Pressure Gradient: The Ultimate Explainer You Need to See!
Understanding fluid dynamics often hinges on grasping the concept of a pressure gradient. NASA, for instance, utilizes the understanding of pressure gradient to design efficient spacecraft wings. Atmospheric scientists analyze pressure gradient forces to forecast weather patterns, focusing on the movement of air masses. The difference in pressure across an area defines the pressure gradient, a vital factor in various engineering applications. Ultimately, pressure gradient plays a central role from designing pipelines by companies like Bechtel to understanding how blood flows within the human circulatory system.
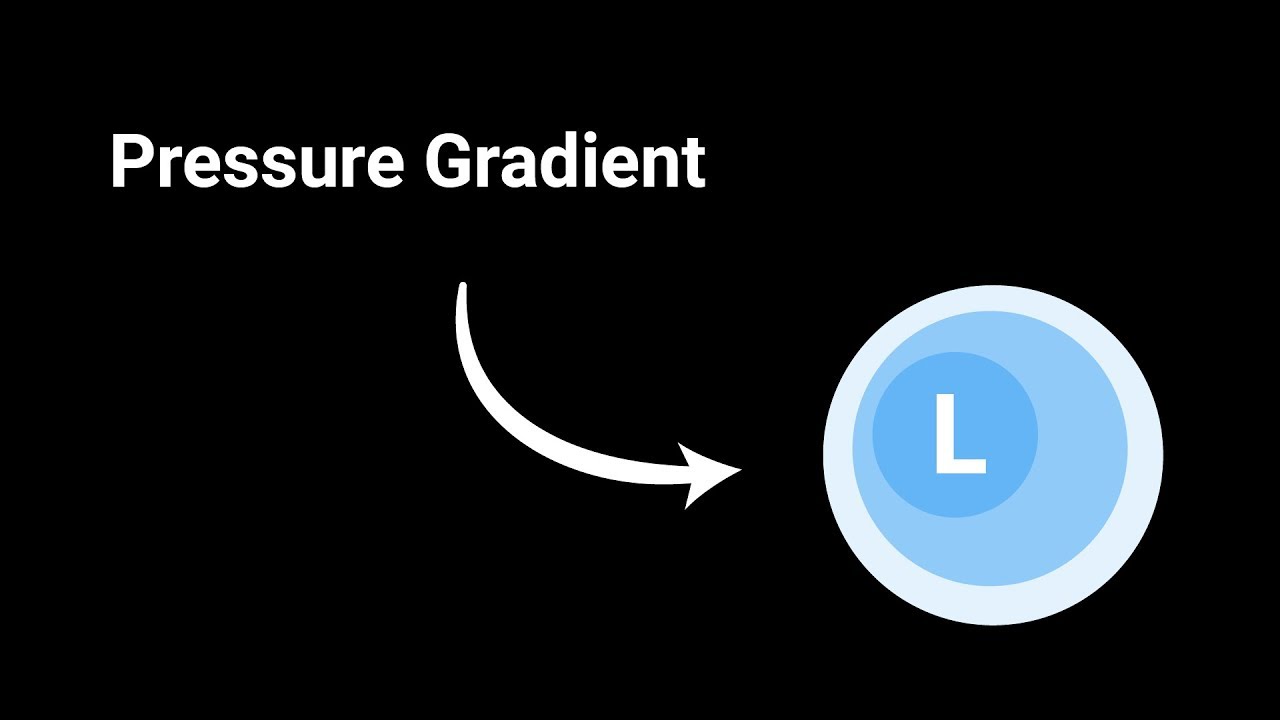
Image taken from the YouTube channel How-To Weather , from the video titled How the Pressure Gradient Force Works .
Remember that time you were walking down the street, and a sudden, unexpected gust of wind nearly knocked you off your feet? That invisible force, that immediate change in air pressure, is a prime example of a pressure gradient in action.
It’s more than just a fleeting annoyance; it’s a fundamental concept that governs everything from the gentle breeze to the most violent storms.
But what exactly is a pressure gradient, and why should you care?
Defining the Pressure Gradient: A Layman's Explanation
In its simplest form, a pressure gradient describes the rate at which air pressure changes over a certain distance. Imagine a map where some areas are labeled "high pressure" and others "low pressure."
The pressure gradient is essentially the steepness of the pressure change between those areas. A steep gradient means a rapid change in pressure over a short distance, while a gentle gradient indicates a more gradual shift.
This "steepness" is what creates force.
Why Understanding Pressure Gradients Matters
The importance of understanding pressure gradients extends far beyond just bracing yourself against a strong wind.
They are critical in fields like:
-
Meteorology: Pressure gradients are the primary drivers of weather patterns. They dictate wind speed and direction, influence the formation of storms, and are crucial for accurate weather forecasting.
-
Engineering: Understanding pressure gradients is vital in designing everything from aircraft wings to pipelines. Engineers must account for pressure differences to ensure structural integrity and efficient fluid flow.
-
Aviation: Pilots rely on their understanding of pressure gradients to navigate safely and efficiently. Pressure differences affect lift, drag, and overall aircraft performance.
-
Climate Science: Global pressure gradients play a significant role in large-scale atmospheric circulation, influencing climate patterns and the distribution of heat around the planet.
In essence, wherever there are fluids (liquids or gases) in motion, pressure gradients are at play, shaping the world around us in profound ways.
Remember that time you were walking down the street, and a sudden, unexpected gust of wind nearly knocked you off your feet? That invisible force, that immediate change in air pressure, is a prime example of a pressure gradient in action.
It’s more than just a fleeting annoyance; it’s a fundamental concept that governs everything from the gentle breeze to the most violent storms. But what exactly is a pressure gradient, and why should you care?
In its simplest form, a pressure gradient describes the rate at which air pressure changes over a certain distance. Imagine a map where some areas are labeled "high pressure" and others "low pressure."
The pressure gradient is essentially the steepness of the pressure change between those areas. A steep gradient means a rapid change in pressure over a short distance, while a gentle gradient indicates a more gradual shift. This "steepness" is what creates force.
The importance of understanding pressure gradients extends far beyond just bracing yourself against a strong wind. They are critical in fields like:
Meteorology: Pressure gradients are the primary drivers of weather patterns. They dictate wind speed and direction, influence the formation of storms, and are crucial for accurate weather forecasting.
Engineering: Understanding pressure gradients is vital in designing everything from aircraft wings to pipelines. Engineers must account for pressure differences to ensure structural integrity and efficient fluid flow.
Aviation: Pilots rely on their understanding of pressure gradients...
Stepping beyond the basic definition, we need to recognize that the pressure gradient isn't just a scalar value. To truly grasp its implications, we need to understand it as a vector.
Deciphering the Pressure Gradient: A Vector Explanation
The pressure gradient, at its core, is a vector quantity. This means it possesses both magnitude and direction. Thinking of it as just a number would be like describing a road trip solely by the distance traveled, without considering which way you're headed.
Magnitude: The Steepness of Change
The magnitude of the pressure gradient tells us how quickly the pressure is changing over a specific distance. A large magnitude signifies a rapid change, a "steep" pressure slope, while a small magnitude indicates a more gradual pressure shift. This magnitude is directly related to the strength of the force it generates.
Direction: The Path of Influence
The direction of the pressure gradient is equally critical. It always points from areas of high pressure towards areas of low pressure. Imagine a ball rolling downhill; it naturally moves from a higher elevation to a lower one. Similarly, the pressure gradient force propels air (or any fluid) from high to low pressure.
Quantifying the Gradient: Change in Pressure Over Distance
The pressure gradient isn't just some abstract concept; it can be quantified. The simplest way to conceptualize the calculation is as follows:
Pressure Gradient = Change in Pressure / Distance
Let's break that down:
- Change in Pressure: This is the difference in pressure between two points. It is measured in Pascals (Pa) or millibars (mb).
- Distance: This is the distance between the two points where the pressure is measured. It is measured in meters (m) or kilometers (km).
Therefore, the pressure gradient is typically expressed in units like Pascals per meter (Pa/m) or millibars per kilometer (mb/km).
This calculation provides the magnitude of the pressure gradient. Remember, the direction is always implied: from high to low pressure.
The Pressure Gradient Force: Always From High to Low
The pressure gradient is intrinsically linked to the pressure gradient force. This force, as the name suggests, is the force that arises due to the pressure difference. And its direction is unwavering: it always points from regions of high pressure to regions of low pressure.
This force is fundamental to understanding fluid dynamics and, most notably, weather patterns. It's the initial impetus that sets air in motion, creating wind. Without a pressure gradient, there would be no pressure gradient force, and the atmosphere would be largely stagnant. The steeper the pressure gradient, the stronger the force, and the faster the air will move.
Stepping beyond the basic definition, we need to recognize that the pressure gradient isn’t just a static concept. It's a dynamic force that breathes life into fluid motion. This is where fluid dynamics comes into play, revealing the pressure gradient as a central player in how liquids and gases behave.
Pressure Gradients in Motion: Fluid Dynamics in Action
Pressure gradients are not merely interesting meteorological phenomena. They are fundamental drivers in the world of fluid dynamics. This branch of physics explores how liquids and gases move, and pressure gradients are at the heart of it all. Understanding their influence is key to grasping the complexities of fluid flow, from the simplest of systems to the most intricate engineering designs.
The Pressure Gradient: The Prime Mover of Fluids
At its core, fluid dynamics is about understanding how and why fluids move. A pressure gradient creates a force that acts upon a fluid, prompting it to accelerate from areas of high pressure to areas of low pressure.
The steeper the gradient, the stronger the force, and the faster the fluid will accelerate. Without a pressure difference, a fluid will remain stationary, at equilibrium. It is this imbalance that sets things in motion.
Shaping the Flow: How Pressure Gradients Influence Patterns
Beyond just initiating movement, pressure gradients dictate the intricate patterns of fluid flow. These gradients are responsible for everything from smooth, laminar flow to turbulent, chaotic motion.
Consider how fluids navigate bends in a pipe. The pressure on the outside of the curve is typically higher than on the inside. This difference in pressure forces the fluid to turn, creating a curved flow path. Complex interactions like these, governed by pressure gradients, shape the beautiful and often unpredictable patterns we see in fluid motion.
Real-World Examples: From Wings to Waterways
To truly appreciate the impact of pressure gradients, let's consider some real-world applications:
Airflow Over an Airplane Wing
The lift generated by an airplane wing is a classic example of pressure gradients in action. The curved shape of the wing forces air to travel faster over the top surface than the bottom.
This difference in speed results in lower pressure above the wing and higher pressure below. This pressure difference creates an upward force (lift), allowing the plane to defy gravity.
Water Flowing Through a Pipe
Even in a seemingly simple scenario like water flowing through a pipe, pressure gradients play a crucial role. A pressure difference between the inlet and outlet drives the flow.
The pressure is higher at the inlet, pushing the water through the pipe towards the lower pressure at the outlet. Obstructions or changes in pipe diameter create localized pressure variations, influencing the flow patterns.
Everyday Examples
- Ventilation Systems: Relies on pressure gradients created by fans to circulate fresh air, removing stale air.
- The Human Circulatory System: Blood flow is driven by pressure differences created by the heart.
These examples are just a glimpse into the ubiquitous nature of pressure gradients in fluid dynamics. From engineering design to natural phenomena, understanding these forces is essential to unraveling the complexities of the world around us.
Pressure gradients, therefore, are not merely interesting meteorological phenomena. They are fundamental drivers in the world of fluid dynamics. This branch of physics explores how liquids and gases move, and pressure gradients are at the heart of it all. Understanding their influence is key to grasping the complexities of fluid flow, from the simplest of systems to the most intricate engineering designs. It shouldn't be a surprise then that these same forces are responsible for driving weather patterns across the globe.
Pressure Gradients and Weather: The Meteorological Connection
The relationship between pressure gradients and meteorology is undeniable. Pressure gradients are the unsung heroes behind almost every weather event we experience. They are the invisible hand that shapes our daily forecasts. Understanding this connection provides a deeper appreciation for the dynamics of the atmosphere. It highlights how simple differences in air pressure can translate into complex and often dramatic weather patterns.
The Prime Mover: Wind Creation
At its most fundamental, the pressure gradient is responsible for the creation of wind. Air naturally flows from areas of high pressure to areas of low pressure. This movement is nature's way of attempting to equalize the pressure imbalance.
The greater the difference in pressure between two locations, the stronger the pressure gradient force. This results in faster-moving winds. Conversely, a weak pressure gradient will lead to gentle breezes or even calm conditions. Wind, in essence, is the atmosphere's response to a pressure gradient.
Highs, Lows, and the Pressure Gradient Force
High-pressure and low-pressure systems are the cornerstones of weather forecasting. They represent regions of relatively higher or lower atmospheric pressure compared to their surroundings. The pressure gradient force plays a pivotal role in the behavior of air within and around these systems.
Air spirals outward from high-pressure systems as it attempts to move toward areas of lower pressure. This outward movement is deflected by the Coriolis effect. As a result, in the Northern Hemisphere, high-pressure systems are associated with clockwise rotation. They bring stable conditions and clear skies.
Conversely, air spirals inward toward low-pressure systems as it rushes to fill the pressure void. This inward movement is also affected by the Coriolis force, causing a counter-clockwise rotation in the Northern Hemisphere. Low-pressure systems are often associated with rising air, cloud formation, and precipitation.
The strength of the pressure gradient directly influences the intensity of these rotational patterns. A strong pressure gradient around a low-pressure system can fuel powerful storms, while a weak gradient around a high-pressure system might result in stagnant air.
Visualizing the Gradient: The Role of Isobars
Meteorologists use weather maps to visualize pressure patterns and gradients. Isobars, lines connecting points of equal atmospheric pressure, are a crucial tool in this visualization. The spacing of isobars reveals the strength of the pressure gradient.
Closely spaced isobars indicate a strong pressure gradient and, therefore, stronger winds. Widely spaced isobars indicate a weak pressure gradient and lighter winds. By analyzing isobar patterns, meteorologists can quickly assess the potential for windy conditions and predict the direction of airflow.
Isobars paint a picture of the atmospheric landscape, allowing forecasters to see the "hills" and "valleys" of pressure. This allows them to understand how the wind will flow across the terrain. These lines are, in effect, a visual representation of the forces that drive our weather.
Pressure gradients, therefore, are not merely interesting meteorological phenomena. They are fundamental drivers in the world of fluid dynamics. This branch of physics explores how liquids and gases move, and pressure gradients are at the heart of it all. Understanding their influence is key to grasping the complexities of fluid flow, from the simplest of systems to the most intricate engineering designs. It shouldn't be a surprise then that these same forces are responsible for driving weather patterns across the globe.
High vs. Low: Understanding Pressure Systems
The atmosphere is in constant flux, a dynamic interplay of forces seeking equilibrium. Among these forces, pressure gradients reign supreme, dictating air movement and shaping the character of our weather. To truly understand weather patterns, we must delve into the nature of high-pressure and low-pressure systems, the very engines that drive atmospheric circulation.
High-Pressure Systems: Zones of Stability
High-pressure systems, often associated with fair weather, are regions where the atmospheric pressure is higher than the surrounding areas. Air within these systems descends, a process known as subsidence.
As air descends, it compresses and warms, inhibiting cloud formation.
This is why high-pressure systems are typically characterized by clear skies, light winds, and stable atmospheric conditions. These systems act as atmospheric "shields," deflecting incoming weather disturbances.
The descending air also contributes to a stable air mass. This means that vertical air movement is suppressed.
Consequently, pollutants and moisture are trapped near the surface, sometimes leading to stagnant air and reduced visibility.
Low-Pressure Systems: Brewing Storms
In stark contrast to their high-pressure counterparts, low-pressure systems are associated with rising air and unstable weather conditions. These systems are characterized by lower atmospheric pressure than their surroundings.
As air converges towards the center of a low-pressure area, it rises, expands, and cools.
This cooling leads to condensation and the formation of clouds and precipitation.
Low-pressure systems are dynamic and often bring about unsettled conditions, including rain, snow, thunderstorms, and strong winds.
The intensity of a low-pressure system can range from a mild trough of low pressure bringing scattered showers to a powerful hurricane wreaking havoc.
The Pressure Gradient's Role: Driving Air Movement
The pressure gradient force is the primary driver of air movement in both high- and low-pressure systems.
In high-pressure systems, air flows outward from the center towards areas of lower pressure.
However, due to the Coriolis effect (the deflection of moving objects due to Earth's rotation), this outflow is deflected to the right in the Northern Hemisphere and to the left in the Southern Hemisphere. This deflection creates a clockwise circulation around high-pressure systems in the Northern Hemisphere and a counterclockwise circulation in the Southern Hemisphere.
Conversely, in low-pressure systems, air flows inward from areas of higher pressure.
The Coriolis effect again deflects this inflow, resulting in a counterclockwise circulation around low-pressure systems in the Northern Hemisphere and a clockwise circulation in the Southern Hemisphere. This inward spiral of air towards the center of a low-pressure system is what fuels rising air motion and the development of stormy weather.
Understanding how the pressure gradient interacts with the Coriolis effect is critical for predicting wind patterns and forecasting weather events associated with these systems.
High and low-pressure systems, while defining atmospheric zones, are only part of the story. The atmosphere is a complex and constantly changing system and the real magic happens when meteorologists put their knowledge of pressure gradients into action, using them as a key ingredient in weather forecasting.
Predicting the Future: Pressure Gradients in Weather Forecasting
The pressure gradient isn't just a theoretical concept; it's a working tool for predicting the weather. Modern weather forecasting relies heavily on sophisticated computer models, and pressure gradients are a fundamental component of these calculations.
Pressure Gradients in Weather Models
Weather models are complex simulations of the atmosphere that solve equations governing air movement, temperature, and moisture.
The pressure gradient force is a critical input into these models, providing information about the forces driving air motion.
By incorporating pressure gradient data from various sources, including surface observations, weather balloons, and satellites, models can simulate how air will move in the future. This allows them to forecast wind patterns, storm development, and other weather phenomena.
These models use complex mathematical equations to simulate the interactions of pressure gradients with other atmospheric factors.
These equations include the influence of topography, temperature gradients, and the Earth's rotation to project future weather conditions.
Isobars: A Meteorologist's Compass
Isobars, those familiar lines on weather maps connecting points of equal pressure, provide a visual representation of pressure gradients. The closer together the isobars, the steeper the pressure gradient, and therefore, the stronger the wind.
Meteorologists analyze isobar patterns to determine the direction and speed of the wind.
Closely packed isobars indicate strong winds, while widely spaced isobars suggest lighter winds.
By observing the shape and movement of isobar patterns, forecasters can anticipate the arrival of weather fronts, the development of storms, and shifts in wind direction. The curvature of isobars also provides clues about areas of converging and diverging air, which are important for forecasting precipitation.
The Limits of Pressure: Acknowledging Complexity
While pressure gradients are crucial for weather forecasting, it's vital to acknowledge their limitations. Weather prediction is not solely based on pressure, as the atmosphere is a complex interplay of various elements.
Other factors, such as temperature, humidity, the Coriolis effect, and topography, all play significant roles.
The Coriolis effect, caused by the Earth's rotation, deflects moving air masses, affecting wind direction, especially over long distances.
Topography, like mountains and valleys, can significantly alter local wind patterns.
Temperature differences create density variations, leading to thermal winds that interact with pressure-driven winds.
To achieve accurate forecasts, meteorologists must integrate information from all these sources, recognizing that pressure gradients are just one piece of the puzzle.
High and low-pressure systems, while defining atmospheric zones, are only part of the story. The atmosphere is a complex and constantly changing system and the real magic happens when meteorologists put their knowledge of pressure gradients into action, using them as a key ingredient in weather forecasting.
Wind's Driving Force: The Pressure Gradient Connection
The pressure gradient is more than just a pretty line on a weather map; it's the fundamental engine that drives wind. Understanding this connection unlocks a deeper appreciation for the forces shaping our daily weather. The stronger the pressure gradient, the more powerful the resulting wind.
Pressure Gradient and Wind Speed: A Direct Link
The relationship between pressure gradient strength and wind speed is remarkably direct. A steeper pressure gradient, indicated by closely spaced isobars, signifies a more substantial force pushing air from high to low pressure.
Conversely, a weaker pressure gradient, with isobars further apart, translates to lighter winds. This simple principle explains why some days are calm and breezy, while others bring gale-force winds.
Beyond the Gradient: Modifying Factors
While the pressure gradient sets wind into motion, its direction and speed are subject to other significant influences. Two of the most important are the Coriolis effect and friction.
The Coriolis Effect: Earth's Spin
The Coriolis effect, caused by the Earth's rotation, deflects moving air to the right in the Northern Hemisphere and to the left in the Southern Hemisphere. This deflection is most pronounced over long distances and significantly impacts large-scale wind patterns.
The Coriolis effect explains why winds don't simply blow straight from high to low pressure. Instead, they curve, creating spiral patterns around high and low-pressure systems.
Friction: Grounding the Wind
Friction, particularly near the Earth's surface, also plays a crucial role in modifying wind. As wind interacts with trees, buildings, and terrain, it slows down and changes direction.
The effect of friction is most noticeable in the lower atmosphere. Higher up, where friction is minimal, winds tend to be stronger and more closely aligned with the pressure gradient and Coriolis effect.
Real-World Examples: Localized Wind Patterns
The interplay of pressure gradients, the Coriolis effect, and friction creates a variety of localized wind patterns, each with unique characteristics.
Sea Breezes and Land Breezes
Sea breezes and land breezes are excellent examples of localized winds driven by pressure gradients created by temperature differences between land and water. During the day, land heats up faster than the sea, creating a pressure gradient that draws cool air from the sea inland, resulting in a sea breeze.
At night, the land cools more quickly, reversing the pressure gradient and causing a land breeze to blow from the land out to sea.
Mountain Winds
Mountain winds are another type of localized wind influenced by topography and temperature differences. During the day, mountain slopes heat up, causing air to rise and creating a valley breeze.
At night, the slopes cool, causing air to sink and creating a mountain breeze. These winds can significantly impact local weather conditions.
Pressure Gradients in the Real World: Applications and Examples
We've explored the fundamental nature of pressure gradients and their pivotal role in weather forecasting, observing how they drive wind and shape atmospheric systems. But the influence of pressure gradients extends far beyond the realm of meteorology. Let's delve into some real-world examples.
Weather Patterns and Climate Dynamics
Pressure gradients are not just responsible for our daily weather; they also govern large-scale atmospheric circulation patterns that influence global climate.
The Hadley, Ferrel, and Polar cells, for instance, are driven by pressure differences resulting from uneven solar heating across the Earth's surface. These cells dictate prevailing wind patterns and rainfall distribution around the globe.
The Intertropical Convergence Zone (ITCZ), a region of low pressure near the equator, is another prime example. This zone is characterized by rising air, heavy rainfall, and converging trade winds driven by pressure gradients.
These large-scale pressure systems directly impact regional climates, influencing everything from monsoon seasons to desert formation. Understanding these dynamics is critical for climate modeling and predicting future climate change scenarios.
Industrial Applications: Ventilation Systems
Pressure gradients play a crucial role in various industrial applications, most notably in ventilation systems.
These systems rely on creating controlled pressure differences to move air, removing pollutants and ensuring a healthy and safe working environment.
For example, exhaust fans create a low-pressure zone, drawing air and contaminants away from workers.
Careful design of ventilation systems, based on principles of pressure gradients, is essential in industries ranging from manufacturing to mining, and even laboratory settings.
Aviation: Lift Generation
The principles of pressure gradients are fundamental to the science of aviation, especially the concept of lift.
An aircraft wing is designed to create a pressure difference between its upper and lower surfaces.
The curved upper surface forces air to travel a longer distance, resulting in lower pressure, while the relatively flat lower surface experiences higher pressure.
This pressure difference generates an upward force – lift – that counteracts gravity and allows the aircraft to fly.
Understanding and manipulating pressure gradients around airfoils is critical for aircraft design, ensuring efficient and safe flight.
Other Relevant Examples
Beyond weather, industry, and aviation, pressure gradients manifest in various other fields:
-
Fluid Transport: Pipelines rely on pressure gradients to transport liquids and gases over long distances. Pumps are used to create pressure differences that drive the flow.
-
Medical Devices: Devices like inhalers and nebulizers utilize pressure gradients to deliver medication to the lungs.
-
Natural Ventilation: Building design often incorporates natural ventilation strategies, utilizing pressure differences caused by wind or temperature variations to circulate fresh air.
-
Hydroelectric Power: Dams create a pressure difference due to water height, which drives turbines to generate electricity.
These examples illustrate the ubiquitous nature of pressure gradients and their vital role in numerous aspects of our lives.
Video: Pressure Gradient: The Ultimate Explainer You Need to See!
Frequently Asked Questions About Pressure Gradients
Here are some common questions about pressure gradients and their importance in understanding fluid dynamics and weather patterns.
What exactly is a pressure gradient?
A pressure gradient is simply the change in pressure over a certain distance. It indicates how rapidly pressure is increasing or decreasing in a given direction. Think of it as the slope of pressure – a steeper "slope" means a stronger pressure gradient.
Why are pressure gradients important?
Pressure gradients are the driving force behind many natural phenomena. In fluids like air and water, a pressure gradient creates a force that pushes the fluid from areas of high pressure to areas of low pressure, leading to movement and flow.
How does a pressure gradient affect wind?
Wind is directly caused by pressure gradients in the atmosphere. Air moves from high-pressure zones to low-pressure zones, creating wind. The stronger the pressure gradient, the stronger the wind. Closely spaced isobars (lines of equal pressure) on a weather map indicate a strong pressure gradient and thus, strong winds.
What is the difference between a strong and a weak pressure gradient?
A strong pressure gradient means that there is a large difference in pressure over a short distance. This results in a strong force and, consequently, a fast flow (like a strong wind or current). Conversely, a weak pressure gradient means the pressure changes slowly over a longer distance, resulting in a weaker force and slower flow.
And there you have it – hopefully, this cleared up any confusion around pressure gradient! Feel free to experiment and explore how pressure gradient impacts the world around you. Now go on and put that newfound knowledge to good use!