Potassium Reactivity: The Complete Guide You Need!
Understanding potassium reactivity is fundamental in numerous scientific disciplines. Electrochemistry provides the framework for analyzing potassium's highly reactive nature, explaining its eager oxidation. This inherent reactivity often necessitates careful handling within a laboratory setting, especially when dealing with its explosive reaction with water. Exploring the principles outlined by Linus Pauling, particularly concerning electronegativity, illuminates why potassium readily forms ionic bonds. Furthermore, the application of potassium reactivity in fertilizer production highlights its essential role in supporting plant growth by providing necessary nutrients.
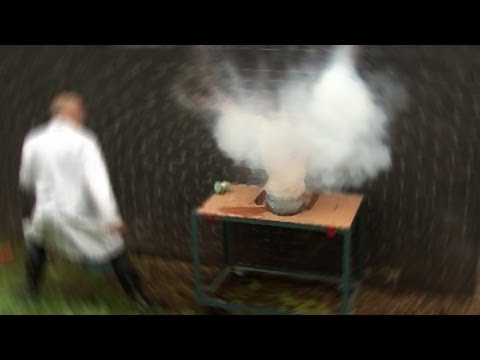
Image taken from the YouTube channel Periodic Videos , from the video titled Potassium in Water (reaction only) .
Potassium, symbolized by K on the periodic table, is far more than just a nutrient vital for plant growth or a component in the electrolyte balance of the human body. It's an element brimming with energetic potential, a key player in the drama of chemical reactions. This often-underappreciated element holds a central role in numerous natural processes and industrial applications.
Potassium: A Vital Element
Potassium's importance spans diverse fields.
In agriculture, it's indispensable for crop development, influencing water retention and nutrient absorption.
Within the human body, potassium ions are critical for nerve function, muscle contraction, and maintaining fluid balance.
Beyond these biological roles, potassium compounds are used in manufacturing fertilizers, soaps, and even glass. The widespread presence of potassium underscores its fundamental role in sustaining life and driving industrial processes.
The Defining Trait: Remarkable Reactivity
What truly sets potassium apart is its exceptional reactivity. As an alkali metal, it readily donates its single valence electron to form positive ions. This eagerness to react manifests in spectacular fashion when potassium encounters common substances like water or air.
The reactions can be so energetic that they release significant heat and light, sometimes even resulting in flames. This high reactivity is a double-edged sword, making potassium incredibly useful in certain applications but also necessitating careful handling and storage.
Understanding Potassium's Reactive Behavior
This guide aims to provide a comprehensive understanding of potassium's reactive behavior. We will explore the underlying causes of its reactivity, delving into its electronic structure and its position in the electrochemical series.
Furthermore, we will examine the practical implications of this reactivity, including safe handling procedures and potential applications in various fields.
By the end of this exploration, you will gain a deeper appreciation for the energetic nature of potassium and its significant role in the world around us.
The reactions can be so energetic that they release significant heat and light, sometimes even resulting in flames. This high reactivity is a double-edged sword, making potassium incredibly useful in certain applications but also necessitating careful handling and storage. It's crucial, therefore, to understand the underlying factors that govern its behavior.
Potassium: An Alkali Metal Deep Dive
To truly grasp potassium's reactive nature, we must first examine its fundamental characteristics. Its position on the periodic table, its relationship to other alkali metals, and, most importantly, its electronic structure all play critical roles in determining its chemical behavior.
Potassium's Place in the Periodic Table
Potassium (K), with an atomic number of 19, resides in Group 1 (also known as Group IA) of the periodic table. This group is famously known as the alkali metals.
Its location immediately reveals several key properties. As a member of Group 1, potassium is a metal, exhibiting the characteristic metallic luster, conductivity, and malleability.
However, it's also a relatively soft metal, easily cut with a knife, unlike many of its transition metal counterparts. The single valence electron, characteristic of all alkali metals, is at the heart of potassium's reactivity.
The Alkali Metal Family
The alkali metals – lithium (Li), sodium (Na), potassium (K), rubidium (Rb), cesium (Cs), and francium (Fr) – form a closely related group. They share similar chemical properties due to their identical valence electron configuration (ns1).
All alkali metals are highly reactive, readily losing their single valence electron to form +1 cations.
However, reactivity increases as you descend the group. This trend is critical to understanding potassium's particular energetic behavior. Potassium is more reactive than lithium and sodium, but less reactive than rubidium and cesium.
This increasing reactivity down the group is primarily attributed to two factors:
- Atomic Size: As you move down the group, the atomic radius increases. The valence electron is further from the nucleus and is shielded by more inner electrons.
- Ionization Energy: The ionization energy, the energy required to remove an electron, decreases down the group, making it easier to lose the valence electron.
Electronic Configuration and Reactivity
Potassium's electron configuration is [Ar] 4s1. The "[Ar]" represents the electron configuration of argon, the noble gas preceding potassium on the periodic table.
This shorthand notation highlights the key point: potassium has one single electron in its outermost (4s) shell. This lone electron is the key to its reactivity.
Ionization energy is a measure of how easily an atom loses an electron. Potassium has a relatively low ionization energy, meaning it readily gives up its 4s1 electron to form a K+ ion.
Electronegativity, on the other hand, is a measure of an atom's ability to attract electrons in a chemical bond. Potassium has a low electronegativity, indicating that it is more likely to donate its electron than to attract electrons from other atoms.
This combination of a single valence electron, low ionization energy, and low electronegativity makes potassium exceptionally prone to reacting with other elements. It readily forms ionic compounds with nonmetals, releasing significant energy in the process.
The alkali metals – lithium (Li), sodium (Na), potassium (K), rubidium (Rb), cesium (Cs), and francium (Fr) – form a closely related group. They share similar chemical properties due to their identical valence electron configuration (ns1).
All alkali metals are highly reactive, readily losing their single valence electron to form +1 cations.
However, it's the nuances of their individual reactions that truly reveal the fascinating complexities of potassium.
The Chemistry of Potassium: Reactions with Common Substances
Potassium's extreme reactivity stems from its eagerness to shed its single valence electron. This eagerness manifests in a variety of chemical reactions, particularly with common substances like water, air, and halogens. Understanding these reactions provides crucial insight into the element's behavior and the energy transformations involved.
Understanding Chemical Reactions
Chemical reactions, at their core, involve the rearrangement of atoms and molecules. Reactants transform into products, and this transformation is almost always accompanied by a change in energy.
Exothermic reactions release energy into the surroundings, often in the form of heat and light, while endothermic reactions require energy input to proceed.
Potassium's reactions are typically exothermic, reflecting its strong drive to achieve a stable electron configuration.
Potassium and Water: A Vigorous Encounter
The reaction of potassium with water is perhaps one of its most dramatic demonstrations of reactivity.
When potassium metal is dropped into water, it immediately ignites, producing a lilac flame.
The reaction is so energetic that the heat generated is sufficient to ignite the hydrogen gas produced.
The balanced chemical equation for this reaction is:
2K(s) + 2H₂O(l) → 2KOH(aq) + H₂(g) + Heat
This equation tells us that solid potassium reacts with liquid water to produce potassium hydroxide (a strong base) in aqueous solution and hydrogen gas.
The "Heat" notation indicates the significant energy release, making this a highly exothermic process.
The Role of Potassium Hydroxide
Potassium hydroxide (KOH), also known as caustic potash, is a highly alkaline compound. It's a powerful base and contributes to the overall corrosiveness of the reaction products.
The Slow Dance: Potassium's Oxidation in Air
Unlike its rapid reaction with water, potassium's interaction with air is a slower, more gradual process.
In dry air, potassium tarnishes relatively slowly, forming a layer of potassium oxide (K₂O) on its surface.
However, in moist air, the reaction is accelerated due to the presence of water vapor. The potassium reacts with both oxygen and water vapor to form a mixture of potassium hydroxide (KOH) and potassium carbonate (K₂CO₃).
The formation of potassium carbonate is a result of the reaction of potassium hydroxide with carbon dioxide in the air.
This oxidation process is why potassium is typically stored under mineral oil or in an inert atmosphere to prevent it from reacting with the surrounding environment.
Halogen Reactions: A Fiery Embrace
Potassium reacts vigorously with halogens (fluorine, chlorine, bromine, and iodine) to form potassium halides.
These reactions are highly exothermic, often producing intense light and heat.
For example, the reaction with chlorine can be represented as:
2K(s) + Cl₂(g) → 2KCl(s) + Heat
Potassium chloride (KCl), common table salt, is the product of this reaction. The "Heat" indication highlights the energy released, further emphasizing the exothermic nature.
The reactivity decreases as we move down the halogen group (fluorine being the most reactive and iodine being the least).
Potassium's reaction with fluorine is so rapid and energetic that it can be difficult to control.
Unveiling Oxidation and Reduction
Potassium's reactions are classic examples of oxidation-reduction (redox) reactions.
Oxidation involves the loss of electrons, while reduction involves the gain of electrons.
In the reaction with water, potassium is oxidized, losing an electron to form a K+ ion. Simultaneously, hydrogen in water is reduced, gaining an electron to form hydrogen gas (H₂).
Similarly, in the reaction with chlorine, potassium is oxidized, and chlorine is reduced.
The driving force behind these redox reactions is potassium's low ionization energy, its ability to readily lose its valence electron.
By understanding these individual reactions and the underlying principles of oxidation and reduction, we gain a much clearer and more profound understanding of potassium's place in the chemical world.
The reactions of potassium with water, air, and halogens showcase its inherent eagerness to participate in chemical transformations. But what are the underlying reasons for this exceptional reactivity? The answer lies in understanding the fundamental factors that govern potassium's chemical behavior.
Factors Influencing Potassium Reactivity
Potassium distinguishes itself through its remarkable eagerness to engage in chemical reactions. This section delves into the primary influences that contribute to potassium's high level of reactivity, with particular emphasis on its placement within the reactivity series and the crucial role played by its valence electrons.
Potassium's Place in the Reactivity Series
The reactivity series is a hierarchical arrangement of metals based on their relative ease of oxidation. A metal's position in the reactivity series is a direct indicator of its tendency to lose electrons and form positive ions.
Potassium occupies a prominent position near the top of this series, signifying its high reactivity. This placement highlights potassium's strong tendency to lose its valence electron. Elements positioned higher in the series exhibit a greater driving force for oxidation compared to those lower down.
Comparing Potassium to Other Metals
Compared to metals like iron or copper, potassium readily undergoes oxidation. This means that potassium will displace these metals from their compounds in a chemical reaction. For instance, if potassium were to react with copper oxide, it would readily steal the oxygen, forming potassium oxide and leaving elemental copper behind.
This illustrates potassium's greater "desire" to form chemical bonds. The further apart two metals are in the reactivity series, the more vigorous the reaction between them will be.
The Role of Valence Electrons
The electronic configuration of an atom is a fundamental determinant of its chemical properties. Potassium's electron configuration ([Ar] 4s1) reveals that it possesses a single valence electron in its outermost shell.
This solitary electron is relatively easy to remove, as its removal leads to a stable, noble gas configuration. The energy required to remove this electron, known as the ionization energy, is comparatively low for potassium. This low ionization energy is a critical factor contributing to its high reactivity.
Ionization Energy and Reactivity
The lower the ionization energy, the more readily an atom loses an electron. Potassium's low ionization energy facilitates the formation of the K+ ion. This ease of ionization explains its tendency to react vigorously with substances that can readily accept an electron, such as water, oxygen, and halogens.
Shielding Effect
The inner electrons in potassium shield the valence electron from the full positive charge of the nucleus. This shielding effect reduces the effective nuclear charge experienced by the valence electron, making it even easier to remove. The combination of low ionization energy and the shielding effect contribute to potassium's place at the top of the reactivity series.
Electron Configuration Stability
Atoms "strive" to achieve a stable electron configuration, typically resembling that of a noble gas. By losing its single valence electron, potassium achieves the stable electron configuration of argon. This drive toward stability provides the thermodynamic impetus for potassium's reactions.
In summary, potassium's high reactivity is a consequence of its position in the reactivity series, its low ionization energy, and the shielding effect, all stemming from its unique electronic structure. These factors combine to make potassium an exceptionally reactive element, readily participating in a variety of chemical reactions.
The further apart two metals are in the reactivity series, the more vigorous the reaction will be when they interact. This explains why potassium reacts so dramatically with substances like water and halogens, showcasing its pronounced tendency to shed its valence electron and achieve a more stable electronic configuration. Understanding this drive towards stability is essential, but equally critical is recognizing the inherent dangers associated with such potent reactivity.
Hazards and Safe Handling of Potassium
Potassium's high reactivity, while fascinating from a chemical standpoint, presents significant hazards that demand meticulous handling and storage procedures. Its eagerness to react with air and water, the very substances that are ubiquitous in most environments, makes it a substance that can quickly become hazardous if not managed correctly. Ignoring the inherent dangers of potassium can lead to severe consequences, ranging from minor burns to explosions.
Understanding the Risks: Flammability and Explosion Hazards
Potassium's reaction with water is not merely a gentle fizzing; it's a highly exothermic process that generates substantial heat and hydrogen gas. The heat produced can easily ignite the hydrogen, resulting in a violent explosion. The reaction can be summarized as:
2K(s) + 2H₂O(l) → 2KOH(aq) + H₂(g) + Heat
Even the moisture present in the air can initiate a reaction, leading to gradual oxidation and the formation of potassium superoxide (KO₂), which is itself a powerful oxidizer and can react explosively with organic materials. Therefore, potassium should never be exposed to open air or moisture.
The flammability of potassium is another significant concern. Fine particles or shavings of potassium can spontaneously ignite in air, especially if the humidity is high. This is due to the rapid oxidation process, which generates enough heat to reach the autoignition temperature of potassium.
Essential Safety Precautions for Handling Potassium
Given the inherent dangers, strict safety protocols are essential when handling potassium. Personal Protective Equipment (PPE) is paramount.
This includes:
- Eye protection: Safety goggles or a face shield are crucial to protect against splashes or flying debris.
- Skin protection: Impervious gloves, such as nitrile or neoprene, should always be worn to prevent skin contact.
- Protective clothing: A lab coat or apron made of flame-resistant material is recommended to shield clothing from potential spills or fires.
In addition to PPE, the following safety measures should be observed:
- Work in a well-ventilated area: This helps to dissipate any hydrogen gas that may be generated during handling.
- Avoid contact with water: Potassium should never come into contact with water or any water-containing solutions.
- Use appropriate tools: Use clean, dry metal tools (such as a knife or spatula) for cutting or handling potassium.
- Have a Class D fire extinguisher readily available: Class D extinguishers are specifically designed for metal fires and are essential for controlling potassium fires. Never use water on a potassium fire.
Best Practices for Potassium Storage
Proper storage is just as crucial as safe handling. The goal is to isolate potassium from air and moisture to prevent unwanted reactions.
The recommended storage method is to:
- Store potassium under an inert liquid: Typically, a mineral oil or kerosene is used to create an air-excluding barrier. The potassium should be completely submerged in the oil.
- Use a tightly sealed container: The container should be made of a material that is impermeable to air and moisture, such as glass or metal.
- Label the container clearly: The label should clearly identify the contents as "Potassium - Flammable Solid - Reacts Violently with Water" and include appropriate hazard symbols.
- Store in a cool, dry place: Avoid storing potassium in areas with high humidity or temperature fluctuations.
Safe Handling in an Inert Environment
For more complex manipulations or experiments involving potassium, working in an inert environment is highly recommended.
This typically involves using:
- Gloveboxes: Gloveboxes are sealed enclosures filled with an inert gas, such as argon or nitrogen. This allows for manipulation of potassium without exposure to air or moisture.
- Schlenk lines: Schlenk lines are specialized glassware setups that allow for reactions to be carried out under an inert atmosphere.
When working in an inert environment, it's still important to follow all the other safety precautions mentioned above, including wearing appropriate PPE and having a Class D fire extinguisher readily available. It is also crucial to routinely verify the integrity of the inert atmosphere to ensure that it remains free of oxygen and moisture. Regular maintenance and monitoring are keys to safe operations.
The flammability of potassium is another critical aspect of its reactivity. Even without the presence of water, potassium can ignite spontaneously in air, especially when finely divided or exposed to elevated temperatures. This poses a considerable fire hazard in laboratory and industrial settings, necessitating rigorous fire safety protocols. The dangers are undeniable, yet this very reactivity, the same trait that makes potassium hazardous, also underpins its utility in a surprising array of applications.
Real-World Applications of Potassium Reactivity
Potassium, often perceived as a hazardous element due to its vigorous reactions, also possesses a surprising number of real-world applications. Its inherent reactivity, the very characteristic that necessitates careful handling, is precisely what makes it valuable in various industrial and scientific fields. From agriculture to energy storage, potassium's unique properties are harnessed to drive innovation and improve existing technologies.
Potassium in Agriculture: A Vital Nutrient
Perhaps the most well-known application of potassium is in agriculture. Potassium is one of the three macronutrients essential for plant growth (nitrogen, phosphorus, and potassium), playing a crucial role in various physiological processes within plants.
It is involved in:
- Water regulation
- Nutrient transport
- Enzyme activation
- Photosynthesis
Potassium fertilizers, such as potassium chloride (KCl) and potassium sulfate (K₂SO₄), are widely used to enhance crop yields and improve the overall health and resilience of plants. Adequate potassium levels in soil lead to stronger stems, improved disease resistance, and enhanced fruit and grain development.
The impact of potassium on agricultural productivity cannot be overstated, as it directly contributes to global food security.
Potassium Superoxide: Supplying Oxygen in Confined Environments
Potassium superoxide (KO₂) is an interesting compound formed when potassium reacts with oxygen. This yellow-orange solid has the remarkable ability to release oxygen and absorb carbon dioxide, making it invaluable in closed-system life support systems.
It reacts with carbon dioxide and water vapor according to the following simplified reactions:
4KO₂(s) + 2CO₂(g) → 2K₂CO₃(s) + 3O₂(g)
4KO₂(s) + 2H₂O(g) → 4KOH(s) + 3O₂(g)
This property makes KO₂ essential in:
- Self-contained breathing apparatus (SCBA): Used by firefighters and miners.
- Rebreather systems: Employed in submarines and spacecraft.
The chemical reactivity of potassium superoxide ensures a continuous supply of breathable air in environments where external oxygen sources are limited or unavailable.
Potassium Alloys: Enhancing Material Properties
Potassium can be alloyed with other metals to create materials with enhanced or novel properties. For example, NaK, an alloy of sodium and potassium, is liquid at room temperature and possesses excellent heat transfer capabilities. This makes it suitable as a coolant in nuclear reactors, where efficient heat removal is crucial for safe operation.
Another notable application is the use of potassium in certain types of batteries. Potassium-ion batteries are being explored as potential alternatives to lithium-ion batteries, particularly due to the abundance and lower cost of potassium. While still in the developmental stage, potassium-ion batteries hold promise for large-scale energy storage applications.
Other Scientific and Industrial Uses
Beyond the major applications discussed above, potassium compounds find use in a variety of other scientific and industrial processes. Potassium hydroxide (KOH), also known as caustic potash, is a strong base used in:
- The production of liquid soaps
- The manufacturing of various chemicals.
Potassium salts are used in:
- The production of glass
- As electrolytes in batteries.
The reactivity of potassium and its compounds, when carefully controlled and understood, enables a wide range of technological advancements and industrial applications.
Video: Potassium Reactivity: The Complete Guide You Need!
Frequently Asked Questions About Potassium Reactivity
This FAQ section clarifies common points about potassium reactivity discussed in our guide. We hope this helps address any lingering questions you may have.
Why is potassium so reactive?
Potassium is highly reactive because it only has one electron in its outermost shell. This electron is easily lost, leading to the formation of a stable potassium ion with a positive charge. This eagerness to lose an electron is the core reason behind potassium reactivity.
What happens when potassium reacts with water?
When potassium reacts with water, it vigorously produces hydrogen gas and potassium hydroxide. This reaction is exothermic, generating enough heat to ignite the hydrogen gas, often resulting in a purple flame due to the potassium ions in the flame. This illustrates the intense potassium reactivity.
How should potassium be stored safely?
Due to its high reactivity, potassium should be stored under mineral oil or kerosene. This prevents it from reacting with atmospheric moisture and oxygen, both of which can cause dangerous reactions. Proper storage is critical for managing potassium reactivity.
Is potassium reactivity affected by temperature?
Yes, higher temperatures generally increase the rate of potassium reactivity. The increased kinetic energy of the potassium atoms at higher temperatures means they collide with other substances more frequently and with greater force, leading to faster and more vigorous reactions.
So, there you have it! Everything you need to know about potassium reactivity. Now go forth and, you know, be careful with that potassium!