mass value indicates a longer path length and, consequently, greater atmospheric absorption and scattering of solar radiation.
AM0 and AM1.5: Standard Reference Points
AM0 (Air Mass 0) refers to the solar spectrum outside the Earth's atmosphere. It represents the solar radiation before any atmospheric attenuation.
AM1.5 is a standard reference spectrum used in solar energy research and testing. It corresponds to a solar zenith angle of 48.2 degrees, representing typical mid-latitude conditions.
Impact of Air Mass on Irradiance
As air mass increases, the intensity of solar radiation decreases. This is due to increased absorption by atmospheric gases (such as ozone and water vapor) and increased scattering by air molecules and aerosols.
Understanding air mass is crucial for accurately modeling and predicting solar energy availability at different locations and times.
The Spectral Dimension: Understanding Irradiance by Wavelength
Fundamental Physical Quantities Related to Irradiance. Irradiance, at its core, quantifies the power of light. It's a critical concept in understanding how energy, in the form of electromagnetic radiation, interacts with surfaces. This section will unpack its definition, relevance, and practical implications.
To fully grasp the nuances of irradiance, we must consider its spectral distribution. This means examining how irradiance is distributed across different wavelengths of electromagnetic radiation. Understanding this spectral dimension unlocks deeper insights into its interaction with various materials and processes.
Wavelength: The Key to Spectral Irradiance
Wavelength (λ) is a fundamental property of electromagnetic radiation, representing the distance between two successive crests or troughs of a wave. It's measured in units of length, such as nanometers (nm) or micrometers (µm). The wavelength of light directly determines its energy and color, influencing how it interacts with matter.
Regions of the Solar Spectrum
The solar spectrum, the range of electromagnetic radiation emitted by the sun, is conventionally divided into three primary regions based on wavelength:
-
Ultraviolet (UV): Encompassing wavelengths from approximately 100 nm to 400 nm. UV radiation is further subdivided into UV-A (315-400 nm), UV-B (280-315 nm), and UV-C (100-280 nm). UV radiation is energetic and can be harmful to living organisms, causing sunburn and increasing the risk of skin cancer. Atmospheric ozone strongly absorbs UV-B and UV-C radiation, protecting the Earth's surface.
-
Visible: Spanning wavelengths from approximately 400 nm to 700 nm. This is the range of light that the human eye can perceive. Different wavelengths within the visible spectrum correspond to different colors, ranging from violet (shortest wavelength) to red (longest wavelength).
-
Infrared (IR): Encompassing wavelengths from approximately 700 nm to 1 mm. IR radiation is associated with heat. Objects emit IR radiation based on their temperature. IR radiation is used in applications such as thermal imaging and remote sensing.
Spectrum: Irradiance's Fingerprint
The spectrum is essentially a "fingerprint" of irradiance, showing how much energy is present at each wavelength. It's a graphical representation of spectral irradiance as a function of wavelength. Analyzing the spectrum provides critical information about the source of the radiation and its potential effects.
Variability of Spectral Irradiance
Spectral irradiance is not constant. It varies depending on several factors, including:
-
Solar Angle: The angle of the sun in the sky affects the path length of sunlight through the atmosphere. At lower solar angles (e.g., sunrise or sunset), sunlight travels through more of the atmosphere, leading to increased absorption and scattering of shorter wavelengths (blue light). This explains why sunsets appear red.
-
Atmospheric Conditions: Clouds, aerosols, and other atmospheric constituents can absorb and scatter solar radiation, altering its spectral composition. For example, clouds tend to scatter shorter wavelengths more effectively, reducing the amount of blue light reaching the surface on cloudy days.
Applications of Spectral Irradiance Knowledge
Understanding spectral irradiance has profound implications across a wide range of disciplines:
-
Plant Growth (Photosynthetically Active Radiation - PAR): PAR refers to the portion of the solar spectrum (typically 400-700 nm) that plants use for photosynthesis. Measuring PAR is crucial for optimizing plant growth in agriculture and horticulture. Different plants have different spectral sensitivities, so tailoring the light spectrum can improve yields.
-
Materials Testing (UV Degradation): UV radiation can cause degradation in many materials, including plastics, polymers, and coatings. By analyzing the UV portion of the spectrum, engineers can predict the lifespan of materials exposed to sunlight and develop more durable products. Accelerated weathering tests use controlled UV exposure to simulate long-term degradation in a short period.
-
Photovoltaic (PV) Cell Design: The efficiency of a solar cell depends on how well it can absorb different wavelengths of light. By understanding the spectral irradiance of sunlight, engineers can design solar cells that are optimized for maximum energy conversion. Different materials are used to absorb different parts of the spectrum, and multi-junction solar cells are designed to capture a wider range of wavelengths.
In conclusion, the spectral dimension of irradiance provides a crucial layer of information that is essential for understanding light-matter interactions across diverse applications. By analyzing the spectral distribution of irradiance, we can gain valuable insights and optimize various processes from agriculture to materials science.
Units of Measurement for Irradiance: A Practical Guide
The Spectral Dimension: Understanding Irradiance by Wavelength Fundamental Physical Quantities Related to Irradiance. Irradiance, at its core, quantifies the power of light. It's a critical concept in understanding how energy, in the form of electromagnetic radiation, interacts with surfaces. This section will unpack its definition, relevance, and... Before diving deeper into instrumentation and data analysis, it's crucial to understand the units used to express irradiance. Selecting the appropriate unit enhances comprehension and facilitates effective communication within specific fields.
The Standard: Watts per Square Meter (W/m²)
The watt per square meter (W/m²) is the standard SI unit for irradiance. It directly expresses the power of electromagnetic radiation incident on a surface area of one square meter. This unit is universally applicable, making it ideal for scientific research, engineering calculations, and general comparisons of irradiance levels across different contexts.
Its widespread adoption simplifies data interpretation and reduces the risk of errors associated with unit conversions. When analyzing solar energy potential or evaluating the performance of lighting systems, W/m² provides a clear and intuitive measure of light intensity.
Scaling Up: Kilowatts per Square Meter (kW/m²)
For high irradiance applications, such as concentrated solar power (CSP) or laser technology, kilowatts per square meter (kW/m²) becomes a more practical unit. One kilowatt is equal to one thousand watts, making kW/m² a convenient way to express large values without resorting to cumbersome scientific notation.
Using kW/m² allows for easier comparison of irradiance levels in high-energy systems. This unit maintains the same fundamental meaning as W/m², only scaled to better reflect the magnitude of power involved.
Smaller Scales: Milliwatts per Square Centimeter (mW/cm²)
While less common, milliwatts per square centimeter (mW/cm²) is still encountered in certain scientific and engineering disciplines. One milliwatt is equal to one-thousandth of a watt, and one square centimeter is equal to one ten-thousandth of a square meter.
This unit is particularly useful when dealing with small areas or relatively low-intensity light sources.
mW/cm² can be found in applications such as laser safety analysis, where precise measurements of low-level radiation exposure are critical.
Imperial Influence: BTU per Hour per Square Foot (BTU/hr/ft²)
The BTU per hour per square foot (BTU/hr/ft²) is a unit of power per unit area within the imperial system. It is most frequently used in the United States, particularly in fields related to building energy analysis, heating, ventilation, and air conditioning (HVAC).
Understanding this unit is essential for professionals working with older building systems or collaborating with engineers accustomed to imperial units. While the SI system is preferred in many contexts, BTU/hr/ft² remains relevant due to its historical prevalence and continued use in specific industries.
Historical Perspective: Langleys (ly)
The Langley (ly), named after Samuel Pierpont Langley, is a unit of energy per unit area that was historically used in solar radiation measurement. One Langley is defined as one calorie per square centimeter (1 cal/cm²).
While largely replaced by SI units, the Langley provides a valuable historical context for understanding the evolution of solar radiation measurement. To convert Langleys to W/m², one must consider the time period over which the energy was accumulated. Typically, daily solar radiation is reported in Langleys, requiring a conversion factor that accounts for the number of seconds in a day.
Understanding the Langley's historical significance sheds light on the past methods and practices. It also helps to interpret older datasets expressed in this unit.
Instrumentation for Measuring Irradiance: Tools of the Trade
Units of measurement provide the language for quantifying irradiance, but specific instruments are needed to actually capture and record these values. These tools are the backbone of data collection, enabling us to understand the radiant energy landscape around us. From the sun-tracking precision of a pyrheliometer to the broad-spectrum sensitivity of a pyranometer, each instrument plays a vital role.
This section delves into the key instruments used to measure irradiance, detailing their functionality and the principles behind their operation.
Pyranometers: Capturing Global Horizontal Irradiance (GHI)
Pyranometers are fundamental instruments for measuring global horizontal irradiance (GHI). GHI represents the total solar radiation received on a horizontal surface, encompassing both direct and diffuse components.
Working Principle: Thermopile Sensors
The core of most pyranometers is a thermopile sensor. This sensor converts thermal energy into an electrical signal. The sensor consists of a series of thermocouples, which generate a voltage proportional to the temperature difference between a black absorber and a reference point.
Incoming solar radiation is absorbed by the black surface, causing it to heat up. The temperature difference between this surface and the pyranometer body generates a voltage signal, which is then calibrated to provide an irradiance reading in W/m².
Pyrheliometers: Tracking Direct Normal Irradiance (DNI)
Pyrheliometers are specialized instruments designed to measure direct normal irradiance (DNI). Unlike pyranometers, they only capture the radiation coming directly from the sun's disk.
The Importance of a Narrow Field of View
A key characteristic of pyrheliometers is their narrow field of view. This is achieved through a collimating tube, which restricts the incoming radiation to a small solid angle centered on the sun.
This precise alignment is crucial for accurate DNI measurement, ensuring that diffuse radiation and radiation from surrounding areas are excluded.
Sun Tracking Systems
To maintain alignment with the sun, pyrheliometers are typically mounted on sun-tracking systems. These systems use motors and sensors to continuously adjust the instrument's position, compensating for the sun's movement across the sky.
Shade Ring Pyranometers: Isolating Diffuse Horizontal Irradiance (DHI)
Shade ring pyranometers are modified pyranometers used to measure diffuse horizontal irradiance (DHI). They are designed to block direct solar radiation, allowing only diffuse radiation to reach the sensor.
The Role of the Shade Ring
The shade ring is a semicircular band that is carefully positioned to block the direct beam of sunlight from reaching the pyranometer's sensor. The ring must be regularly adjusted to account for the sun's changing position throughout the year.
Calculating Diffuse Irradiance
By subtracting the DHI measured by the shade ring pyranometer from the GHI measured by a standard pyranometer, you can estimate the direct component of solar radiation. However, keep in mind, that DHI measurements from a shade ring pyranometer can be affected by the shade ring blocking some of the diffuse radiation as well.
Radiometers: A General Term
The term "radiometer" encompasses a broad range of instruments that measure electromagnetic radiation. While pyranometers and pyrheliometers are specialized types of radiometers, the term can also refer to instruments that measure radiation in other parts of the electromagnetic spectrum.
Spectroradiometers: Measuring Spectral Irradiance
Spectroradiometers go a step further than standard radiometers by measuring irradiance as a function of wavelength. This provides detailed information about the spectral composition of light.
Applications of Spectral Irradiance Data
Spectroradiometers are essential tools for characterizing light sources, analyzing atmospheric effects, and studying the interaction of light with materials. For example, they are used to measure the spectral output of solar simulators, assess the UV degradation of polymers, and monitor changes in atmospheric composition.
Data Loggers: Capturing and Storing Irradiance Data
Data loggers are electronic devices used to automatically record irradiance measurements over time. They are an integral part of any irradiance monitoring system.
The Importance of Long-Term Monitoring
Data loggers enable long-term monitoring of irradiance, providing valuable data for solar energy assessments, climate studies, and other applications. They also offer the capability to connect to a variety of sensors, and are often weather proof to ensure continuous operation in demanding climates.
Instrumentation for Measuring Irradiance: Tools of the Trade Units of measurement provide the language for quantifying irradiance, but specific instruments are needed to actually capture and record these values. These tools are the backbone of data collection, enabling us to understand the radiant energy landscape around us. From the sun-tracking precision of pyrheliometers to the broad-spectrum capture of pyranometers, each device offers a unique window into the world of solar radiation.
Data Resources and Software for Irradiance Analysis
The accurate measurement of irradiance is only the first step. To truly harness its potential, we must turn to sophisticated data resources and software tools capable of transforming raw data into actionable insights. These platforms allow us to model, simulate, and optimize energy systems, driving innovation across diverse sectors.
Publicly Accessible Irradiance Databases
The proliferation of publicly accessible irradiance databases has democratized access to crucial data, empowering researchers, engineers, and policymakers alike. These resources offer a wealth of historical and real-time data, enabling informed decision-making and accelerating the development of sustainable energy solutions.
PVGIS (Photovoltaic Geographical Information System)
PVGIS stands out as a leading platform for accessing solar radiation data and estimating PV performance. Developed by the European Commission, PVGIS offers a user-friendly interface and a comprehensive suite of tools tailored to the needs of the solar energy community.
Key Features of PVGIS
Mapping tools allow users to visualize solar radiation levels across Europe and Africa, identifying areas with high solar potential.
Time-series data provides hourly, daily, and monthly irradiance values for specific locations, enabling detailed performance analysis.
PV simulation capabilities allow users to model the performance of different PV systems, taking into account factors such as module type, orientation, and shading. The latest version incorporates climate change scenario considerations.
PVGIS is freely accessible and widely used for feasibility studies, site selection, and performance monitoring of PV installations.
SAM (System Advisor Model, NREL)
Developed by the National Renewable Energy Laboratory (NREL), SAM is a powerful software tool for modeling the performance and economics of renewable energy systems. While it's not limited to solar, it is a gold standard in the industry.
Key Features of SAM
Performance analysis enables users to simulate the energy production of solar PV, concentrating solar power (CSP), and other renewable energy systems.
Financial modeling allows users to evaluate the economic viability of projects, taking into account factors such as capital costs, operating expenses, and incentives.
Optimization tools help users identify the optimal system configuration and operating parameters to maximize energy production and minimize costs. SAM is especially valuable for project developers, investors, and researchers seeking to evaluate the financial viability of renewable energy projects.
It is well-regarded for its thoroughness, accuracy, and inclusion of financial considerations.
NASA POWER Project
The NASA POWER (Prediction Of Worldwide Energy Resources) Project provides access to a wealth of solar radiation and meteorological data derived from satellite observations.
Key Features of NASA POWER
Global coverage ensures that data is available for virtually any location on Earth.
Historical data availability extends back several decades, enabling long-term trend analysis and climate change studies. The POWER data is particularly valuable in areas with limited ground-based measurements.
Diverse data parameters include not only solar irradiance but also temperature, wind speed, and humidity, providing a comprehensive picture of the climate conditions affecting renewable energy systems.
Video: Irradiance Units: Solar Energy Guide & Conversion
FAQs: Irradiance Units
What is irradiance?
Irradiance is the power of electromagnetic radiation (like sunlight) per unit area striking a surface. It's a measure of how much solar energy is arriving at a particular location. Understanding irradiance units is crucial for solar energy calculations.
Why are there different units for irradiance?
Different units are used for irradiance depending on the context. Watts per square meter (W/m²) is common in scientific and engineering fields, while kilowatt-hours per square meter per day (kWh/m²/day) is more useful for estimating solar energy production over time. Knowing how to convert between these irradiance units is important.
How do you convert between W/m² and kWh/m²/day?
To convert average daily irradiance from W/m² to kWh/m²/day, multiply the W/m² value by 24 hours and then divide by 1000. This accounts for the continuous measurement and changes it to daily energy received. Correctly applying this conversion with irradiance units ensures accurate energy production estimates.
What factors affect solar irradiance values?
Several factors impact solar irradiance, including the time of day, season, latitude, and weather conditions. Cloud cover, atmospheric particles, and the angle of the sun relative to the surface all affect the amount of solar radiation reaching a specific location. These variations influence the specific irradiance units measured.
So, next time you're geeking out over solar panels or trying to figure out how much power your rooftop is pulling in, hopefully, you'll feel a bit more confident navigating the world of irradiance units! It's all about understanding the sun's power, and now you're a little closer to speaking its language.