Rayleigh Scattering: Why is the Sky Blue?
The captivating hue of the sky blue results from an atmospheric phenomenon known as Rayleigh scattering, a process extensively studied at institutions like the National Oceanic and Atmospheric Administration (NOAA). This type of scattering, fundamentally different from Mie scattering which affects larger particles, involves the dispersion of electromagnetic radiation, such as sunlight, by particles of a much smaller wavelength. Specifically, the Rayleigh scattering sky blue effect is most pronounced with shorter wavelengths of light—blue and violet—as described by the Rayleigh scattering equation. Due to the sun's intensity, and human vision being more sensitive to blue, our perception is dominated by the azure tint that painters and photographers strive to capture accurately.
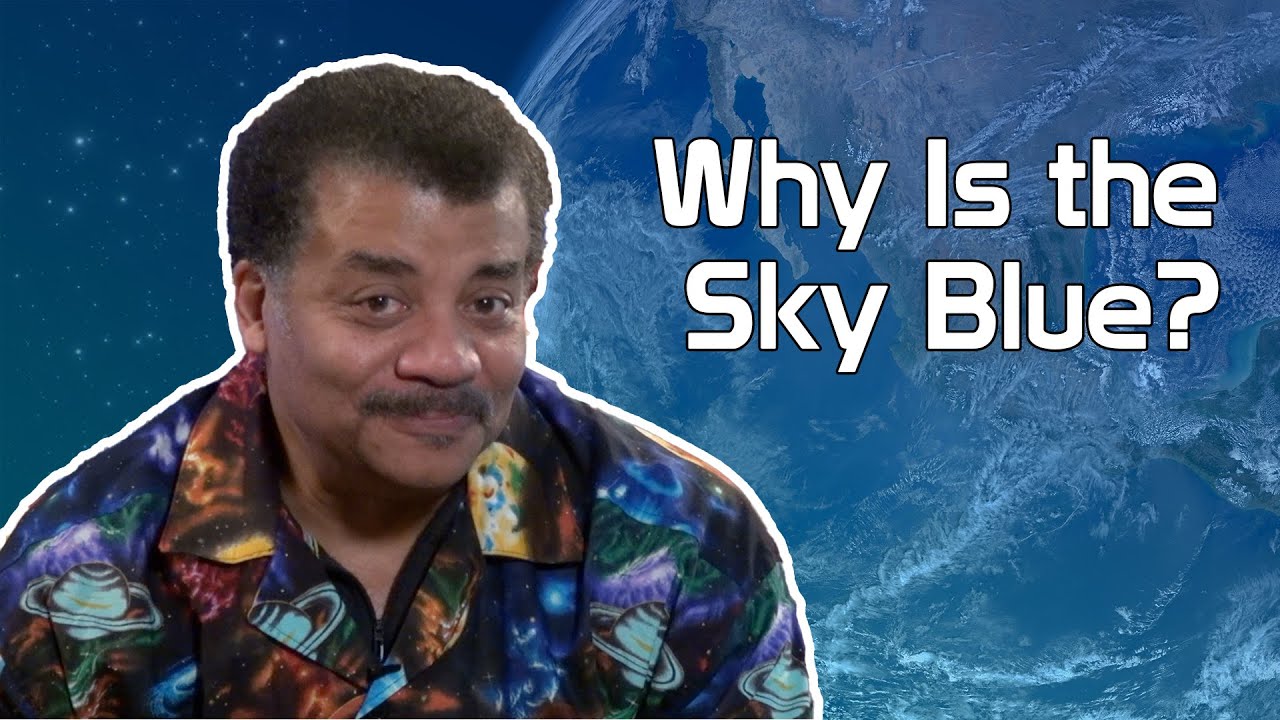
Image taken from the YouTube channel StarTalk , from the video titled Neil deGrasse Tyson Explains Why The Sky Is Blue .
Unveiling the Blue Sky: An Exploration of Rayleigh Scattering
The cerulean canvas above us, the azure dome we call the sky, is not merely an empty void. Its color, so pervasive and comforting, is a direct consequence of a fascinating phenomenon known as Rayleigh scattering.
This effect, the elastic scattering of electromagnetic radiation by particles of a much smaller wavelength, is a fundamental process that governs how light interacts with matter on a microscopic scale.
Defining Rayleigh Scattering
At its core, Rayleigh scattering describes what happens when light encounters particles significantly smaller than its own wavelength.
"Elastic scattering" indicates that the energy (and therefore wavelength) of the light remains unchanged. The light is simply redirected.
Imagine ripples in a pond encountering tiny pebbles; the pebbles don't absorb the water's energy, but they do disrupt the wave's path, causing it to spread out in different directions. This is analogous to how Rayleigh scattering works with light and air molecules.
The Sky's True Color: A Result of Atmospheric Scattering
The most prominent and beautiful manifestation of Rayleigh scattering is the blue color of the daytime sky. Sunlight, composed of all colors of the rainbow, enters the Earth's atmosphere. The shorter wavelengths of light – blue and violet – are scattered much more efficiently than the longer wavelengths, such as red and orange.
This preferential scattering of blue light is why we perceive the sky as overwhelmingly blue.
Without Rayleigh scattering, the sky would appear black, much like the view from the moon, where there is no atmosphere to scatter sunlight. The life we know is profoundly different without this phenomenon.
A Legacy of Discovery: Lord Rayleigh's Contribution
The understanding of this process is largely attributed to Lord Rayleigh (John William Strutt, 3rd Baron Rayleigh), a British physicist who made significant contributions to various fields, including acoustics and optics. His work in the late 19th century provided the theoretical framework for explaining why the sky is blue.
Rayleigh's meticulous experiments and mathematical formulations revealed the relationship between the intensity of scattered light and its wavelength, cementing his place as a pioneer in the field of atmospheric optics.
His dedication has been vital to our overall understanding of light and colour in the world we live in.
Laying the Foundation: Understanding Light and Scattering
Before delving deeper into the intricacies of Rayleigh scattering, it is crucial to establish a firm understanding of the fundamental concepts that underpin this phenomenon. These include the dual nature of light, the properties of wavelength and frequency, and the basic definition of scattering itself.
The Duality of Light: Wave and Particle
Light, or more accurately, electromagnetic radiation, exhibits a peculiar duality, behaving as both a wave and a particle. This concept, known as wave-particle duality, is a cornerstone of quantum mechanics.
As a wave, light is characterized by its oscillating electric and magnetic fields, propagating through space. This wave-like nature explains phenomena such as interference and diffraction.
Conversely, as a particle, light is composed of discrete packets of energy called photons. These photons carry specific amounts of energy, which determine the light's frequency and wavelength.
This duality is not merely an abstract theoretical concept; it is essential for understanding how light interacts with matter and, consequently, how Rayleigh scattering occurs.
Wavelength and Frequency: An Inverse Dance
Two critical properties of light are its wavelength and frequency, which are inversely related.
Wavelength (λ) refers to the distance between successive crests or troughs of a wave, typically measured in nanometers (nm).
Frequency (ν), on the other hand, represents the number of wave cycles that pass a given point per unit of time, usually measured in Hertz (Hz).
The relationship between wavelength and frequency is defined by the equation: c = λν, where c is the speed of light in a vacuum. This equation highlights the inverse relationship: as wavelength increases, frequency decreases, and vice versa. This relationship is critical to understanding why shorter wavelengths are scattered more effectively in Rayleigh scattering.
Defining Scattering: Redirection of Light
In its simplest form, scattering refers to the redirection of light (or other electromagnetic radiation) as it interacts with matter.
This interaction can occur with particles of various sizes, from atoms and molecules to larger objects.
Scattering does not involve the absorption of light energy; instead, it involves a change in the direction of the light's propagation. The type of scattering that occurs depends on the size of the scattering particle relative to the wavelength of the light. Rayleigh scattering, as we will see, is a specific type of scattering that occurs when light interacts with particles much smaller than its wavelength.
The Mechanism Explained: How Rayleigh Scattering Works
Having established the foundational concepts, we can now turn our attention to the specific mechanisms that govern Rayleigh scattering. This phenomenon arises from the interaction of light with particles much smaller than its wavelength, leading to the characteristic scattering effects observed in our atmosphere.
Interaction with Air Molecules
Rayleigh scattering predominantly occurs when sunlight interacts with air molecules, primarily nitrogen (N2) and oxygen (O2), in the Earth's atmosphere.
These molecules, significantly smaller than the wavelengths of visible light, act as scattering centers, redirecting the light in various directions. The efficiency of this scattering process is heavily dependent on the wavelength of the incident light, a crucial factor in determining the color of the sky.
Inducing an Oscillating Dipole Moment
The interaction between light and air molecules begins with the oscillating electric field of the light wave. This electric field induces an oscillating dipole moment in the air molecule.
In essence, the electric field causes the positively charged nucleus and the negatively charged electrons within the molecule to separate slightly, creating a temporary electric dipole.
This induced dipole then oscillates at the same frequency as the incident light, effectively turning the air molecule into a tiny antenna that radiates electromagnetic radiation (light) in all directions.
The Inverse Fourth Power Law: Wavelength Dependence
The intensity of the scattered light exhibits a strong dependence on the wavelength of the incident light.
Specifically, the intensity of the scattered light is inversely proportional to the fourth power of the wavelength (Intensity ∝ 1/λ4). This relationship, derived from Rayleigh's scattering formula, is paramount in explaining the blue color of the sky.
Shorter wavelengths, such as those corresponding to blue and violet light, are scattered much more efficiently than longer wavelengths, such as red and orange light.
As sunlight enters the atmosphere, the shorter wavelengths are scattered more intensely in all directions, resulting in the pervasive blue hue that we observe. This preferential scattering of shorter wavelengths is the definitive hallmark of Rayleigh scattering.
Rayleigh vs. the Rest: Distinguishing from Other Optical Phenomena
Having established the foundational concepts, we can now turn our attention to the specific mechanisms that govern Rayleigh scattering. However, Rayleigh scattering is not the only game in town when it comes to light's interaction with matter. To fully appreciate its unique characteristics, it is crucial to differentiate it from other related optical phenomena, such as Mie scattering, absorption, and transmission. This section will dissect these distinctions, providing clarity and context to our understanding of Rayleigh scattering.
Rayleigh Scattering vs. Mie Scattering: A Matter of Size
Perhaps the most common point of confusion lies between Rayleigh and Mie scattering. Both are scattering phenomena, but they differ significantly in terms of the size of the scattering particles relative to the wavelength of light.
Rayleigh scattering, as previously discussed, occurs when light interacts with particles much smaller than its wavelength. Air molecules, being significantly smaller than the wavelengths of visible light, are a prime example.
Mie scattering, on the other hand, arises when the scattering particles are comparable in size to or larger than the wavelength of light.
Think of water droplets in clouds, or particulate matter in smog. These larger particles scatter light in a more complex manner, with the intensity and direction of scattering being less dependent on wavelength compared to Rayleigh scattering.
This difference in wavelength dependence has profound implications. Mie scattering, unlike Rayleigh scattering, scatters all wavelengths of light relatively equally. This is why clouds, composed of water droplets that induce Mie scattering, appear white.
Absorption vs. Scattering: A Tale of Two Fates
It is also imperative to distinguish scattering from absorption. Scattering is a physical process that redirects light without changing its energy. Light simply changes direction.
Absorption, conversely, is a process where the energy of the light is converted into another form of energy, typically heat.
When light is absorbed, it ceases to exist as light; its energy is transferred to the absorbing material. Different molecules are more prone to absorb light from different parts of the spectrum (the exact wavelengths that are absorbed, and the efficiency of absorption, depend on the atomic structure and molecular configuration of the target molecule).
The interplay between absorption and scattering determines how light propagates through a medium.
A medium that strongly absorbs light will appear dark or opaque, while a medium that strongly scatters light will appear diffuse or translucent.
Transmission: The Uninterrupted Journey
Finally, we must consider transmission. Transmission refers to the passage of light through a medium without significant scattering or absorption. In an ideal transparent medium, light would travel unimpeded, with minimal loss of intensity or change in direction.
Of course, no real-world medium is perfectly transparent. Some degree of scattering and absorption is always present.
The extent to which a medium transmits light depends on its composition, density, and the wavelength of light.
Transparency, therefore, is a relative concept.
In summary, understanding the distinctions between Rayleigh scattering, Mie scattering, absorption, and transmission is crucial for a complete grasp of how light interacts with matter. Each phenomenon plays a unique role in shaping our perception of the world, from the vibrant blue of the sky to the opaque darkness of an absorbing material.
Everyday Manifestations: Seeing Rayleigh Scattering in Action
Having distinguished Rayleigh scattering from other optical phenomena, we can now turn our attention to its observable effects in the world around us. Rayleigh scattering paints the sky with its familiar hues, contributing to both the cerulean daytime canopy and the fiery sunsets that captivate our gaze.
The Azure Sky: A Rayleigh Masterpiece
The most striking manifestation of Rayleigh scattering is undoubtedly the blue color of the sky on a clear day. This phenomenon arises from the preferential scattering of shorter wavelengths of visible light (blue and violet) by air molecules.
Because the intensity of scattered light is inversely proportional to the fourth power of the wavelength (1/λ4), blue light, with its shorter wavelength, is scattered far more effectively than longer wavelengths like red and orange.
Consequently, when we look up at the sky, we perceive the scattered blue light coming from all directions. While violet light is scattered even more intensely, our eyes are less sensitive to violet, and the sun emits less violet light than blue light. This is why the sky appears predominantly blue.
The intensity of this scattering effect also clarifies why the sky appears darker the further we look away from the sun. Near the sun, the intensity of all wavelengths is overwhelmingly high, washing out the preferential blue scattering to some extent. Further away, the blue scattering becomes more dominant.
Sunset Spectacles: A Reddening Revelation
As the sun approaches the horizon during sunset or sunrise, its light must travel through a significantly greater distance of atmosphere to reach our eyes. This extended path length has a profound impact on the colors we perceive.
The increased atmospheric path leads to enhanced Rayleigh scattering, removing much of the blue light from the direct sunlight. The blue light is scattered away in other directions, no longer traveling directly to the observer.
As the blue component is depleted, the longer wavelengths—namely, orange and red—become proportionally more dominant in the remaining direct sunlight.
Therefore, the sun appears increasingly reddish-orange as it sets, and the sky around the setting sun can also display vibrant hues of red, orange, and yellow due to the scattered light.
Factors Influencing Sunset Colors
It's important to note that the intensity and vibrancy of sunset colors can vary significantly depending on atmospheric conditions. A greater concentration of particles in the atmosphere (e.g., dust, pollutants, volcanic ash) can enhance scattering, leading to more spectacular sunsets.
These particles, often larger than the air molecules responsible for Rayleigh scattering, can also contribute to Mie scattering, which scatters light more uniformly across all wavelengths.
This can make sunsets appear more diffuse and less intensely colored. Conversely, exceptionally clean air can result in less dramatic, but still beautiful, sunsets.
Therefore, the beauty and intensity of a sunset are a complex interplay between Rayleigh scattering, atmospheric composition, and viewing conditions, presenting a breathtaking example of physics in action.
The Math Behind the Magic: A Glimpse at Rayleigh's Formula
Having witnessed the beautiful manifestations of Rayleigh scattering in the sky, it's natural to wonder if there's a way to quantify this phenomenon. While a deep dive into the mathematics requires a solid foundation in physics and electromagnetism, we can appreciate the essence of Rayleigh's scattering formula by focusing on its key components and their relationships.
The Core Equation: A Conceptual View
The intensity of scattered light (I) is proportional to:
I ∝ (1/λ4) (1 + cos2θ) (N
**α2)
Let's break down what this means, without getting bogged down in complex derivations.
Unpacking the Key Parameters
The equation reveals the critical factors influencing Rayleigh scattering. It highlights why some colors are scattered more effectively than others.
Wavelength (λ): The Dominant Factor
The most striking aspect of the formula is the inverse fourth-power relationship with wavelength (λ). This means that shorter wavelengths are scattered much more intensely than longer wavelengths.
For example, blue light (shorter wavelength) is scattered about 10 times more strongly than red light (longer wavelength). This explains the blue sky.
Scattering Angle (θ): Directionality Matters
The term (1 + cos2θ) describes the angular distribution of the scattered light. The intensity of scattered light depends on the angle (θ) between the incident light and the direction of observation.
This indicates that scattering is not uniform in all directions.
Number Density (N) and Polarizability (α): Medium Properties
The term (N** α2) represents the properties of the scattering medium (e.g., air). N refers to the number density of the scattering particles (air molecules), and α represents the polarizability of these particles.
Polarizability measures how easily the electron cloud of a molecule is distorted by an electric field. A higher polarizability leads to stronger scattering.
Implications and Significance
Although we've only touched upon the mathematical description, it's clear that the formula provides a powerful tool for understanding and predicting the behavior of light in the atmosphere. By understanding the relationship between intensity, wavelength, particle size, and number density, we gain valuable insights into a wide range of optical phenomena. From the intensity to the direction of scattering.
Video: Rayleigh Scattering: Why is the Sky Blue?
FAQs: Rayleigh Scattering and the Blue Sky
Why is the sky blue and not another color?
The sky is blue because of rayleigh scattering. Rayleigh scattering is the scattering of electromagnetic radiation (of which light is a form) by particles of a much smaller wavelength. This scattering is more effective at shorter wavelengths; blue and violet light are scattered more strongly than other colors.
If violet light is scattered more, why isn't the sky violet?
While violet light is indeed scattered more strongly by rayleigh scattering, the sun emits less violet light than blue light. Also, our eyes are more sensitive to blue. The combination of these factors makes the sky appear blue, even though rayleigh scattering affects violet light more.
Does rayleigh scattering happen everywhere?
Rayleigh scattering occurs anywhere there are particles that are much smaller than the wavelength of light. This is why the effect is noticeable in Earth's atmosphere. Understanding rayleigh scattering sky blue light is key to understanding its effect in planetary atmospheres.
Why are sunsets often red or orange?
During sunset, sunlight travels through more of the atmosphere to reach our eyes. Blue light is scattered away even more, leaving mostly red and orange light to reach us directly. This longer path length through the atmosphere enhances the rayleigh scattering effect, which is why we see the red and orange hues.
So, the next time you're gazing up at that beautiful Rayleigh scattering sky blue, remember it's not just a pretty color; it's a whole physics phenomenon happening right before your eyes! Pretty neat, huh?