Sodium Chloride: Electrical Conductivity Explained
The capacity of materials to conduct electrical current, primarily investigated using tools like a conductivity meter, is a cornerstone of modern science. Sodium chloride, a prevalent ionic compound, presents a unique case study in this domain due to its behavior under varying conditions. The National Institute of Standards and Technology (NIST) provides extensive data on material properties, including electrical conductivity, which is crucial in determining whether the electrical conductivity of sodium chloride exist or not. A deeper dive into electrolyte chemistry reveals that while solid sodium chloride is a poor conductor, its molten or aqueous forms readily facilitate ion transport, exhibiting significant electrical conductivity.
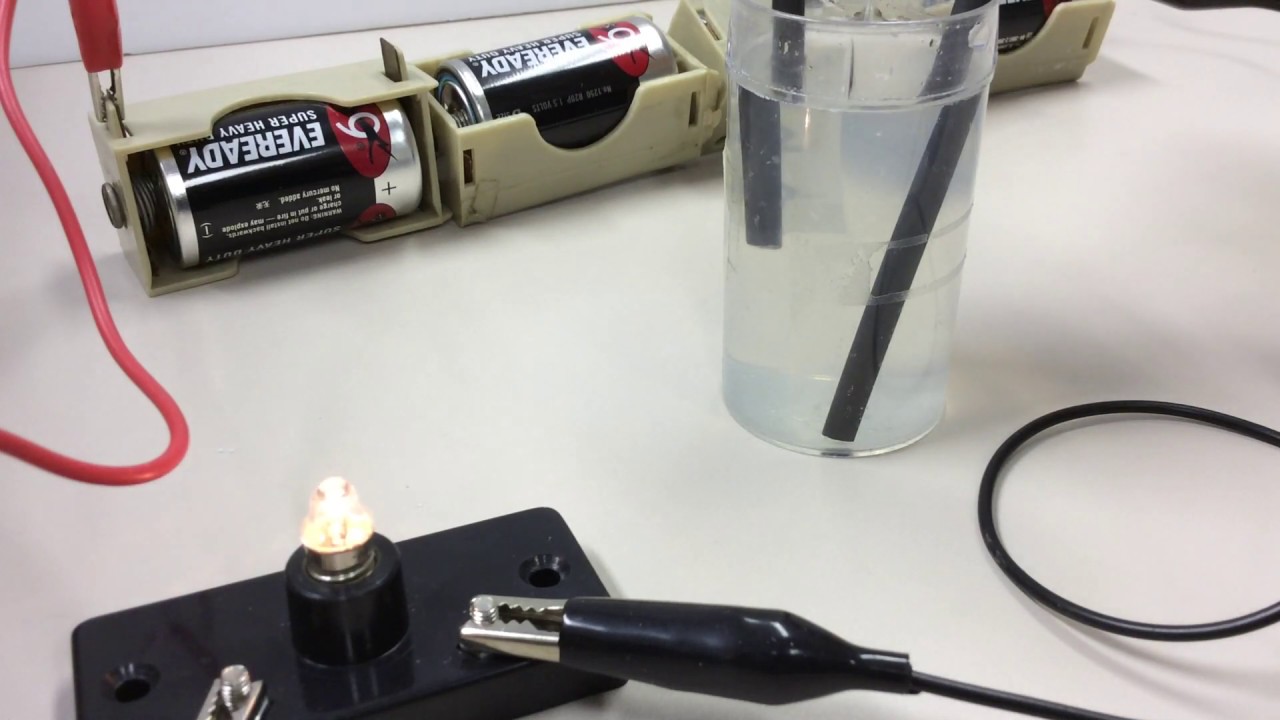
Image taken from the YouTube channel JH Science NJC , from the video titled Aqueous sodium chloride as an electrical conductor .
The capacity of a material to facilitate the flow of electric current, known as electrical conductivity, is a cornerstone property that dictates its behavior in electrical and electronic applications. Materials are broadly categorized based on their conductivity, ranging from highly conductive metals to insulating non-metals.
This exploration delves into the intriguing case of Sodium Chloride (NaCl), commonly known as table salt. While its ubiquitous presence in our daily lives is undeniable, its electrical properties are less straightforward.
Sodium Chloride: More Than Just Table Salt
Sodium Chloride (NaCl) is an ionic compound formed through the electrostatic attraction between positively charged sodium ions (Na+) and negatively charged chloride ions (Cl-). Its crystalline structure is well-defined, but its conductive behavior is significantly influenced by external conditions.
The Central Question: Conductivity Under What Circumstances?
The pivotal question we address is: Does Sodium Chloride conduct electricity?
And, if so, under what specific conditions does this conductivity manifest?
The answer is not a simple yes or no. The electrical conductivity of NaCl is highly dependent on its physical state.
Understanding these conditions is vital for comprehending the broader principles of ionic conductivity and its implications in various scientific and industrial applications. The subsequent sections will dissect the behavior of NaCl in its solid, aqueous, and molten states, elucidating the factors that govern its ability to conduct electricity.
Solid NaCl: An Insulator's Tale
The capacity of a material to facilitate the flow of electric current, known as electrical conductivity, is a cornerstone property that dictates its behavior in electrical and electronic applications. Materials are broadly categorized based on their conductivity, ranging from highly conductive metals to insulating non-metals.
This exploration delves into the intriguing question of why solid sodium chloride (NaCl), common table salt, stands as a poor conductor of electricity under ordinary conditions. To understand this, we must first examine the very architecture of its solid form – its crystal lattice structure – and the implications this structure holds for the mobility of charge carriers.
The Crystalline Order of Solid NaCl
At room temperature, solid NaCl exists as a highly ordered crystalline solid. This arrangement, known as a crystal lattice, is characterized by the precise and repeating arrangement of positively charged sodium ions (Na+) and negatively charged chloride ions (Cl-).
Each Na+ ion is surrounded by six Cl- ions, and each Cl- ion is, likewise, surrounded by six Na+ ions. This three-dimensional network is held together by strong electrostatic forces – the attraction between oppositely charged ions.
The consequence of this rigid, highly ordered arrangement is that the ions are essentially locked in place. They are not free to move about the structure.
Immobility: The Bane of Conductivity
Electrical conductivity relies fundamentally on the presence of mobile charge carriers – particles capable of transporting electrical charge through a material. In metals, these charge carriers are electrons. In ionic solutions, and as we will discuss later, molten salts, these charge carriers are ions themselves.
However, in solid NaCl, the ions are tightly bound within the crystal lattice.
They cannot readily migrate under the influence of an electric field. The strong electrostatic forces holding the lattice together far outweigh the forces that could be exerted by a typical applied voltage.
The Absence of Mobile Charge Carriers
Therefore, solid NaCl, at room temperature, lacks the crucial ingredient for electrical conductivity: mobile charge carriers. The ions, which are the potential carriers of charge, are immobilized by the crystal lattice.
Without freely moving charges, solid NaCl behaves as an electrical insulator, effectively blocking the flow of electric current. This insulating behavior is a direct consequence of its crystalline structure and the immobility of its constituent ions.
Aqueous NaCl: Conductivity in Solution
Having established the insulating properties of solid NaCl, it is crucial to examine its behavior when introduced into an aqueous environment. The dissolution of NaCl in water fundamentally alters its electrical characteristics, transforming it from a non-conductor to a conductive medium.
The Role of Water as a Solvent
Water's unique properties as a polar solvent are central to understanding the conductivity of aqueous NaCl. The polar nature of water molecules, characterized by a partial positive charge on the hydrogen atoms and a partial negative charge on the oxygen atom, allows them to interact strongly with ionic compounds like NaCl.
This interaction facilitates the dissociation of NaCl into its constituent ions: sodium cations (Na+) and chloride anions (Cl-).
Dissociation and the Formation of an Electrolyte
When NaCl is introduced into water, the polar water molecules surround the ions, effectively shielding them from their strong electrostatic attraction. This process, known as hydration, weakens the ionic bonds within the crystal lattice.
The energy gained from hydration overcomes the lattice energy holding the ions together.
As a result, the ions dissociate and disperse throughout the solution, forming an electrolyte.
An electrolyte is a solution containing free ions capable of conducting electricity.
Ionic Conductivity: Mechanism and Influencing Factors
The electrical conductivity of aqueous NaCl is attributed to ionic conductivity. Unlike metals, where electrons serve as charge carriers, in aqueous NaCl, the Na+ and Cl- ions are responsible for carrying the electrical charge.
When an electric field is applied across the solution, the positively charged Na+ ions migrate towards the cathode (negative electrode), while the negatively charged Cl- ions migrate towards the anode (positive electrode).
This movement of ions constitutes an electric current, thereby enabling the solution to conduct electricity.
Concentration's Impact
The concentration of NaCl in the aqueous solution significantly affects its conductivity. A higher concentration of NaCl translates to a greater number of ions available to carry charge.
Consequently, the conductivity generally increases with increasing concentration, up to a certain point.
Beyond a specific concentration threshold, the conductivity may plateau or even decrease due to ion-ion interactions that impede their mobility.
Temperature Dependence
Temperature also plays a crucial role in influencing the conductivity of aqueous NaCl. Higher temperatures increase the kinetic energy of the ions, enabling them to move more freely and rapidly through the solution.
This enhanced mobility translates to higher conductivity. Additionally, increased temperature may promote further dissociation of NaCl.
Leading to a greater number of charge carriers, further enhancing conductivity.
Molten NaCl: High-Temperature Conductivity
Having established the conductive properties of aqueous NaCl, a pertinent question arises: can NaCl conduct electricity without the presence of water? The answer lies in its behavior at elevated temperatures, where it transitions into a molten state. This transformation profoundly impacts its electrical properties, enabling it to conduct electricity through a mechanism distinct from that observed in aqueous solutions.
Overcoming Lattice Energy: The Key to Mobility
In its solid form, NaCl's ions are rigidly held in a crystal lattice, preventing any significant movement and hindering electrical conductivity. However, upon heating to its melting point (approximately 801°C), the energy supplied overcomes the electrostatic forces binding the ions, disrupting the lattice structure.
This process, driven by thermal energy, weakens the ionic bonds, allowing the ions to become mobile. This is a critical threshold for conductivity.
The high temperature requirement is not merely a matter of convenience; it is a fundamental aspect of NaCl's inherent chemical properties. It directly influences the ion's capacity to transition from a fixed point to a dynamic contributor in electrical conductance.
Ionic Conductivity in the Absence of Solvent
In the molten state, both sodium (Na+) and chloride (Cl-) ions are free to move throughout the liquid.
When an external electric field is applied, these ions respond by migrating towards the electrode of opposite charge. This movement of charged particles constitutes an electric current, rendering the molten NaCl conductive.
Unlike aqueous solutions where water facilitates ion separation and mobility, molten NaCl relies solely on thermal energy to achieve the necessary ionic mobility. The absence of a solvent introduces a new dynamic where ionic interaction relies on temperature-induced kinetic energy.
Applications of Molten Salt Electrolysis
The high-temperature ionic conductivity of molten NaCl finds crucial applications in industrial processes. Electrolysis, the process of using electric current to drive non-spontaneous chemical reactions, often employs molten salts like NaCl.
This method is essential in the production of chlorine gas and sodium metal, both of which are vital in various industrial sectors. The ability of molten NaCl to conduct electricity at high temperatures makes it an indispensable component in these processes, enabling efficient and cost-effective production.
The study and application of molten NaCl conductivity underscore the profound influence of temperature and phase transitions on material properties.
Key Factors Influencing NaCl Conductivity
Having established the conductive properties of molten NaCl, understanding the nuances of what influences the electrical conductivity becomes paramount. Two dominant factors emerge from solution: temperature and concentration, both playing critical roles in modulating the movement and availability of ions, the very carriers of electrical charge.
Temperature: A Catalyst for Ionic Mobility and Dissociation
Temperature exerts a dual influence on the conductivity of NaCl solutions. First and foremost, it directly affects ionic mobility.
As temperature rises, the kinetic energy of the ions increases, allowing them to move more freely through the solution. This heightened mobility translates into a more efficient transfer of charge, leading to a measurable increase in conductivity.
Secondly, temperature can also influence the dissociation of NaCl. Although NaCl is generally considered a strong electrolyte that fully dissociates in water, subtle shifts in the equilibrium can occur with temperature changes.
Higher temperatures may slightly favor increased dissociation, leading to a greater number of free ions available to conduct electricity. This effect is generally less pronounced than the direct impact on ionic mobility, but still contributes to the overall temperature dependence of conductivity.
Concentration: Finding the Sweet Spot
The relationship between NaCl concentration and conductivity is not linear. Initially, as the concentration of NaCl increases, so does the conductivity. This is a direct consequence of having more charge carriers (Na+ and Cl- ions) in the solution.
However, this positive correlation plateaus and eventually begins to decline at higher concentrations. This phenomenon can be attributed to several factors, including increased ion-ion interactions.
At high concentrations, the ions are packed more closely together, hindering their free movement due to increased electrostatic forces. This crowding effect reduces the effective mobility of the ions, thereby diminishing the overall conductivity.
Therefore, an optimal concentration exists where the balance between the number of charge carriers and their mobility is maximized. This concentration will vary depending on the specific solvent and temperature, but generally lies within a moderate range, avoiding both excessively dilute and highly concentrated solutions.
Measuring Conductivity: Tools and Techniques
Having established the conductive properties of NaCl under varying conditions, the practical question arises: how do we quantify this conductivity? The measurement of electrical conductivity in NaCl solutions, or any conductive material, relies primarily on the use of specialized instruments called conductivity meters. These devices, employing sophisticated electronic circuitry and carefully designed probes, offer a precise and reliable means of assessing the ability of a solution to conduct electrical current.
Understanding Conductivity Meters
A conductivity meter, at its core, is a device that measures the electrical resistance of a solution between two or more electrodes. From this measured resistance, and knowing the geometry of the electrode arrangement, the instrument calculates and displays the conductivity, typically expressed in Siemens per meter (S/m) or millisiemens per centimeter (mS/cm). Understanding the components and operational principles of these meters is crucial for accurate and meaningful measurements.
The Role of Electrodes
The electrodes are arguably the most critical component of a conductivity meter. They serve as the interface between the instrument and the solution under test, facilitating the passage of electrical current. Several types of electrodes are commonly used, each with its own advantages and limitations.
Common Electrode Types
-
Two-Electrode Cells: These are the simplest design, consisting of two conductive plates or cylinders immersed in the solution. A known voltage is applied across the electrodes, and the resulting current is measured. The conductivity is then calculated based on Ohm's Law.
However, two-electrode cells are susceptible to polarization effects, particularly at higher conductivities, which can lead to inaccuracies.
-
Four-Electrode Cells: To mitigate polarization effects, four-electrode cells are often preferred. In this configuration, two outer electrodes apply the current, while two inner electrodes measure the potential difference across a defined volume of the solution.
This eliminates the influence of electrode polarization on the voltage measurement, resulting in more accurate conductivity values.
-
Inductive (Electrodless) Sensors: For challenging applications involving highly conductive or corrosive solutions, inductive sensors offer a robust alternative. These sensors utilize two coils, one to induce a current in the solution and the other to measure the induced current.
Since the electrodes do not directly contact the solution, they are less prone to fouling and corrosion.
Electrode Materials
The choice of electrode material is also critical. Common materials include platinum, gold, stainless steel, and graphite.
- Platinum is often favored for its chemical inertness and high conductivity.
- Stainless steel offers a cost-effective alternative for less demanding applications.
- The selected material must be compatible with the solution being tested to avoid corrosion or contamination, which can significantly impact the accuracy of the measurements.
Performing Conductivity Measurements
To accurately measure the conductivity of a NaCl solution, the following steps are generally followed:
-
Calibration: The conductivity meter must be calibrated using standard solutions of known conductivity. This ensures the accuracy and reliability of the instrument.
-
Electrode Preparation: Electrodes must be clean and free of any contaminants. Rinse with distilled water before each measurement.
-
Measurement: Immerse the electrodes in the NaCl solution, ensuring they are fully submerged and that there are no air bubbles trapped. Allow the reading to stabilize before recording the conductivity value.
-
Temperature Compensation: Conductivity is temperature-dependent. Many conductivity meters have built-in temperature sensors and automatically compensate for temperature variations. If not, temperature should be carefully controlled.
By understanding the principles of conductivity measurement and employing proper techniques, reliable and meaningful data can be obtained, providing valuable insights into the behavior of NaCl solutions and their applications in diverse fields.
Applications and Real-World Relevance
Having established the conductive properties of NaCl under varying conditions, the practical question arises: how do we quantify this conductivity?
Beyond the laboratory, the electrical conductivity of sodium chloride is not merely an academic curiosity but a cornerstone of numerous industrial processes and electrochemical applications. Its ability to facilitate charge transport in aqueous and molten states makes it indispensable in several key sectors, impacting everything from chemical synthesis to energy storage.
The Foundation of Electrochemistry
The relevance of NaCl conductivity is profoundly intertwined with the principles of electrochemistry. Electrochemical reactions, which involve the transfer of electrons at an electrode-electrolyte interface, are fundamentally dependent on the presence of ions to carry charge.
NaCl, when dissolved in water, provides a rich source of these ions (Na+ and Cl-), forming an electrolytic solution capable of supporting electrochemical processes. Without the presence of such conductive electrolytes, many crucial chemical transformations would simply be impossible to achieve efficiently, if at all.
Electrolysis: Decomposing with Electricity
One of the most significant applications of NaCl conductivity lies in the realm of electrolysis. Electrolysis is the process of using electrical energy to drive non-spontaneous chemical reactions. It is through electrolysis that we can decompose NaCl into its constituent elements: sodium and chlorine.
The Chlor-Alkali Process
The chlor-alkali process, a cornerstone of the chemical industry, exemplifies this application. In this process, an aqueous solution of NaCl (brine) undergoes electrolysis to produce chlorine gas, hydrogen gas, and sodium hydroxide (caustic soda).
Chlorine is used in the production of PVC, disinfectants, and various other chemicals.
Sodium hydroxide finds applications in pulp and paper manufacturing, soap and detergent production, and water treatment.
The hydrogen gas produced can be used as a fuel source or in various industrial processes. The chlor-alkali process is a prime example of how the conductivity of NaCl enables the large-scale production of essential chemicals, underpinning numerous downstream industries.
Other Industrial Applications
Beyond the chlor-alkali process, NaCl conductivity plays a role in other industrial applications:
-
Metal Production: Electrolysis of molten NaCl is used in the production of metallic sodium.
-
Desalination: Electrochemical methods utilizing NaCl solutions are being explored for water desalination.
-
Batteries: While not a primary component in most battery chemistries, NaCl solutions can act as electrolytes in certain experimental battery designs.
The conductive properties of NaCl are critical to a vast array of industrial operations. These applications demonstrate NaCl's vital role in facilitating efficient and scalable chemical transformations, underpinning many modern manufacturing processes.
Video: Sodium Chloride: Electrical Conductivity Explained
Frequently Asked Questions
Why doesn't solid sodium chloride conduct electricity?
Solid sodium chloride (table salt) doesn't conduct electricity because its ions (Na+ and Cl-) are locked in a rigid crystal lattice. These ions are not free to move and carry an electrical charge. So, the electrical conductivity of sodium chloride does not exist in the solid state.
How does dissolving sodium chloride in water make it conductive?
When sodium chloride dissolves in water, it dissociates into free-moving sodium (Na+) and chloride (Cl-) ions. These charged ions can then move freely through the water, carrying an electrical current. This demonstrates how the electrical conductivity of sodium chloride manifests in an aqueous solution.
Does molten sodium chloride conduct electricity?
Yes, molten (melted) sodium chloride conducts electricity. Heating sodium chloride breaks the rigid crystal structure, freeing the Na+ and Cl- ions. These mobile ions can then carry an electric current. Thus, electrical conductivity of sodium chloride exists in the molten state.
Is the electrical conductivity of sodium chloride the same at all concentrations in water?
No, the electrical conductivity of sodium chloride in water varies depending on the concentration. Higher concentrations of NaCl mean more ions are present, which allows for greater charge flow. As the concentration increases, so does the ability of the solution to conduct electricity. Therefore electrical conductivity of sodium chloride is directly proportional to the amount of sodium chloride dissolved in water.
So, there you have it! Hopefully, this clears up any confusion you might've had about the electrical conductivity of sodium chloride. Remember, pure, solid salt doesn't conduct electricity because the ions are locked in place. But dissolve it in water, and boom – free-moving ions, ready to carry a charge. So, while solid NaCl itself isn't a conductor, it's all about the environment and the movement of those little ions to answer whether electrical conductivity of sodium chloride exists.