Sodium Charge Demystified: What You NEED to Know!
The electronegativity of elements influences the sodium charge within chemical compounds, a crucial factor explored by Linus Pauling in his groundbreaking work on chemical bonding. Understanding this charge is vital in fields like neuroscience, where the movement of sodium ions governs nerve impulses. Furthermore, accurate determination of sodium charge often relies on sophisticated simulation software, such as those provided by Gaussian, to model the complex interactions within molecules. By grasping these fundamental relationships, one can begin to demystify the behavior of sodium charge and its impact across various scientific disciplines.
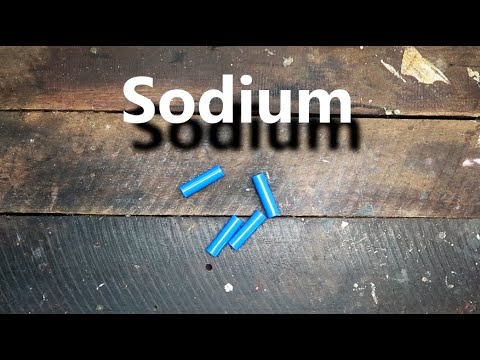
Image taken from the YouTube channel Off-Grid Garage , from the video titled Sodium Cell Charging and Discharging Analysis, Hardware Compatibility. Future or Niche product? .
Sodium. It's a word we hear often, whether we're discussing dietary guidelines, chemistry experiments, or even the vibrant glow of streetlights. But have you ever stopped to consider the fundamental nature of this ubiquitous element?
Sodium, represented by the symbol Na, is far more than just table salt (sodium chloride). It’s a cornerstone of both the physical and biological worlds, playing a vital role in countless processes.
Sodium: An Essential Element
From a chemical perspective, sodium is an alkali metal, a group known for its high reactivity. In biological systems, sodium ions are crucial for maintaining fluid balance, nerve impulse transmission, and muscle function.
Sodium's versatility stems from its electronic structure, specifically its tendency to readily lose an electron. But before we delve too deeply, consider this: sodium is so reactive that it bursts into flames when it comes into contact with water!
This dramatic reaction underscores the power locked within this seemingly simple element.
The Sodium Story: A Journey into Atomic Charge
This article embarks on a journey to unravel the secrets of sodium's charge. We'll explore its atomic structure, the electron configuration that dictates its behavior, and the process of ionization that leads to the formation of a positively charged sodium ion (Na+).
We will also examine how sodium's position in the periodic table relates to its reactivity and tendency to form ionic bonds. Finally, we will discuss the profound biological significance of the sodium ion, highlighting its role as a crucial electrolyte in living organisms.
Join us as we unpack the story behind sodium's charge and its far-reaching consequences.
Sodium's propensity to shed an electron hints at the deeper forces at play within the atom itself. To truly grasp why sodium readily becomes a positively charged ion, we need to understand the fundamental concept of electric charge and its influence on atomic behavior.
Charge: The Foundation of Atomic Interactions
At the heart of matter lies electric charge, an intrinsic property that governs how particles interact with electromagnetic fields. In the context of atoms, it's the balance between positive and negative charges that dictates stability and reactivity.
Defining Charge Within the Atomic Realm
Charge, in its most basic form, is the physical property that causes a particle to experience a force when placed in an electromagnetic field.
There are two types of electric charge: positive and negative.
Atoms, in their neutral state, contain an equal number of positively charged particles (protons) and negatively charged particles (electrons).
It is this delicate balance that dictates the overall stability and behavior of the atom.
Protons and Electrons: The Charge Carriers
Within an atom, protons and electrons are the primary carriers of electric charge. Protons, located in the nucleus, carry a positive charge (+1). Electrons, orbiting the nucleus, carry a negative charge (-1).
Neutrons, also residing in the nucleus, are electrically neutral, possessing no charge.
The number of protons defines the element. Changing the number of protons transforms the atom into a completely different element.
The interplay between protons and electrons is the driving force behind all chemical interactions.
The Influence of Charge on Atomic Stability
Charge plays a critical role in maintaining atomic stability.
The attractive electrostatic force between the positively charged protons in the nucleus and the negatively charged electrons in orbit holds the atom together.
This force counteracts the repulsive forces between the protons themselves, preventing the nucleus from flying apart.
When the number of protons and electrons are equal, the atom is electrically neutral and generally more stable.
However, atoms can gain or lose electrons, disrupting this balance and creating ions – atoms with a net positive or negative charge. It is this very imbalance that forms the basis for the sodium charge.
Charge dictates how atoms interact, setting the stage for understanding chemical behavior. But to fully appreciate sodium’s willingness to become positively charged, we must examine its inherent architecture. Let’s delve into the specifics of sodium’s atomic structure and electron arrangement to reveal the key factors that govern its reactivity.
Diving into Sodium's Atomic Architecture
Every element possesses a unique atomic fingerprint. This fingerprint is defined by its atomic number, representing the count of protons within its nucleus. It also includes its atomic mass, which is the approximate total mass of protons and neutrons found in the nucleus.
Unveiling Sodium's Identity: Atomic Number and Mass
Sodium, symbolized as Na, holds the atomic number 11. This means that every sodium atom contains exactly 11 protons nestled within its nucleus. This is the defining characteristic of sodium; any atom with a different number of protons is, by definition, a different element.
Sodium's atomic mass is approximately 23 atomic mass units (amu). The difference between the atomic mass (23) and the atomic number (11) indicates that a typical sodium atom has 12 neutrons. Keep in mind that isotopes of sodium can exist with varying numbers of neutrons.
Electron Configuration: A Road Map of Sodium's Electrons
While the nucleus defines the element, the electrons dictate its chemical behavior. Electrons reside in specific energy levels, or shells, around the nucleus. The electron configuration provides a "road map" showing how these electrons are distributed.
For sodium, the electron configuration is 1s² 2s² 2p⁶ 3s¹. This notation reveals a specific arrangement.
- The first energy level (n=1) holds a maximum of two electrons, as indicated by 1s².
- The second energy level (n=2) houses eight electrons, divided into two sublevels: 2s² and 2p⁶.
- Finally, the third energy level (n=3) contains a single electron in the 3s orbital.
The Lone Voyager: Sodium's Valence Electron
The outermost electron shell is known as the valence shell. The electrons residing in this shell are called valence electrons. These are the key players in chemical bonding.
Sodium possesses only one valence electron, located in its 3s orbital. This single electron is relatively far from the nucleus. Because of this, it is more easily influenced by external forces.
The presence of this single valence electron is the pivotal reason behind sodium's eagerness to lose an electron and attain a positive charge. This, in turn, influences its chemical reactivity.
Charge dictates how atoms interact, setting the stage for understanding chemical behavior. But to fully appreciate sodium’s willingness to become positively charged, we must examine its inherent architecture. Let’s delve into the specifics of sodium’s atomic structure and electron arrangement to reveal the key factors that govern its reactivity.
From Sodium Atom to Sodium Ion (Na+): The Act of Ionization
The ionization of sodium is a pivotal event that explains its chemical behavior and the formation of its characteristic positive charge. This process involves the departure of sodium's single valence electron, transforming a neutral sodium atom (Na) into a positively charged sodium ion (Na+).
The Electron's Exit: A Step-by-Step Look
Ionization is not a spontaneous act; it requires energy input to overcome the attractive forces between the nucleus and the electron.
Imagine sodium's valence electron as being held onto the atom's outermost orbit. Sufficient energy, often in the form of heat or interaction with another atom or molecule, must be supplied to eject this electron from the atom's grasp.
Once the valence electron gains enough energy, it overcomes the nuclear pull and leaves the atom entirely.
The Birth of Na+: Positive Charge Takes Center Stage
With the loss of a negatively charged electron, the balance between protons and electrons is disrupted within the sodium atom. Sodium, which initially possessed 11 protons and 11 electrons (rendering it neutral), now has 11 protons but only 10 electrons.
This imbalance results in a net positive charge of +1. The sodium atom has now transformed into a sodium ion, denoted as Na+.
The resulting positive charge is not merely a formality; it fundamentally alters sodium's properties and its interactions with other atoms.
Electron Configuration: Before and After
The change in the electron configuration is equally significant. Before ionization, sodium’s electron configuration is 1s² 2s² 2p⁶ 3s¹.
The 3s¹ signifies that single, reactive valence electron in the outermost shell.
After losing this electron, the sodium ion's electron configuration becomes 1s² 2s² 2p⁶.
This new configuration mirrors that of neon (Ne), a noble gas with a completely filled outer electron shell. This newly achieved state of stability is crucial for understanding why sodium readily forms a positive charge. The loss of the electron from the 3s orbital is the event which makes the process energetically favourable.
With the understanding that sodium readily gives up its valence electron, we can now look at what governs this tendency. The key to understanding sodium's reactivity lies in its address within the grand map of elements: the periodic table.
Sodium's Place in the Periodic Table: A Key to Understanding Reactivity
Sodium's location in the periodic table isn't arbitrary. It's a direct reflection of its electronic structure and, consequently, its chemical behavior. Specifically, sodium resides in Group 1, also known as the alkali metals.
This placement reveals fundamental truths about the element.
Group 1: The Alkali Metal Family
Alkali metals share a common trait: a single valence electron in their outermost shell. This seemingly small detail has profound implications for their reactivity.
Think of Group 1 as a family sharing similar characteristics.
Each member, including sodium, has a strong desire to lose that lone valence electron to achieve a more stable electron configuration.
The Valence Electron Connection
The periodic table is structured so that elements within the same group exhibit similar chemical properties. The group number directly corresponds to the number of valence electrons in the outermost shell of the atom.
Sodium, in Group 1, possesses one valence electron.
Potassium (K), also in Group 1, also possesses one valence electron.
This shared characteristic is not a coincidence.
Ionization Energy: A Measure of Reactivity
Ionization energy is the energy required to remove an electron from a gaseous atom. It's a quantifiable measure of how easily an atom loses an electron and, therefore, a direct indicator of its reactivity.
Within Group 1, ionization energy decreases as you move down the group.
This means that sodium (Na) has a higher ionization energy than potassium (K), but a lower ionization energy than lithium (Li).
The Downward Trend: Why It Matters
The decreasing ionization energy down Group 1 is due to two primary factors:
- Increased Atomic Size: As you move down the group, the atomic radius increases. The valence electron is further from the positively charged nucleus, experiencing a weaker attractive force.
- Increased Shielding: Inner electrons shield the valence electron from the full positive charge of the nucleus, further reducing the attractive force.
Therefore, the valence electron is easier to remove as you descend Group 1.
In summary, sodium's location in Group 1 of the periodic table tells us it's an alkali metal with one valence electron. This placement makes it eager to lose that electron, a tendency reflected in its relatively low ionization energy compared to elements further up or to the right in the periodic table.
Sodium's eagerness to relinquish its valence electron might seem odd at first glance. After all, wouldn't it be more stable to simply hold onto what it already possesses? The answer lies in a fundamental principle of chemistry: the drive for stability.
The Quest for Stability: Why Sodium Prefers a Positive Charge
The driving force behind sodium's proclivity for acquiring a positive charge stems from its pursuit of a stable electron configuration. This pursuit is governed by a concept known as the octet rule and ultimately leads to a state of lower energy, making the formation of Na+ energetically favorable.
The Octet Rule: A Foundation of Chemical Stability
The octet rule is a guiding principle in chemistry that dictates atoms are most stable when surrounded by eight valence electrons. This "magic number" of eight corresponds to a filled s and p subshell in the outermost energy level. Think of it as an atom's aspiration to mimic the noble gases, which are renowned for their inertness precisely because they already possess a full octet.
Sodium, with its electron configuration of 1s² 2s² 2p⁶ 3s¹, is just one electron away from achieving the noble gas configuration of neon (1s² 2s² 2p⁶). Rather than gaining seven electrons to complete its octet, which would require a tremendous amount of energy, sodium finds it far easier to simply lose its single 3s¹ electron.
Ionic Bonding: Sodium's Generous Donation
The act of donating its valence electron allows sodium to attain a full outer shell, satisfying the octet rule. This electron doesn't simply vanish; instead, it is typically transferred to another atom, most commonly one that is in need of electrons to complete its octet. This transfer is the foundation of ionic bonding.
For instance, consider the reaction between sodium and chlorine. Chlorine, with seven valence electrons, needs only one more to achieve a stable octet. Sodium readily donates its electron to chlorine, forming the positively charged sodium ion (Na+) and the negatively charged chloride ion (Cl-).
These oppositely charged ions are then attracted to each other, forming the ionic compound sodium chloride (NaCl), common table salt.
Energetic Favorability: A Downhill Path
The formation of Na+ is not merely a matter of achieving a stable electron configuration; it is also energetically favorable. This means that the process releases energy, resulting in a lower, more stable energy state for the sodium ion compared to the neutral sodium atom.
Quantifying Energetic Favorability
The energetic favorability can be understood through the lens of ionization energy and electron affinity. Ionization energy, as previously mentioned, is the energy required to remove an electron from an atom. While it costs energy to remove sodium's valence electron, this energy input is offset by the energy released when another atom, such as chlorine, accepts that electron (electron affinity) and by the subsequent formation of the ionic bond.
The overall energy change for the process is negative, indicating that the formation of Na+ is thermodynamically favorable. This is why sodium readily forms ionic bonds, seeking the stability and lower energy state that comes with a full octet and a positive charge.
In essence, sodium's "preference" for a positive charge is not a matter of choice but rather a consequence of fundamental physical laws that govern the behavior of atoms and their quest for stability.
That electron, now happily residing with another atom, leaves sodium with a net positive charge. This positively charged ion opens the door to a whole new world of chemical interactions.
Cations: Sodium as a Prime Example
In the realm of ionic chemistry, the loss of electrons is just as important as the gain. Atoms that surrender one or more electrons, like sodium, transform into positively charged ions known as cations.
Sodium (Na), upon losing its single valence electron, becomes the sodium ion (Na+), a quintessential and highly significant cation.
What Defines a Cation?
A cation is simply an ion with a positive electrical charge. This charge arises because the atom now has more protons (positive charges) than electrons (negative charges).
The magnitude of the positive charge indicates the number of electrons lost. In the case of Na+, the +1 charge signifies the loss of one electron.
The Link Between Cations and Ionic Bonding
Cations play a pivotal role in ionic bonding, the electrostatic attraction between oppositely charged ions.
The positive charge of a cation is irresistibly drawn to the negative charge of an anion (a negatively charged ion).
This attraction forms a strong chemical bond, creating ionic compounds. Sodium's willingness to become Na+ makes it a key player in forming these compounds.
Common Sodium Compounds: Examples
Sodium's cationic form (Na+) is found in countless compounds essential to our daily lives and industrial processes. Here are a few noteworthy examples:
-
Sodium Chloride (NaCl): More commonly known as table salt, sodium chloride is ubiquitous in cooking and food preservation. It's also a crucial electrolyte in biological systems.
-
Sodium Bicarbonate (NaHCO₃): Baking soda, or sodium bicarbonate, is a versatile compound used in baking, cleaning, and even as an antacid.
-
Sodium Hydroxide (NaOH): Also known as lye or caustic soda, sodium hydroxide is a highly alkaline substance used in manufacturing soap, paper, and various chemical processes.
-
Sodium Carbonate (Na₂CO₃): Commonly known as washing soda, it's used as a water softener and in the manufacturing of glass and detergents.
These examples merely scratch the surface of the vast array of sodium compounds that exist. The tendency of sodium to readily form cations makes it a vital building block in the chemical world, impacting everything from the food we eat to the medicines we take.
That electron, now happily residing with another atom, leaves sodium with a net positive charge. This positively charged ion opens the door to a whole new world of chemical interactions.
Sodium in Action: Ionic Compounds and Their Properties
Sodium's journey from a neutral atom to a positively charged ion (Na+) is just the beginning of its chemical story. The real magic happens when this ion interacts with negatively charged ions, forming ionic compounds with distinct and fascinating properties. Let's explore this world of sodium-containing ionic compounds.
The Essence of Ionic Bonding with Sodium
Ionic bonding is the electrostatic attraction between oppositely charged ions. In the case of sodium, the Na+ ion is strongly attracted to anions (negatively charged ions), such as chloride (Cl-), oxide (O2-), or bicarbonate (HCO3-).
This attraction arises from the fundamental principle that opposites attract. The positive charge of sodium seeks to neutralize itself by associating with a negative charge, resulting in a stable chemical bond.
Properties of Ionic Compounds with Sodium
Ionic compounds, formed through the electrostatic interactions of sodium ions and other anions, display distinctive characteristics.
High Melting and Boiling Points
A hallmark of ionic compounds is their relatively high melting and boiling points. This stems from the strength of the ionic bonds holding the ions together in a crystal lattice structure.
Significant energy is required to overcome these powerful electrostatic forces, leading to higher temperatures needed to induce phase changes from solid to liquid and from liquid to gas.
Solubility in Polar Solvents
Many ionic compounds, including those containing sodium, exhibit good solubility in polar solvents like water (H2O).
Water molecules, being polar themselves, can effectively interact with and solvate the individual ions, breaking apart the crystal lattice and dispersing the ions throughout the solution.
Electrical Conductivity
In their solid state, ionic compounds do not conduct electricity very well. The ions are locked in a rigid lattice, preventing them from freely moving and carrying an electrical charge.
However, when dissolved in water or melted, ionic compounds become excellent conductors of electricity. The ions are now free to move and transport charge, facilitating electrical current flow.
Real-World Examples of Sodium Compounds
Sodium, in its ionic form, is a versatile player in a multitude of compounds that touch our lives every day.
Sodium Chloride (NaCl): Table Salt
Perhaps the most well-known sodium compound is sodium chloride (NaCl), commonly known as table salt. It's a crucial ingredient in cooking, food preservation, and various industrial processes.
Sodium Bicarbonate (NaHCO3): Baking Soda
Sodium bicarbonate (NaHCO3), also known as baking soda, is a versatile compound with applications ranging from baking to cleaning to acting as an antacid.
Sodium Hydroxide (NaOH): Lye
Sodium hydroxide (NaOH), also known as lye or caustic soda, is a highly alkaline compound used in soap making, drain cleaning, and the manufacturing of various chemicals.
Sodium Carbonate (Na2CO3): Washing Soda
Sodium carbonate (Na2CO3), also known as washing soda, is employed as a water softener, cleaning agent, and a component in the manufacturing of glass and detergents.
These examples illustrate the wide-ranging applications of sodium compounds, highlighting the importance of understanding the principles of ionic bonding and the unique properties of these substances. From the food we eat to the products we use every day, sodium plays a vital role in our modern world.
That electron, now happily residing with another atom, leaves sodium with a net positive charge. This positively charged ion opens the door to a whole new world of chemical interactions.
Sodium: A Crucial Electrolyte in Biological Systems
Beyond the test tube and the industrial applications, sodium ions (Na+) play a starring role in the intricate chemistry of life. They are not merely bystanders; they are essential electrolytes, vital for maintaining the delicate balance within our bodies and enabling critical physiological functions.
The Electrolyte Imperative
Electrolytes are minerals in your body that have an electric charge. They are found in your blood, urine, and sweat. Electrolytes are critical because they help:
- Balance the amount of water in your body.
- Balance your body's acid/base (pH) level.
- Move nutrients into your cells.
- Move waste out of your cells.
Sodium is one of the most important electrolytes in the human body.
Sodium's Role in Fluid Balance and Blood Pressure
Sodium ions are instrumental in regulating fluid balance. Through osmosis, water moves to areas with higher solute concentrations. By controlling sodium concentration in various compartments, the body dictates where water flows.
This is crucial for maintaining cell volume, blood volume, and overall hydration. Kidneys play a critical role in regulating the concentration of sodium in body fluids.
Furthermore, sodium is a key player in blood pressure regulation. Sodium affects blood volume, and blood volume affects blood pressure.
The relationship between sodium intake and blood pressure is complex and influenced by factors such as genetics, kidney function, and overall diet. However, maintaining a proper sodium balance is crucial for supporting healthy blood pressure levels.
The Sodium-Potassium Pump and Nerve Function
One of the most fascinating examples of sodium's biological role is its involvement in the sodium-potassium pump, a protein found in the cell membrane of all animal cells. This pump uses energy (in the form of ATP) to actively transport sodium ions out of the cell and potassium ions into the cell.
This creates an electrochemical gradient across the cell membrane, essential for nerve impulse transmission, muscle contraction, and nutrient transport. Without this gradient, our nerves wouldn't fire, our muscles wouldn't contract, and our cells wouldn't function properly.
Nerve cells use the electrochemical gradient generated by the sodium-potassium pump to transmit electrical signals. These signals allow us to think, feel, and move. The rapid influx of sodium ions into a nerve cell is what generates the action potential, the fundamental unit of nerve communication.
The sodium-potassium pump is not just a static structure; it's a dynamic molecular machine constantly working to maintain the cellular environment necessary for life.
Sodium is more than just table salt; it's a fundamental element in the intricate machinery that keeps us alive and functioning.
Video: Sodium Charge Demystified: What You NEED to Know!
Sodium Charge Demystified: Frequently Asked Questions
Here are some common questions about sodium and its charge to help clarify the key concepts from the main article.
What exactly is the charge of a sodium ion?
A sodium ion (represented as Na+) has a positive charge of +1. This means it has lost one electron. This loss is what creates the sodium charge and makes it an ion.
Why does sodium lose an electron and become positively charged?
Sodium readily loses its outermost electron to achieve a more stable electron configuration. By losing one electron, it resembles the noble gas neon. This loss results in a positive sodium charge because there is now one more proton than electrons.
How does the sodium charge relate to its reactivity?
The positive sodium charge makes sodium highly reactive. It is driven to form ionic bonds with negatively charged ions, like chloride (Cl-), to become stable. That's why sodium chloride (table salt) exists.
Where do we typically find sodium in its charged form?
Sodium is rarely found in its pure, uncharged metallic form in nature. Due to its reactivity and resulting sodium charge, it almost always exists as a positively charged ion within compounds, such as salts dissolved in water or within minerals in rocks.
Alright, that's a wrap on sodium charge! Hopefully, you've got a better grasp of things now. Go forth and use that knowledge – maybe even impress your friends with some fun facts! See ya around!