Recessive Trait: Inheritance, Risks Explained
Inheritance patterns, studied extensively using tools like the Punnett Square, often determine the expression of genetic characteristics, where a recessive trait manifests only when an individual inherits two copies of the responsible allele. Genetic counseling, provided by organizations such as the National Society of Genetic Counselors, plays a crucial role in assessing the risks associated with inheriting certain recessive conditions, particularly when both parents are carriers. Understanding the principles established by Gregor Mendel helps to explain how these traits, though not immediately apparent, can appear in subsequent generations. The expression of a recessive trait requires the absence of a dominant allele, showcasing the fundamental mechanisms of genetic inheritance and potential health implications.
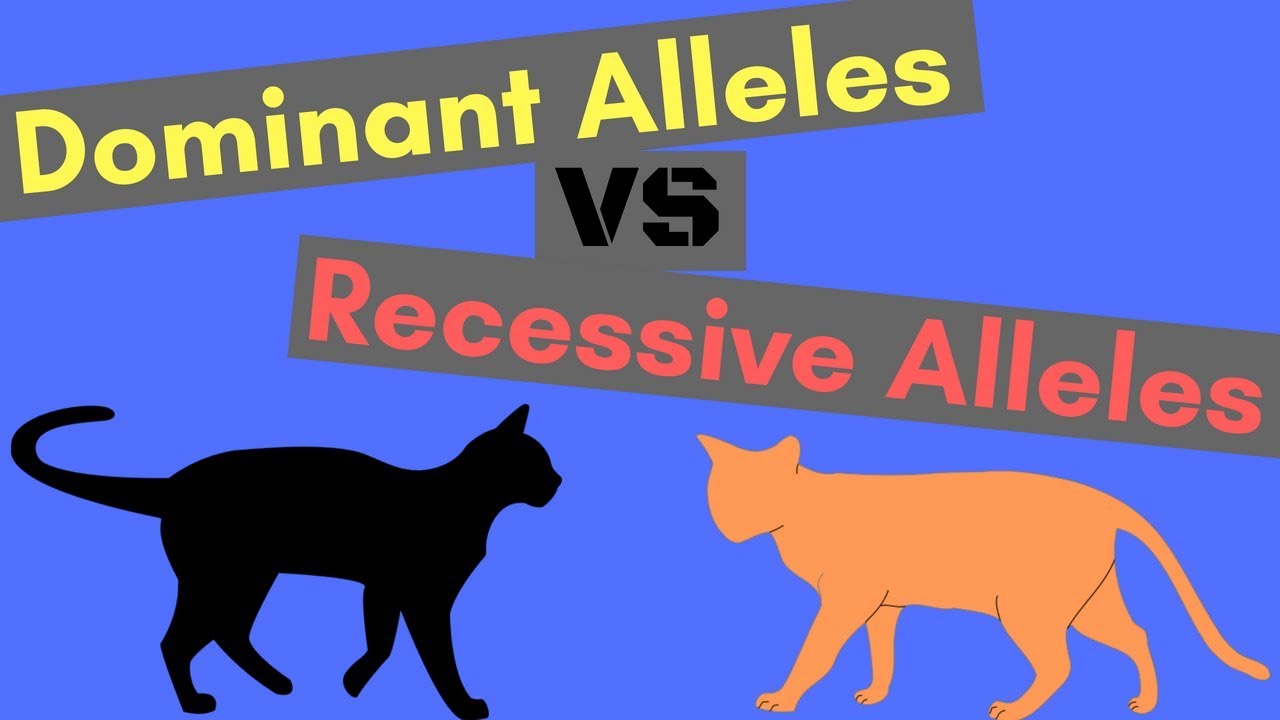
Image taken from the YouTube channel 2 Minute Classroom , from the video titled Dominant Alleles vs Recessive Alleles | Understanding Inheritance .
Unlocking the Secrets of Genetics and Inheritance
Genetics, the scientific study of heredity and variation, stands as a cornerstone in our comprehension of life itself. It delves into the intricate mechanisms by which traits are passed down through generations, shaping the diversity and complexity of living organisms. From the smallest microbe to the largest mammal, genetics provides the framework for understanding the very essence of life's continuity.
The Essence of Inheritance
At the heart of genetics lies the concept of inheritance—the process by which characteristics are transmitted from parents to their offspring. This inheritance occurs through the transmission of genes, the fundamental units of heredity.
These genes, composed of DNA, carry the coded instructions that determine an organism's traits, influencing everything from physical appearance to predisposition to certain diseases. Understanding inheritance is crucial for deciphering how traits are passed on and how variations arise within populations.
Genetics and Human Well-being
The relevance of genetics extends far beyond the realm of pure science, profoundly impacting human health, disease, and evolution. Genetic factors play a significant role in the development of many diseases, including cancer, heart disease, and neurological disorders. Identifying these genetic predispositions allows for early detection, personalized treatments, and preventive measures.
Furthermore, genetics sheds light on the evolutionary history of humans, tracing our ancestry and illuminating the processes that have shaped our species over millennia.
A Comprehensive Overview
This article serves as an introduction to the fundamental concepts of genetics and inheritance. It explores the foundational principles that underpin heredity, elucidating the patterns of inheritance observed in nature.
Moreover, it examines the role of mutations in causing genetic disorders and discusses the methods used to analyze genetic information and provide counseling. The role of probability in predicting genetic outcomes is also addressed.
Finally, we acknowledge key contributors to the field and provide information about support resources available to individuals and families affected by genetic disorders.
Foundational Concepts: Building Blocks of Heredity
To fully grasp the complexities of genetics and inheritance, it's imperative to establish a strong foundation in the core concepts that govern the field. These fundamental principles provide the necessary framework for understanding how traits are passed down, expressed, and contribute to the diversity of life.
Decoding Genetics: The Science of Heredity
Genetics is the scientific study of heredity and variation in living organisms. It encompasses the intricate mechanisms by which traits are passed from one generation to the next, and how these traits can vary among individuals.
This vast field explores the roles of genes, genetic variation, and heredity, seeking to unravel the complexities of how life is encoded and transmitted. Genetics provides insight into understanding the transmission of traits and the underlying causes of genetic variation.
Unveiling Inheritance: The Transmission of Traits
Inheritance is the process by which genetic information is passed from parents to their offspring. This crucial mechanism involves the transmission of genes and alleles, the building blocks that determine an organism's characteristics.
Through inheritance, offspring inherit a combination of genetic material from their parents, contributing to their unique genetic makeup. Understanding inheritance patterns allows us to predict the likelihood of certain traits being passed down.
Genes and Alleles: The Blueprint of Life
Genes are the fundamental units of heredity, composed of DNA sequences that encode specific traits. They serve as the blueprints for an organism's development, function, and characteristics.
Genes are the functional units of heredity.
Alleles, on the other hand, are alternative forms of a gene. For example, a gene for eye color might have alleles for blue eyes, brown eyes, or green eyes.
The interaction of alleles determines how a trait is expressed.
Dominant Traits: Expressing Genetic Potential
A dominant trait is one that is expressed in an individual even when only one copy of the dominant allele is present. In other words, the presence of a single dominant allele is sufficient for the trait to manifest.
Dominant traits mask the expression of recessive alleles.
Genotype vs. Phenotype: Unveiling the Genetic Code
The genotype refers to the genetic constitution of an individual, encompassing the specific alleles they possess for a particular trait. It represents the underlying genetic makeup that influences observable characteristics.
The phenotype, however, refers to the observable characteristics of an individual, resulting from the interaction of their genotype with environmental factors. Phenotype is the physical expression.
Understanding the distinction between genotype and phenotype is crucial for deciphering the relationship between genes and traits.
Homozygous and Heterozygous: Exploring Allele Combinations
An individual is homozygous for a particular gene if they possess two identical alleles for that gene. This means that both alleles are the same, resulting in a consistent expression of the trait.
Conversely, an individual is heterozygous if they possess two different alleles for a particular gene. In this case, the interaction between the different alleles determines the resulting phenotype.
Punnett Square: Predicting Genetic Outcomes
The Punnett square is a powerful tool used in genetics to predict the potential genotypes and phenotypes of offspring based on the genotypes of their parents. This diagrammatic representation allows us to visualize the possible combinations of alleles that offspring can inherit.
By constructing a Punnett square, we can calculate the probabilities of different genetic outcomes, providing valuable insights into inheritance patterns.
Patterns of Inheritance: How Traits Are Passed Down
Understanding the patterns of inheritance is crucial to deciphering how genetic traits are transmitted from parents to offspring. These patterns dictate the likelihood of inheriting specific characteristics, including those associated with genetic disorders.
Let's delve into some of the most common inheritance patterns, specifically focusing on autosomal recessive and X-linked recessive inheritance, unraveling the intricacies of their transmission and impact.
Autosomal Recessive Inheritance
Autosomal recessive inheritance describes a scenario where an individual must inherit two copies of a mutated gene, located on a non-sex chromosome (autosome), to express the associated trait or disorder.
This pattern has distinct characteristics that can be traced through family pedigrees. Often, autosomal recessive conditions appear to "skip" generations.
This occurs because carriers, who possess only one copy of the mutated gene, typically do not exhibit the trait themselves but can pass the mutated allele to their children.
Characteristics of Autosomal Recessive Inheritance
Key characteristics include:
-
Skipping Generations: Affected individuals may not have affected parents, as both parents can be carriers.
-
Equal Affectation of Sexes: Males and females are equally likely to be affected, as the mutated gene is located on an autosome, not a sex chromosome.
-
Carrier Status: Individuals with one copy of the mutated gene are carriers and usually asymptomatic.
The Role of Carriers
Carriers play a pivotal role in autosomal recessive inheritance. A carrier is an individual who possesses one normal allele and one mutated allele for a particular gene. While carriers typically do not exhibit the phenotype associated with the mutated gene, they can transmit the mutated allele to their offspring.
If two carriers have children, each child has a 25% chance of inheriting both mutated alleles and expressing the recessive trait, a 50% chance of becoming a carrier themselves, and a 25% chance of inheriting two normal alleles and not being affected or a carrier.
X-linked Recessive Inheritance
X-linked recessive inheritance is characterized by a mutated gene located on the X chromosome. Due to the differences in sex chromosome composition between males (XY) and females (XX), the expression of X-linked recessive traits differs significantly between the sexes.
Males, possessing only one X chromosome, will express the trait if they inherit the mutated allele. Females, on the other hand, need to inherit two copies of the mutated allele (one on each X chromosome) to express the trait, making them less likely to be affected.
Prevalence in Males
A defining characteristic of X-linked recessive inheritance is the higher prevalence of affected males compared to females.
This disparity arises because males only need to inherit one copy of the mutated gene on their single X chromosome to express the trait, whereas females need two copies.
Carrier Females
Females who inherit one copy of the mutated allele on one X chromosome are typically carriers. They usually do not exhibit the full-blown phenotype associated with the trait, but they can pass the mutated allele to their children.
The sons of carrier females have a 50% chance of inheriting the mutated X chromosome and expressing the X-linked recessive trait. Their daughters have a 50% chance of becoming carriers themselves.
Understanding the nuances of X-linked recessive inheritance is critical for predicting disease risk and providing informed genetic counseling.
Genetic Disorders and Mutations: When Things Go Wrong
Understanding the patterns of inheritance is crucial to deciphering how genetic traits are transmitted from parents to offspring. These patterns dictate the likelihood of inheriting specific characteristics, including those associated with genetic disorders.
Let's delve into some of the most common ways genetics can go awry, leading to a range of health conditions and challenges.
The Central Role of Mutations
At the heart of many genetic disorders lies the mutation, a fundamental alteration in the DNA sequence of a gene. These changes can range from a single nucleotide substitution to larger-scale rearrangements of entire chromosomal regions.
Mutations can arise spontaneously during DNA replication or be induced by external factors such as radiation or exposure to certain chemicals. The consequences of a mutation vary widely, depending on its location within the gene and the nature of the change.
Some mutations may have no discernible effect, while others can disrupt gene function, leading to the production of a non-functional or dysfunctional protein. It is this disruption that often underlies the development of a genetic disorder.
Common Genetic Disorders: A Closer Look
Several genetic disorders are relatively common, each arising from a specific genetic mutation and affecting particular organ systems. Understanding these disorders provides valuable insight into the complexities of genetics and their impact on human health.
Cystic Fibrosis (CF)
Cystic Fibrosis (CF) is an autosomal recessive disorder primarily affecting the respiratory and digestive systems. It stems from mutations in the CFTR gene, which encodes a protein responsible for regulating the flow of salt and water across cell membranes.
The most common mutation, deltaF508, leads to the production of a misfolded CFTR protein that is degraded before reaching the cell surface. This results in the buildup of thick mucus in the lungs and other organs, leading to chronic infections, breathing difficulties, and digestive problems.
Sickle Cell Anemia
Sickle Cell Anemia is another autosomal recessive disorder, this time affecting red blood cells. It arises from a mutation in the HBB gene, which encodes a subunit of hemoglobin, the protein responsible for carrying oxygen in red blood cells.
The most common mutation, HbS, causes the hemoglobin molecules to clump together under low-oxygen conditions, deforming the red blood cells into a characteristic "sickle" shape.
These sickled cells are rigid and fragile, leading to chronic anemia, pain crises, and damage to various organs due to impaired blood flow.
Phenylketonuria (PKU)
Phenylketonuria (PKU) is an autosomal recessive metabolic disorder resulting from a deficiency in the enzyme phenylalanine hydroxylase (PAH). This enzyme is responsible for converting the amino acid phenylalanine into tyrosine.
Mutations in the PAH gene lead to the accumulation of phenylalanine in the blood and brain, which can cause intellectual disability and other neurological problems if left untreated.
Newborn screening for PKU is routinely performed in many countries, allowing for early dietary interventions to prevent these severe complications.
Tay-Sachs Disease
Tay-Sachs Disease is a rare autosomal recessive disorder that progressively destroys nerve cells (neurons) in the brain and spinal cord. It is caused by mutations in the HEXA gene, which encodes an enzyme called beta-hexosaminidase A.
This enzyme is responsible for breaking down a fatty substance called GM2 ganglioside in lysosomes, the cell's recycling centers. Mutations in HEXA prevent the breakdown of GM2 ganglioside, leading to its accumulation in neurons, ultimately causing cell death.
Tay-Sachs disease is particularly prevalent in certain populations, such as Ashkenazi Jews, and is typically fatal in early childhood.
Albinism
Albinism refers to a group of inherited disorders characterized by a lack of pigment (melanin) in the skin, hair, and eyes. It results from mutations in genes involved in the production or distribution of melanin.
The most common type, oculocutaneous albinism (OCA), is caused by mutations in genes such as TYR, OCA2, and TYRP1.
The severity of albinism varies depending on the specific mutation, ranging from a complete absence of pigment to a mild reduction. Individuals with albinism are at increased risk of sun damage and skin cancer and may experience vision problems.
Spinal Muscular Atrophy (SMA)
Spinal Muscular Atrophy (SMA) is a group of genetic disorders that affect the motor neurons in the spinal cord, leading to muscle weakness and atrophy. It is most commonly caused by mutations in the SMN1 gene, which encodes a protein called survival motor neuron (SMN).
This protein is essential for the survival and function of motor neurons. SMA is characterized by progressive muscle weakness and can range in severity from mild weakness to profound paralysis and respiratory failure.
Galactosemia
Galactosemia is a rare autosomal recessive metabolic disorder that affects the body's ability to process galactose, a sugar found in milk and other dairy products.
It is caused by mutations in genes involved in the galactose metabolism pathway, most commonly the GALT gene, which encodes the enzyme galactose-1-phosphate uridyltransferase.
A deficiency in this enzyme leads to the accumulation of galactose in the blood and tissues, causing liver damage, kidney failure, and developmental delays. Early diagnosis and dietary restriction of galactose are crucial to prevent these severe complications.
Genetic Analysis and Counseling: Understanding Your Risk
Understanding the patterns of inheritance is crucial to deciphering how genetic traits are transmitted from parents to offspring. These patterns dictate the likelihood of inheriting specific characteristics, including those associated with genetic disorders.
Let's delve into some of the most common techniques in genetic analysis and how genetic counseling empowers individuals and families to navigate the complexities of inherited risks.
Pedigree Analysis: Tracing Family Traits
Pedigree analysis is a powerful tool used to trace the inheritance of specific traits through generations of a family. It involves creating a visual representation of a family's history, highlighting affected individuals and their relationships.
Symbols are used to denote males, females, affected individuals, carriers, and deceased family members. This graphical representation allows geneticists to identify patterns of inheritance, such as autosomal dominant, autosomal recessive, or X-linked inheritance.
By carefully examining the pedigree, it's often possible to determine whether a trait is dominant or recessive. It can also reveal whether it is linked to a specific chromosome. This information is crucial for assessing the risk of future generations inheriting a particular condition.
The use of pedigree charts serves as a valuable first step. It helps in understanding potential genetic risks within a family.
The Impact of Consanguinity
Consanguinity refers to relationships between individuals who share a common ancestor. Consanguineous relationships, such as marriages between cousins, increase the risk of offspring inheriting autosomal recessive traits.
This heightened risk arises because related individuals are more likely to carry the same rare, recessive alleles.
When both parents carry the same recessive allele for a particular gene, there is a higher probability that their child will inherit two copies of that allele. This results in the expression of the associated recessive trait or genetic disorder.
Therefore, consanguinity can significantly increase the prevalence of certain genetic conditions within specific populations.
Genetic Screening and Testing: Identifying Risks
Genetic screening and testing encompass a range of techniques. These are employed to identify individuals who are carriers of specific genetic mutations. They also identify those who are affected by genetic disorders.
Genetic screening often targets large populations to identify individuals who may be at increased risk. Newborn screening programs, for instance, test infants for a panel of genetic disorders shortly after birth. This allows for early intervention and management.
Genetic testing, on the other hand, is typically performed on individuals or families with a known risk. This is risk based on family history or specific symptoms.
-
Carrier screening can determine if an individual carries a single copy of a recessive gene mutation. This means they are at risk of passing it onto their children.
-
Diagnostic testing confirms or rules out a specific genetic condition in an individual with symptoms.
-
Predictive testing assesses the risk of developing a genetic disorder in the future. This is done before symptoms appear.
These tests provide valuable information. This allows individuals and families to make informed decisions about family planning and healthcare.
Genetic Counseling: Navigating Complex Decisions
Genetic counseling is a specialized field that provides individuals and families with information, support, and guidance regarding genetic risks and conditions. Genetic counselors are trained healthcare professionals with expertise in medical genetics, counseling, and communication.
They play a crucial role in helping individuals understand the implications of genetic testing results, assess their risk of developing or passing on a genetic disorder, and make informed decisions about their reproductive options and healthcare management.
Genetic counseling sessions often involve:
- A thorough review of family history.
- An explanation of inheritance patterns and genetic testing options.
- A discussion of the psychological and emotional aspects of genetic conditions.
- Support in coping with the emotional and practical challenges of living with a genetic disorder.
Genetic counselors act as advocates for their clients, ensuring that they have access to the information and resources they need to make informed choices that align with their values and goals. They empower individuals to navigate the complex landscape of genetics and make decisions that are right for them and their families.
Probability in Genetics: Predicting the Odds
Understanding the patterns of inheritance is crucial to deciphering how genetic traits are transmitted from parents to offspring. These patterns dictate the likelihood of inheriting specific characteristics, including those associated with genetic disorders.
Let's delve into some of the most essential applications of probability in genetics.
Probability, in the context of genetics, refers to the likelihood of a specific genetic outcome occurring. It allows us to predict the chances of offspring inheriting particular traits or genetic conditions.
The Foundation: Basic Probability Principles
At its core, probability is expressed as a value between 0 and 1. A probability of 0 indicates an impossible event, while a probability of 1 signifies a certain event.
For example, consider a coin toss: The probability of getting heads is 0.5 (or 50%), and the probability of getting tails is also 0.5 (or 50%), assuming a fair coin.
Applying Probability to Genetic Crosses
One of the most practical applications of probability in genetics is in predicting the outcomes of genetic crosses. This is often illustrated through the use of tools like the Punnett square.
Using Punnett Squares
The Punnett square is a visual representation that helps to determine the possible genotypes and phenotypes of offspring based on the genotypes of the parents. Each box within the square represents a possible combination of alleles from the parents.
Let's consider a simple monohybrid cross involving a single gene with two alleles: A (dominant) and a (recessive). If both parents are heterozygous (Aa), we can use a Punnett square to predict the genotypic and phenotypic ratios of their offspring.
The Punnett square would look like this:
A | a | |
---|---|---|
A | AA | Aa |
a | Aa | aa |
From this, we can see that there is a 25% chance (1/4) of the offspring being homozygous dominant (AA), a 50% chance (2/4) of being heterozygous (Aa), and a 25% chance (1/4) of being homozygous recessive (aa).
Calculating Phenotypic Ratios
The phenotypic ratio depends on the dominance relationship between the alleles. In this case, since A is dominant over a, individuals with either the AA or Aa genotype will express the dominant phenotype. Only those with the aa genotype will express the recessive phenotype.
Therefore, the phenotypic ratio is 3:1, meaning there is a 75% chance of the offspring exhibiting the dominant phenotype and a 25% chance of exhibiting the recessive phenotype.
Product and Sum Rules
In genetics, we often use the product rule and the sum rule to calculate probabilities for more complex scenarios.
The Product Rule
The product rule states that the probability of two or more independent events occurring together is the product of their individual probabilities. For example, if you want to know the probability of having two children, both with a specific trait, you would multiply the probability of each child having that trait.
The Sum Rule
The sum rule states that the probability of any one of several mutually exclusive events occurring is the sum of their individual probabilities.
This rule is used when there are multiple ways to achieve a particular outcome. For instance, if you want to know the probability of an offspring having a particular genotype that can result from multiple allele combinations, you would add the probabilities of each of those combinations.
Practical Implications and Limitations
Probability in genetics is not just a theoretical exercise. It has significant practical implications in genetic counseling, disease prediction, and breeding programs.
By understanding the probabilities associated with inheriting specific genes, genetic counselors can provide valuable information to families about the risks of passing on genetic disorders to their children.
However, it's crucial to remember that these are probabilities, not guarantees. Real-world outcomes can be influenced by a variety of factors, including environmental effects and complex gene interactions.
Therefore, while probability provides a powerful tool for predicting genetic outcomes, it should be used in conjunction with other forms of genetic analysis and a comprehensive understanding of the underlying biology.
Key Contributors to Genetics: Pioneers of Discovery
Understanding the patterns of inheritance is crucial to deciphering how genetic traits are transmitted from parents to offspring. These patterns dictate the likelihood of inheriting specific characteristics, including those associated with genetic disorders.
Let's delve into some of the most essential and groundbreaking figures in the history of genetics whose profound work has shaped our understanding of heredity and genetic mechanisms.
Defining the Geneticist: Architects of Heredity's Blueprint
A geneticist is a scientist who studies genes, heredity, and variation in living organisms. These researchers unravel the complexities of DNA, gene expression, and the transmission of traits from one generation to the next. The field of genetics owes its progress to the dedicated efforts of numerous brilliant minds.
Pivotal Figures in Genetics
Gregor Mendel: The Father of Modern Genetics
Gregor Mendel, an Austrian monk, is widely regarded as the father of modern genetics. Through his meticulous experiments with pea plants in the mid-19th century, Mendel formulated the fundamental principles of heredity.
His laws of segregation and independent assortment laid the groundwork for understanding how traits are passed from parents to offspring. Despite the initial lack of recognition, his work was rediscovered in the early 20th century, revolutionizing the field of biology.
Rosalind Franklin: Unveiling the Structure of DNA
Rosalind Franklin, a British chemist and X-ray crystallographer, made critical contributions to the discovery of the structure of DNA. Her X-ray diffraction images, most notably "Photo 51," provided crucial insights into the double helix structure of DNA.
Tragically, her contributions were not fully acknowledged during her lifetime. However, her work was instrumental in shaping our understanding of the molecular basis of heredity.
James Watson and Francis Crick: Decoding the Double Helix
James Watson and Francis Crick are credited with elucidating the double helix structure of DNA in 1953. Drawing upon Franklin’s X-ray diffraction data, they constructed a model that elegantly explained how genetic information is stored and replicated.
Their discovery revolutionized the field of molecular biology and earned them the Nobel Prize in Physiology or Medicine in 1962, along with Maurice Wilkins.
Other Notable Geneticists
Beyond these iconic figures, numerous other scientists have made invaluable contributions to genetics.
- Thomas Hunt Morgan
- Barbara McClintock
- Sewall Wright
- Erwin Chargaff
Their collective efforts have expanded our knowledge of genetics. Each has deepened our comprehension of the intricate mechanisms governing life.
Reflecting on the Legacy of Genetic Pioneers
The pioneers of genetics faced numerous challenges. They navigated skepticism and limited technology. Yet their intellectual curiosity and perseverance propelled the field forward. Their discoveries have laid the foundation for modern genetics, impacting medicine, agriculture, and biotechnology. Their work continues to inspire future generations of scientists. Each continues to push the boundaries of knowledge.
Organizations and Support: Finding Help and Resources
Understanding the patterns of inheritance is crucial to deciphering how genetic traits are transmitted from parents to offspring. These patterns dictate the likelihood of inheriting specific characteristics, including those associated with genetic disorders.
Let's delve into some of the most essential resources available to individuals and families navigating the complexities of genetic conditions. Support networks and advocacy groups provide crucial services, including information, guidance, and community.
The Role of Advocacy and Support Organizations
Disease-specific advocacy groups play a critical role in supporting individuals and families affected by genetic disorders. These organizations serve as hubs for information, connecting patients with healthcare professionals, researchers, and fellow patients.
They often fund research, promote awareness, and advocate for policies that improve the lives of those living with genetic conditions.
Defining Disease-Specific Advocacy Groups
Disease-specific advocacy groups are non-profit organizations dedicated to supporting individuals and families affected by a particular disease or group of related diseases. They focus on raising awareness, funding research, providing resources, and advocating for improved treatment and care.
Examples of Key Organizations
Several organizations stand out for their comprehensive support and advocacy efforts:
-
Cystic Fibrosis Foundation (CFF): The CFF is dedicated to finding a cure for cystic fibrosis and improving the quality of life for those living with the disease. They fund research, provide resources, and offer support to patients and families. Their work has significantly extended the lifespan and improved the well-being of individuals with CF.
-
Sickle Cell Disease Association of America (SCDAA): The SCDAA is committed to advocating for and supporting individuals and families affected by sickle cell disease. They provide education, resources, and support services, as well as advocating for policy changes that benefit the sickle cell community.
-
National Organization for Rare Disorders (NORD): NORD is a leading patient advocacy organization dedicated to individuals with rare diseases and the organizations that serve them. NORD provides advocacy, education, research, and patient services to improve the lives of all people affected by rare diseases.
-
Genetic Alliance: This organization is a network of consumer and professional organizations, universities, government agencies, and private companies. Genetic Alliance drives translational research, supports the development of new technologies, and advocates for policies to improve health.
-
The American Society of Human Genetics (ASHG): While primarily a professional organization for researchers and clinicians, ASHG also offers public resources on genetics and genomics, including educational materials and advocacy initiatives.
Support Beyond Disease-Specific Groups
While disease-specific organizations offer targeted assistance, other types of resources can provide broader support:
Government Agencies and Programs
Government agencies like the National Institutes of Health (NIH) and the Centers for Disease Control and Prevention (CDC) offer valuable information and resources on genetic disorders. These agencies conduct research, provide funding for research projects, and develop educational materials for healthcare professionals and the public.
Hospital and University Genetic Centers
Many hospitals and universities have specialized genetic centers that offer diagnostic testing, genetic counseling, and treatment for genetic disorders. These centers often participate in research studies, providing patients with access to cutting-edge treatments and clinical trials.
Online Communities and Forums
The internet has become an invaluable resource for connecting with others who share similar experiences. Online communities and forums dedicated to genetic disorders provide a space for patients and families to share information, offer support, and connect with experts.
Participating in these online communities can help individuals feel less alone and more empowered in managing their condition.
Navigating the Landscape of Support
Identifying the right resources and support networks can be overwhelming. Here are some steps to consider:
-
Consult with Healthcare Professionals: Your doctor, genetic counselor, or other healthcare providers can provide guidance on relevant organizations and resources.
-
Research Online: Utilize search engines and online directories to identify advocacy groups, support forums, and other resources specific to your condition.
-
Attend Support Group Meetings: In-person or virtual support group meetings offer an opportunity to connect with others who understand what you are going through.
-
Contact National Organizations: National organizations like NORD and Genetic Alliance can provide information and referrals to local resources.
By leveraging available resources and support networks, individuals and families affected by genetic disorders can navigate the challenges they face with greater knowledge, resilience, and hope.
Accessing support and information is a critical step in empowering individuals to manage their genetic health and improve their overall quality of life.
Video: Recessive Trait: Inheritance, Risks Explained
FAQs: Recessive Trait Inheritance
What does it mean for a trait to be recessive?
A recessive trait is a genetic characteristic that only appears when an individual inherits two copies of the responsible gene, one from each parent. If they inherit only one copy, the trait is masked by a dominant gene.
How can two parents without a certain trait have a child with that trait?
Both parents can be carriers of a recessive trait. They each have one normal, dominant gene and one recessive gene for the trait. If the child inherits the recessive gene from both parents, they will express the recessive trait.
What are the risks associated with inheriting a recessive trait?
The risks depend entirely on the specific recessive trait. Some are relatively harmless, like having blue eyes. Others can cause serious health conditions, such as cystic fibrosis or sickle cell anemia, if inherited.
If one parent has a recessive trait, what is the likelihood of their child also having it?
If only one parent has the recessive trait (meaning they have two copies of the recessive gene), and the other parent doesn’t carry it at all, none of their children will express the recessive trait, but all will be carriers. If the other parent is also a carrier, there's a 50% chance their child will be a carrier and a 50% chance they will have the trait.
So, that's the lowdown on recessive traits! While understanding inheritance can feel like a science class throwback, knowing about these traits can be super helpful, especially when planning for the future. Hopefully, this gives you a clearer picture of how recessive trait inheritance works and what it might mean for you and your family.