Protists Definition: Single-Celled Life Explained

Protists, a diverse group of eukaryotic microorganisms, represent a critical area of study within biology, as their evolutionary history sheds light on the development of more complex life forms. Microscopy, a fundamental tool in biological research, allows scientists to observe the intricate structures and behaviors of these single-celled organisms, furthering our understanding of their functions. The Kingdom Protista, while no longer a formal taxonomic rank, historically encompassed this varied collection of organisms, highlighting the challenge in establishing a precise protists definition due to their diverse characteristics. Research conducted at institutions like the Marine Biological Laboratory continues to explore the ecological roles and genetic diversity of protists, contributing to a more comprehensive view of these foundational members of the microbial world.
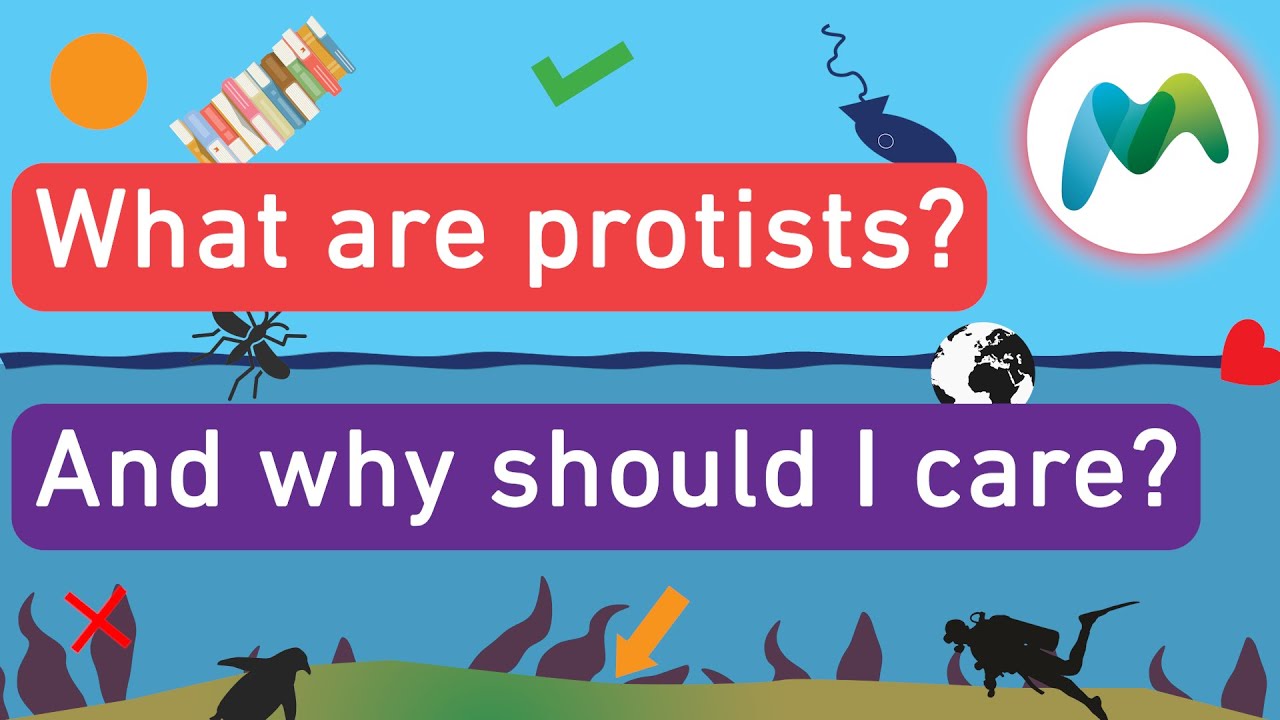
Image taken from the YouTube channel Microbiology Society , from the video titled What are protists? .
Unveiling the Microscopic World of Protists: A Journey into Eukaryotic Diversity
Protists represent a fascinating and ecologically vital, yet often overlooked, domain of life. These organisms, predominantly microscopic, occupy a unique position within the eukaryotic world. Understanding their biology, evolution, and ecological impact is crucial for comprehending the broader tapestry of life on Earth.
Defining the Protists: A Primer on Eukaryotic Microorganisms
At their core, protists are defined as eukaryotic organisms that are not plants, animals, or fungi. This broad definition encompasses a staggering array of unicellular and simple multicellular forms. The defining feature is their eukaryotic cell structure, characterized by a membrane-bound nucleus and other complex organelles.
This cellular organization distinguishes them from prokaryotic bacteria and archaea. Due to their great diversities, protists exhibit vast variances in cellular structure, metabolism, and lifestyle.
A Glimpse into History: From Leeuwenhoek's Observations to Haeckel's Kingdom
The discovery of protists can be traced back to the pioneering work of Anton van Leeuwenhoek, the 17th-century Dutch microscopist. His meticulous observations of microscopic "animalcules" in pond water revealed a hidden world of living organisms.
It wasn't until the 19th century that the German biologist Ernst Haeckel formally established the Kingdom Protista in 1866. Haeckel sought to classify these newly discovered microorganisms, recognizing that they didn't fit neatly into the existing plant or animal kingdoms.
Haeckel's initial classification, while groundbreaking, has been significantly revised over time with the advent of molecular phylogenetics.
The Ecological Significance of Protists: A Foundation for Ecosystems
Protists play myriad roles in the environment, making them indispensable contributors to ecosystem health and function. Many protists are primary producers, harnessing sunlight through photosynthesis to generate organic matter. Diatoms and dinoflagellates, for instance, form the base of aquatic food webs, supporting a vast array of marine life.
Other protists are decomposers, breaking down organic material and recycling nutrients. Still others engage in complex symbiotic relationships, both beneficial and detrimental, with other organisms.
Importantly, some protists are pathogens, causing diseases like malaria and giardiasis, which have significant impacts on human health.
Addressing the Paraphyletic Nature of Protists: A Taxonomic Conundrum
The term "protist" has long been used as a convenient label for eukaryotes that are neither plants, animals, nor fungi. However, modern phylogenetic analyses have revealed that protists do not form a true monophyletic group. In other words, the group "protists" does not include all descendants of a single common ancestor.

Instead, the protists are a paraphyletic group, meaning they include some, but not all, descendants of a common ancestor. This is because the lineages leading to plants, animals, and fungi are nested within the broader protist diversity.
Despite their paraphyletic nature, the term "protist" remains useful for describing this diverse assemblage of eukaryotic microorganisms.
It provides a practical, albeit imperfect, way to group together organisms that share common characteristics and ecological roles.
Understanding the complexities of protist taxonomy is an ongoing challenge, driven by continuous advancements in molecular biology and phylogenetic analysis.
The Evolutionary Story: Endosymbiosis and the Origins of Protists
From the initial definition of protists, it becomes evident that this group isn't merely a collection of simple organisms; it is a living testament to some of the most transformative events in the history of life on Earth. Exploring the evolutionary origins of protists requires a deep dive into the concept of endosymbiosis, a process that fundamentally reshaped the trajectory of cellular evolution and gave rise to the complex eukaryotic cells we know today.
Endosymbiosis: A Symbiotic Revolution
The story of protist evolution is inextricably linked to endosymbiosis, the process by which one organism lives inside another, ultimately leading to a symbiotic relationship. This phenomenon, occurring billions of years ago, is responsible for the origin of two key eukaryotic organelles: mitochondria and chloroplasts.
These organelles, essential for cellular respiration and photosynthesis respectively, were once free-living prokaryotic cells that were engulfed by larger host cells. Instead of being digested, these engulfed prokaryotes established a mutually beneficial relationship with their hosts, gradually evolving into the organelles we recognize today.
The ancestral eukaryote acquired mitochondria first, followed by chloroplasts in the lineage leading to photosynthetic protists and plants. This serial endosymbiosis is a cornerstone of eukaryotic evolution, fundamentally altering the capabilities and complexities of these cells.
Lynn Margulis and the Endosymbiotic Theory
The modern understanding of endosymbiosis owes much to the pioneering work of Lynn Margulis. In the face of considerable initial skepticism, Margulis championed the Endosymbiotic Theory, providing compelling evidence that mitochondria and chloroplasts originated from bacteria.
Her meticulous observations of cellular structures, coupled with the growing body of evidence from molecular biology, ultimately led to the widespread acceptance of this revolutionary concept. Margulis's work not only transformed our understanding of cellular evolution but also highlighted the power of symbiotic relationships in shaping the diversity of life.
Protists: Bridging Prokaryotes and Eukaryotes
Protists occupy a crucial position in the evolutionary narrative, serving as a bridge between prokaryotes and other eukaryotes. While eukaryotes, including protists, possess complex cellular structures and membrane-bound organelles, prokaryotes, such as bacteria and archaea, lack these features.
The endosymbiotic events that gave rise to mitochondria and chloroplasts effectively mark the transition from relatively simple prokaryotic cells to the more intricate eukaryotic cells. Protists, as the earliest diverging lineages of eukaryotes, exhibit a wide range of characteristics that reflect this evolutionary transition.
The diversity of protists provides valuable insights into the intermediate steps of eukaryotic evolution. Some protists possess relatively simple cellular organizations, while others exhibit remarkable complexity, highlighting the progressive evolution of eukaryotic features. This diversity underscores the evolutionary significance of protists in unraveling the origins of eukaryotic life.
Phylogeny and the Tree of Life Project
Understanding the evolutionary relationships among protists and other organisms is a central goal of modern biology. Phylogenetic analysis, which employs molecular data and computational methods, has become an indispensable tool for reconstructing the evolutionary history of life.
The Tree of Life Project is a collaborative effort to build a comprehensive phylogenetic tree encompassing all known organisms. This ambitious project relies heavily on data from protists, which represent a significant portion of eukaryotic diversity and occupy key positions in the tree.
Phylogenetic studies have revealed complex relationships among protists, often challenging traditional classifications based solely on morphology. The ongoing refinement of the Tree of Life continues to reshape our understanding of protist evolution, uncovering novel lineages and shedding light on the processes that have shaped their diversification.
The use of advanced molecular techniques, such as metagenomics and single-cell sequencing, is further revolutionizing our understanding of protist phylogeny, allowing us to explore the genetic diversity of protists in unprecedented detail. These approaches are revealing the hidden diversity of protists in various environments, highlighting their ecological importance and evolutionary significance.
Protist Diversity: A Kaleidoscope of Forms and Functions
From the initial definition of protists, it becomes evident that this group isn't merely a collection of simple organisms; it is a living testament to some of the most transformative events in the history of life on Earth. Exploring the evolutionary origins of protists requires a deeper understanding of the diverse characteristics and functions that define these organisms. Their classification, morphology, nutrition, and motility are as varied as the environments they inhabit, presenting both a challenge and a fascination for biologists.
The Taxonomic Tangle: Challenges in Protist Classification
Classifying protists is not a straightforward task. Their evolutionary relationships are complex and often unclear. The traditional Kingdom Protista, once a convenient catch-all for eukaryotic organisms that weren't plants, animals, or fungi, is now recognized as paraphyletic.
This means that the group does not include all descendants of a single common ancestor. Modern phylogenetic analyses, based on molecular data, have revealed that many protist groups are more closely related to plants, animals, or fungi than they are to other protists.
Consequently, the classification of protists is constantly evolving, with new groups being defined and redefined as our understanding of their evolutionary history improves. This ongoing revision reflects the dynamic nature of scientific knowledge and the challenges of unraveling the intricacies of evolutionary relationships.
Morphological and Genetic Diversity: A Cellular Showcase
Protists exhibit an astonishing range of morphological diversity. From the simple, amorphous shapes of amoebas to the intricate silica shells of diatoms, their cellular structures are remarkably varied.
Some protists are unicellular, existing as single, independent cells. Others are colonial, forming aggregates of cells that may or may not exhibit specialization. Still others are truly multicellular, with differentiated cell types and complex tissue organization.
Their genetic diversity is equally impressive. Protists possess a wide range of genome sizes and structures, reflecting their diverse evolutionary histories and adaptations to different environments. This genetic variation underlies their morphological and physiological diversity, enabling them to thrive in virtually every habitat on Earth.
Nutritional Strategies: Autotrophy, Heterotrophy, and Mixotrophy
Protists have evolved a variety of strategies for obtaining nutrients.
Autotrophy: Harnessing the Power of Sunlight
Many protists are autotrophic, meaning they can synthesize their own food using energy from sunlight. These photosynthetic protists, such as diatoms and dinoflagellates, are primary producers in aquatic ecosystems, converting sunlight into organic matter that forms the base of the food web.
Heterotrophy: Consuming Organic Matter
Other protists are heterotrophic, meaning they obtain nutrients by consuming organic matter. They employ various feeding strategies, including phagocytosis (engulfing particles), pinocytosis (drinking fluids), and osmotrophy (absorbing dissolved organic molecules). Some heterotrophic protists are predators, feeding on bacteria, algae, or other protists. Others are decomposers, breaking down dead organic matter and recycling nutrients.
Mixotrophy: Combining Strategies
Some protists are mixotrophic, meaning they can combine autotrophy and heterotrophy. They can photosynthesize when sunlight is available but also consume organic matter when necessary. This flexibility allows them to thrive in environments where nutrient availability fluctuates.
Locomotion and Motility: Navigating the Microscopic World
Protists exhibit a variety of mechanisms for locomotion and motility.
- Flagella: Long, whip-like appendages that propel the cell through water.
- Cilia: Short, hair-like structures that beat in coordinated waves to move the cell or create currents.
- Pseudopodia: Temporary extensions of the cell membrane that allow the cell to crawl or engulf food particles.
Some protists are non-motile, relying on currents or other organisms to disperse them.
A Glimpse into the Protist World: Notable Examples
The diversity of protists is best illustrated by examining some key examples.
Amoeba: The Shape-Shifting Cell
Amoebas are characterized by their ever-changing shape, using pseudopodia for movement and feeding.
Paramecium: The Ciliated Navigator
Paramecium are covered in cilia, which they use for locomotion and to sweep food into their oral groove.
Euglena: The Photosynthetic Flagellate
Euglena possess flagella for movement and chloroplasts for photosynthesis, exhibiting mixotrophic capabilities.
Diatoms: The Glass-Shelled Algae
Diatoms are unicellular algae with intricate silica shells, playing a crucial role in marine ecosystems.
Dinoflagellates: The Bioluminescent Wonders
Dinoflagellates are often responsible for bioluminescence and can form harmful algal blooms.
Plasmodium: The Malaria Parasite
Plasmodium is an apicomplexan protist that causes malaria, transmitted by mosquitoes.
Giardia: The Intestinal Troublesome
Giardia is a flagellated parasite that causes giardiasis, an intestinal infection.
Life Cycles: Reproduction and Survival Strategies
From the remarkable diversity of protist forms, we transition to an equally fascinating realm: their life cycles. These microscopic organisms have evolved a wide array of reproductive and survival strategies, reflecting their adaptability to diverse and often challenging environments. Understanding these strategies is crucial for comprehending protist ecology and evolution.
Asexual Reproduction: The Foundation of Protist Propagation
Asexual reproduction is a cornerstone of protist propagation. This strategy allows for rapid population growth under favorable conditions.
Binary fission, the most common method, involves a simple cell division resulting in two identical daughter cells. This process is efficient and ensures a quick increase in population size when resources are abundant.
Multiple fission, on the other hand, produces multiple daughter cells simultaneously. This strategy is advantageous when a rapid and widespread dispersal of offspring is needed. Budding, another asexual method, involves the outgrowth of a new individual from the parent cell.
Sexual Reproduction: Genetic Recombination and Evolutionary Potential
While asexual reproduction allows for rapid growth, sexual reproduction introduces genetic recombination, a crucial element for adaptation and evolutionary resilience.
Conjugation, seen in ciliates like Paramecium, involves the temporary fusion of two cells. This allows the exchange of genetic material. This process enhances genetic diversity without necessarily resulting in an immediate increase in population size.
Syngamy, the fusion of gametes, results in a diploid zygote. Meiosis subsequently restores the haploid state. Syngamy introduces new genetic combinations, facilitating adaptation to changing environments and increasing the long-term survival prospects of the protist population.
Cysts: Dormancy as a Survival Imperative
Protists often face harsh environmental conditions. This includes nutrient scarcity, desiccation, or extreme temperatures. To survive these challenges, many protists form cysts – dormant, resistant stages.
Cyst formation involves the encasement of the protist cell within a protective outer layer. This layer shields the organism from external stressors, allowing it to withstand unfavorable conditions.
Once environmental conditions improve, the cyst "excysts." The protist emerges in a metabolically active form. The ability to form cysts is critical for protist survival and dispersal, enabling them to persist in unpredictable environments.
Complex Life Cycles: Integrating Reproduction and Environmental Adaptation
Many protists exhibit complex life cycles that integrate both asexual and sexual reproduction. These cycles often involve multiple stages, each adapted to specific environmental conditions or hosts.
Plasmodium, the causative agent of malaria, exemplifies a complex life cycle involving both mosquito and human hosts. This intricate life cycle includes stages of both asexual and sexual reproduction, each optimized for survival and transmission within its respective host.
The ecological significance of complex life cycles lies in their ability to exploit diverse niches and maximize reproductive success in varied environments. Understanding these cycles is essential for comprehending protist ecology and developing effective strategies for managing protist-related diseases or ecological imbalances.
Ecological Roles: Protists as Key Players in the Environment
From the remarkable diversity of protist forms, we transition to an equally fascinating realm: their life cycles. These microscopic organisms have evolved a wide array of reproductive and survival strategies, reflecting their adaptability to diverse and often challenging environments. Understanding these cycles offers profound insights into their evolutionary success and ecological impact. Now, we turn our attention to the environmental stage upon which protists perform, examining their crucial roles within diverse ecosystems.
Protists, often overlooked, are indispensable components of nearly every ecosystem on Earth. Their roles span from the foundational processes of primary production and nutrient cycling to intricate symbiotic partnerships. Further complicating the picture, certain protists can trigger detrimental effects, demanding a nuanced understanding of their overall impact.
Primary Production and the Aquatic Food Web
Photosynthetic protists, including diatoms and dinoflagellates, are the primary producers in many aquatic environments. Analogous to terrestrial plants, they harness solar energy to convert carbon dioxide and water into organic compounds.
This process forms the base of the aquatic food web, sustaining a vast array of organisms. Their contribution to global oxygen production is substantial, rivaling that of terrestrial forests. Without these microscopic powerhouses, life as we know it in aquatic ecosystems would be unsustainable.
Decomposers and Nutrient Recyclers
Beyond primary production, protists also function as critical decomposers. Present in both soil and aquatic habitats, they break down dead organic matter. This decomposition releases essential nutrients back into the environment.
These nutrients become available for uptake by other organisms, fueling further biological activity. This process is vital for maintaining the balance of ecosystems. Protists are essential to the continual recycling of nutrients.
Symbiotic Relationships: A Spectrum of Interactions
Protists engage in a wide range of symbiotic relationships, demonstrating their adaptability and ecological interconnectedness.
These relationships can be mutualistic, commensalistic, or parasitic, each with distinct consequences for the organisms involved.
Mutualism: Benefits for All
In mutualistic relationships, both the protist and its partner benefit. Consider, for instance, the relationship between certain protists and termites. The protists reside in the termite gut, aiding in the digestion of cellulose from wood.
The termites gain access to essential nutrients, and the protists receive a stable habitat and a constant food supply.
Commensalism: One Benefits, the Other is Unaffected
Commensalism occurs when one organism benefits, while the other is neither harmed nor helped. Some protists live on the surfaces of larger organisms, such as marine invertebrates, obtaining shelter or transportation without affecting their host.
Parasitism: One Benefits, the Other Suffers
Parasitic protists, on the other hand, cause harm to their hosts. These relationships can result in diseases affecting both animals and plants. Examples include Plasmodium, the causative agent of malaria, and Phytophthora, a plant pathogen responsible for devastating agricultural losses.
The Dark Side: Algal Blooms and Parasitic Diseases
While protists play many beneficial roles, some can have significant negative impacts on ecosystems and human health.
Algal Blooms: Environmental Disruptions
Under certain conditions, some protists can experience rapid population growth, leading to algal blooms. These blooms can deplete oxygen levels in the water, harming or killing other aquatic organisms.
Certain species of dinoflagellates produce toxins that accumulate in shellfish, leading to paralytic shellfish poisoning in humans who consume them. The red tides are a visible example.
Parasitic Protists: Threats to Human Health
Several protists are significant human pathogens, causing a range of diseases. Malaria, caused by Plasmodium, remains a major global health challenge.
Giardiasis, caused by Giardia, is a common intestinal infection. African trypanosomiasis (sleeping sickness) caused by Trypanosoma brucei, and amoebic dysentery caused by Entamoeba histolytica, are other examples. These diseases can have severe consequences, particularly in developing countries. Understanding the life cycles and transmission mechanisms of these parasites is crucial for developing effective control and prevention strategies.
Studying Protists: Tools and Techniques for Exploration
From their profound ecological impact, we now turn our attention to the methods scientists employ to unravel the mysteries of protists. Understanding these diverse and often elusive organisms requires a sophisticated toolkit.
This section outlines the essential research methodologies used to study protists, encompassing advanced microscopy, cutting-edge molecular biology techniques, specialized culturing methods, and powerful phylogenetic analysis software. These tools are essential to our understanding.
Microscopy and Imaging Techniques
The ability to visualize protists is fundamental to understanding their morphology, behavior, and interactions with their environment. Microscopy has always been the cornerstone of protistology.
Light Microscopy
Traditional light microscopy remains an invaluable tool. Various techniques enhance contrast and resolution.
Phase contrast and differential interference contrast (DIC) microscopy allow for the observation of live, unstained protists, revealing internal structures and dynamic processes.
Fluorescence microscopy, coupled with fluorescent dyes and antibodies, enables the targeting and visualization of specific cellular components and processes.
Electron Microscopy
For ultrastructural details, electron microscopy is indispensable. Transmission electron microscopy (TEM) provides high-resolution images of internal cellular structures.
Scanning electron microscopy (SEM) reveals intricate surface features. These advanced microscopic techniques offer unparalleled insights.
Advanced Imaging Techniques
Recent advancements in imaging technology have revolutionized protist research. Confocal microscopy allows for the creation of three-dimensional reconstructions of cells and tissues.
Live-cell imaging techniques enable the real-time observation of dynamic processes. These processes include cell division, motility, and feeding behavior.
Molecular Biology Techniques
Molecular biology techniques have become indispensable for studying protist evolution, diversity, and function.
DNA Sequencing and Genomics
DNA sequencing has revolutionized our understanding of protist phylogeny and evolution. Genomic analysis reveals insights into metabolic pathways, gene function, and evolutionary relationships.
Polymerase Chain Reaction (PCR)
PCR is used to amplify specific DNA sequences. This enables the detection and identification of protists in environmental samples.
It also helps in the study of gene expression.
Transcriptomics and Proteomics
Transcriptomics analyzes the complete set of RNA transcripts, revealing gene expression patterns. Proteomics identifies and quantifies proteins, providing insights into cellular function and regulation.
These molecular approaches have significantly advanced our knowledge.
Culturing Techniques
Culturing protists in the laboratory is essential for conducting controlled experiments and studying their physiology.
Axenic and Monoxenic Cultures
Axenic cultures contain only a single species of protist, while monoxenic cultures contain the protist and one bacterial species. Establishing and maintaining these cultures requires sterile techniques and carefully formulated growth media.
Environmental Controls
Temperature, pH, salinity, and light intensity must be carefully controlled to mimic natural conditions. This allows for the optimal growth and reproduction of protists in culture.
High-Throughput Screening
High-throughput screening methods enable the rapid testing of multiple culture conditions. This accelerates the discovery of novel compounds and the optimization of growth parameters.
Phylogenetic Analysis Software
Phylogenetic analysis is essential for reconstructing the evolutionary relationships.
Sequence Alignment
Sequence alignment software, such as MUSCLE and MAFFT, aligns DNA or protein sequences. This identifies regions of similarity and difference.
Tree Building Methods
Tree-building algorithms, such as maximum likelihood and Bayesian inference, construct phylogenetic trees. These trees represent the evolutionary relationships among protists.
Software packages like RAxML and MrBayes are widely used for phylogenetic analysis.
Visualization and Interpretation
Phylogenetic trees are visualized using software such as FigTree. Careful interpretation of these trees is essential for understanding the evolutionary history of protists.
Pioneers of Protistology: Celebrating Key Researchers
From their profound ecological impact, we now turn our attention to the methods scientists employ to unravel the mysteries of protists. Understanding these diverse and often elusive organisms requires a sophisticated toolkit.
This section highlights the monumental contributions of key figures in protistology. Their work has fundamentally shaped our understanding of these ubiquitous and vital organisms.
Thomas Cavalier-Smith: A Paradigm Shift in Protist Systematics
Thomas Cavalier-Smith (1942-2021) stands as a colossus in the field of evolutionary biology and protistology. His career, spanning decades, was marked by a relentless pursuit of clarity in the often murky waters of eukaryotic classification.
His most profound contribution lies in his radical restructuring of the eukaryotic tree of life, challenging conventional wisdom and establishing a new framework based on rigorous phylogenetic analysis.
Cavalier-Smith's work extended far beyond mere taxonomic revisions. He championed the use of ultrastructural data, combined with molecular phylogenies, to reconstruct the evolutionary history of protists.
This integrative approach provided unprecedented insights into the origins and diversification of eukaryotic lineages.
Reclassification and the Rejection of "Protista"
Perhaps Cavalier-Smith's most controversial, yet ultimately influential, act was his dismantling of the Kingdom Protista. He recognized its artificial nature as a paraphyletic group, containing organisms more closely related to other eukaryotic kingdoms than to each other.
He replaced this outdated concept with a series of new kingdoms and supergroups, reflecting a more accurate portrayal of evolutionary relationships. This included the Chromista and Excavata.
This radical reclassification initially faced resistance from the scientific community. However, the weight of evidence supporting Cavalier-Smith's model gradually led to its widespread acceptance.
Today, his framework forms the foundation for most modern classifications of eukaryotes.
Evolutionary Insights: Plastid Evolution and Cellular Complexity
Beyond classification, Cavalier-Smith made significant contributions to our understanding of major evolutionary transitions in protists. He proposed groundbreaking hypotheses regarding the origins of plastids.
His work shed light on the complex processes of endosymbiosis. In particular, his investigations suggested multiple independent events of plastid acquisition in different eukaryotic lineages.
Furthermore, he explored the evolution of cellular complexity in protists, examining the origins of features like flagella and the cytoskeleton.
His insights into the evolution of these features have been crucial for understanding the broader trajectory of eukaryotic evolution. Cavalier-Smith's influence continues to be felt throughout the field.
Contemporary Protist Researchers: Pushing the Boundaries of Knowledge
While Cavalier-Smith's legacy is undeniable, the field of protistology continues to evolve through the work of numerous contemporary researchers. These scientists are building upon his foundations, employing cutting-edge technologies to explore new frontiers in protist biology.
Molecular Ecology and Environmental Protistology
Advances in molecular techniques have revolutionized our ability to study protists in their natural environments. Researchers are now using metagenomics and metabarcoding to assess the diversity and distribution of protist communities in various ecosystems.
This has led to the discovery of numerous previously unknown protist species and a deeper understanding of their ecological roles. For example, scientists are using these tools to study the impact of climate change on protist communities in the oceans.
Others explore the role of protists in nutrient cycling in soil environments.
Genomics and Evolutionary Biology
Genomics is also playing a crucial role in unraveling the evolutionary relationships between protists. By sequencing the genomes of diverse protist species, researchers are gaining insights into the evolutionary history of these organisms.
This provides a deeper understanding of the processes of gene duplication, horizontal gene transfer, and genome reduction. These processes have shaped the evolution of protist genomes.
For example, comparative genomics is shedding light on the evolution of parasitism in protists.
Cell Biology and Physiology
While molecular approaches dominate much of modern protist research, classical cell biological and physiological studies remain essential. Researchers are using advanced microscopy techniques to study the structure and function of protist cells.
They are also investigating the physiological adaptations of protists to extreme environments. This includes studying their responses to stress and limited nutrients.
For example, scientists are exploring the mechanisms by which protists survive in environments with low oxygen levels.
Video: Protists Definition: Single-Celled Life Explained
FAQs: Understanding Protists
Are all single-celled organisms considered protists?
No. While protists are eukaryotic (possessing a nucleus) single-celled organisms, not all single-celled life belongs to this group. Bacteria and archaea are single-celled, but they are prokaryotes, lacking a nucleus. The protists definition specifically requires a eukaryotic cell structure.
What makes protists different from other eukaryotes like plants or animals?
The key difference lies in their level of organization. Unlike plants, animals, and fungi, protists lack complex, multicellular organization. They are mostly unicellular, or if multicellular, lack specialized tissues and organs. This simpler structure is part of the protists definition.
Where are protists typically found?
Protists thrive in aquatic environments, including freshwater, saltwater, and even moist soil. Some can also be found living inside other organisms. These diverse habitats allow for the wide variety seen within the protists definition.
Do protists play an important role in ecosystems?
Absolutely. Protists are vital to many ecosystems. They serve as primary producers (like algae), decomposers, and food sources for other organisms. Some protists are also responsible for diseases. Understanding their roles is crucial to the protists definition and their impact.
So, there you have it! Protists definition might seem a bit technical at first, but once you dive in, you realize these single-celled wonders are everywhere and super important. Keep an eye out for them – you never know where you might spot one!