Polystyrene IR Spectrum: Decoding Plastics Like a Pro!
Infrared Spectroscopy, a vital analytical technique, provides detailed insights into the molecular composition of materials. The National Institute of Standards and Technology (NIST) maintains comprehensive spectral databases, which are essential resources for identifying substances based on their unique IR signatures. Polystyrene, a widely used polymer in applications ranging from packaging to insulation, possesses a distinct infrared spectrum that serves as its fingerprint. The Fourier Transform Infrared (FTIR) spectrometer is the instrumental workhorse for obtaining these spectra, enabling precise analysis. Analyzing the polystyrene IR spectrum allows experts in the field of Materials Science, such as polymer chemists, to confirm the presence, purity, or modifications of the material, ensuring quality control and aiding in research and development.
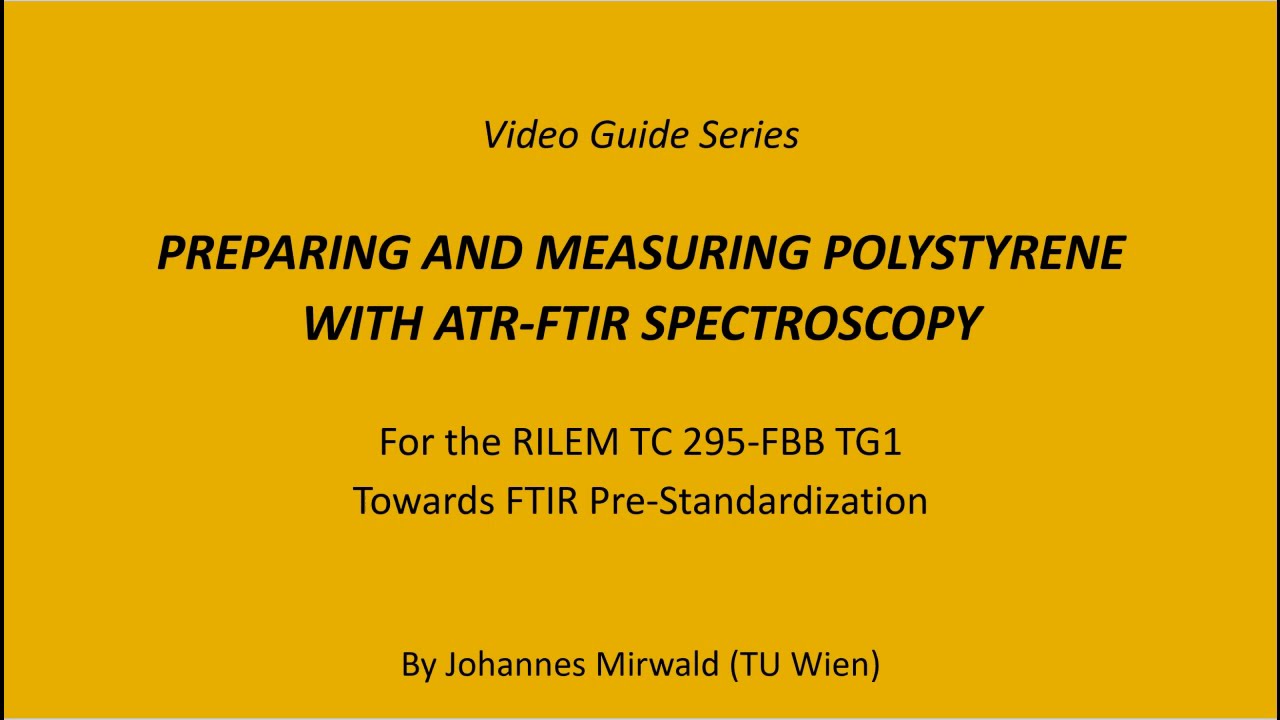
Image taken from the YouTube channel Johannes Mirwald , from the video titled Preparing and Measureing Polystyrene with ATR-FTIR Spectroscopy .
Decoding Polystyrene with Infrared Spectroscopy
Polystyrene, a ubiquitous synthetic polymer, permeates modern life. From disposable cutlery to protective packaging and electronic housings, its versatility and cost-effectiveness have cemented its place in countless applications. Understanding the properties of this material is crucial for optimizing its use, ensuring product quality, and even developing new applications. Material characterization techniques, particularly Infrared (IR) Spectroscopy, play a vital role in achieving this understanding.
The Pervasive Presence of Polystyrene
Polystyrene's popularity stems from its ease of processing, lightweight nature, and relatively low cost. It can be found in various forms, including:
-
General Purpose Polystyrene (GPPS): A rigid, brittle, and transparent form used in packaging and disposable items.
-
High Impact Polystyrene (HIPS): A tougher, more impact-resistant version, often used in appliance housings and toys.
-
Expanded Polystyrene (EPS): A lightweight, cellular form used for insulation and protective packaging (commonly known as Styrofoam).
Its diverse applications highlight the importance of understanding its properties and behavior under different conditions.
The Significance of Material Characterization
Material characterization is the process of probing a material's structure, properties, and performance characteristics. It provides critical insights that inform material selection, processing optimization, and failure analysis. In the context of polymers like polystyrene, characterization helps to:
-
Verify Material Identity: Ensure that the material being used is indeed polystyrene and not a counterfeit or substitute.
-
Assess Purity: Detect the presence of impurities or additives that may affect the material's properties.
-
Evaluate Degradation: Monitor the effects of aging, weathering, or chemical exposure on the polymer's structure and performance.
-
Optimize Processing: Fine-tune manufacturing processes to achieve desired material properties and product performance.
Infrared Spectroscopy: A Window into Molecular Structure
Infrared (IR) Spectroscopy is a powerful analytical technique that exploits the interaction between infrared radiation and the vibrational modes of molecules. When a molecule absorbs infrared radiation, it undergoes vibrational transitions. The specific frequencies at which these transitions occur are highly characteristic of the molecule's structure and chemical bonds. By analyzing the IR Spectrum – a plot of absorbance or transmittance versus wavenumber (related to frequency) – we can gain valuable information about the composition and structure of a material.
For polymers, IR spectroscopy serves as a fingerprinting technique, allowing for the identification of the polymer type, the presence of specific functional groups, and even the degree of crystallinity. It is particularly valuable for:
-
Identifying the characteristic aromatic ring vibrations in polystyrene.
-
Analyzing the C-H stretching and bending modes that define the polymer backbone.
-
Detecting the presence of additives or contaminants.
Thesis Statement
This article provides a comprehensive guide to understanding and interpreting the IR Spectrum of Polystyrene. By delving into the characteristic peaks and bands, correlating spectral features with the polymer's chemical structure, and exploring real-world applications, this guide aims to equip readers with the knowledge and skills necessary to confidently analyze polystyrene using Infrared Spectroscopy.
Decoding the composition and characteristics of polystyrene requires a specific analytical lens, and that lens is Infrared (IR) Spectroscopy. But before we can dissect a polystyrene spectrum, we need to understand the fundamental principles that underpin this powerful technique. Understanding how IR spectroscopy works, specifically how molecules interact with infrared radiation, is crucial. Only then can we unravel the story told by the spectrum's peaks and valleys.
IR Spectroscopy: Unveiling Molecular Vibrations
At its core, Infrared Spectroscopy is a technique that exploits the interaction between infrared radiation and the vibrational modes of molecules. Each molecule possesses a unique set of vibrational frequencies, much like a fingerprint, that can be excited by absorbing IR radiation. This absorption pattern is then translated into a spectrum, revealing key information about the molecule's structure and composition.
Molecular Interaction with Infrared Radiation
Molecules are not static entities; their atoms are constantly in motion, vibrating around their equilibrium positions. These vibrations can be categorized into different modes, such as stretching (changes in bond length) and bending (changes in bond angle).
Infrared radiation, a form of electromagnetic radiation, carries energy. When IR radiation of a specific frequency matches the frequency of a particular vibrational mode within a molecule, the molecule absorbs that energy. This absorption causes the amplitude of the vibration to increase.
However, not all molecular vibrations are IR active. For a vibration to absorb infrared radiation, it must cause a change in the dipole moment of the molecule. In simpler terms, there needs to be a shift in the distribution of electron density during the vibration. This explains why homonuclear diatomic molecules like O₂ or N₂ do not exhibit IR spectra, as their vibrations do not alter their dipole moment.
Wavenumber, Absorbance, and Transmittance: Deciphering the Spectrum
The results of IR Spectroscopy are usually displayed as a graph of absorbance or transmittance versus wavenumber. Understanding these terms is essential for interpreting IR spectra.
-
Wavenumber (cm⁻¹): Wavenumber is the reciprocal of the wavelength, commonly expressed in units of inverse centimeters (cm⁻¹). It is directly proportional to the frequency of the infrared radiation and, therefore, to the energy of the vibration. Higher wavenumbers correspond to higher energy vibrations. Wavenumber is the most common unit used on the X-axis of an IR spectrum.
-
Absorbance (A): Absorbance is a measure of the amount of infrared radiation absorbed by the sample at a particular wavenumber. A higher absorbance value indicates that more radiation was absorbed at that frequency, suggesting a stronger interaction between the molecule and the IR radiation.
-
Transmittance (T): Transmittance is the fraction of infrared radiation that passes through the sample without being absorbed. It is inversely related to absorbance (T = 1/A). A high transmittance value indicates that little radiation was absorbed at that frequency.
These three values are all linked. When a molecule absorbs radiation (high absorbance), less radiation passes through the sample (low transmittance).
The FTIR Spectrometer: A Workhorse of Analytical Chemistry
The most common type of IR spectrometer used today is the Fourier Transform Infrared (FTIR) spectrometer. Unlike older dispersive IR spectrometers that use a prism or grating to separate the different frequencies of infrared radiation, FTIR spectrometers use an interferometer.
The interferometer creates an interference pattern of all the infrared frequencies simultaneously. This interference pattern, called an interferogram, contains information about all the frequencies absorbed by the sample.
A Fourier transform is then applied to the interferogram to convert it into a conventional IR spectrum of absorbance or transmittance versus wavenumber.
FTIR spectrometers offer several advantages over dispersive instruments, including:
-
Higher Sensitivity: FTIR spectrometers can collect data more quickly and efficiently, resulting in better signal-to-noise ratios.
-
Improved Accuracy: The use of an interferometer allows for more precise measurement of wavenumbers.
-
Faster Analysis: FTIR spectrometers can acquire a complete spectrum in a matter of seconds.
In essence, the FTIR spectrometer acts as a sophisticated tool that shines infrared light through a sample, measures which frequencies are absorbed, and then generates a spectrum that reveals the molecular composition of the material.
The dance of infrared radiation and molecular vibrations has set the stage. Now, it's time to spotlight the star of our show: the polystyrene IR spectrum itself. By dissecting its unique landscape of peaks and valleys, we can gain a profound understanding of this ubiquitous polymer.
The Polystyrene IR Spectrum: A Detailed Analysis
Polystyrene's IR spectrum is a rich tapestry woven from the vibrational signatures of its constituent chemical bonds and functional groups. The most prominent features arise from the aromatic ring and the various C-H bonds present in its structure. Understanding the precise locations and intensities of these peaks is key to identifying and characterizing polystyrene samples.
Decoding Characteristic Peaks and Bands
The polystyrene IR spectrum is not just a random collection of signals. Each peak and band corresponds to a specific vibrational mode within the molecule. Let's delve into the most important ones.
Aromatic Ring Vibrations
The presence of the aromatic ring, or benzene ring, is a defining characteristic of polystyrene, and its vibrational modes give rise to a series of distinctive peaks in the IR spectrum. These peaks are typically observed in the following regions:
-
3000-3100 cm-1: Aromatic C-H Stretching. These peaks are due to the stretching vibrations of the C-H bonds directly attached to the aromatic ring.
They are generally weaker in intensity compared to aliphatic C-H stretches.
-
1600 cm-1, 1580 cm-1, and 1493 cm-1: Aromatic Ring Stretching. These peaks arise from the in-plane stretching vibrations of the carbon-carbon bonds within the aromatic ring.
The exact positions and relative intensities of these peaks can provide information about the substitution pattern on the ring.
-
699 cm-1 and 756 cm-1: Aromatic C-H Out-of-Plane Bending. These strong absorptions are characteristic of monosubstituted benzene rings. Their presence confirms the attachment of the styrene unit to the polymer backbone.
The intensity of these peaks is particularly useful for quantitative analysis.
C-H Bond Vibrations
In addition to the aromatic C-H stretches, polystyrene also exhibits peaks corresponding to the C-H bonds in the polymer backbone.
-
2850-3000 cm-1: Aliphatic C-H Stretching. These peaks are due to the stretching vibrations of the C-H bonds in the polymer backbone.
They are typically broader and more intense than the aromatic C-H stretches.
-
1452 cm-1 and 1493 cm-1: C-H Bending. These peaks arise from the bending vibrations of the C-H bonds in the polymer backbone.
These peaks often overlap with the aromatic ring stretching bands, making their interpretation more complex.
-
906 cm-1 : Vinyl C-H out-of-plane bend. This peak is a result of the vinyl group present in the styrene monomer.
Correlation with Chemical Structure
The beauty of IR spectroscopy lies in its ability to connect spectral features with the underlying chemical structure of the molecule. In the case of polystyrene, the presence and positions of the aromatic ring and C-H bond peaks directly reflect its chemical composition.
By analyzing the spectrum, one can confirm the presence of the styrene monomer unit and its characteristic aromatic ring. The relative intensities of the peaks can also provide information about the polymer's molecular weight and branching.
The Importance of Functional Group Identification
While the aromatic ring and C-H bonds are the dominant features in the polystyrene IR spectrum, it's crucial to consider the potential presence of other functional groups. These groups might arise from additives, impurities, or degradation products.
For example, the presence of carbonyl groups (C=O) could indicate oxidation or the presence of plasticizers. Similarly, hydroxyl groups (O-H) might suggest the presence of moisture or alcohol-based additives. Careful analysis of these additional peaks can provide valuable insights into the overall composition and quality of the polystyrene sample.
The dance of infrared radiation and molecular vibrations has set the stage. Now, it's time to spotlight the star of our show: the polystyrene IR spectrum itself. By dissecting its unique landscape of peaks and valleys, we can gain a profound understanding of this ubiquitous polymer.
Decoding Real-World Polystyrene IR Spectra: Variations and Impurities
While the idealized polystyrene IR spectrum offers a solid foundation for analysis, real-world samples often present complexities that demand a nuanced approach. Variations in polymer grade, processing methods, and the presence of impurities or additives can all influence the spectral fingerprint, requiring careful interpretation. Let's explore these deviations and how to navigate them effectively.
Understanding Spectral Variations in Polystyrene
Polystyrene isn't a monolithic entity. Different grades and processing techniques lead to subtle, yet detectable, variations in the IR spectrum.
These variations often reflect differences in molecular weight distribution, tacticity (stereochemical arrangement), or the presence of intentionally added components.
General-purpose polystyrene (GPPS), for example, might exhibit slightly different peak intensities compared to high-impact polystyrene (HIPS), which contains added rubber modifiers for improved impact resistance.
Similarly, processing techniques like injection molding or extrusion can induce changes in polymer chain orientation, subtly affecting the intensities and shapes of certain bands.
Recognizing these potential sources of variation is crucial for accurate interpretation. Comparing spectra against reference libraries of known polystyrene grades can be immensely helpful.
Identifying Impurities and Additives
IR spectroscopy is a powerful tool for detecting impurities or additives within a polystyrene sample.
These foreign substances, whether unintentionally introduced contaminants or deliberately added stabilizers, plasticizers, or colorants, leave their own distinct spectral signatures, superimposed onto the polystyrene backbone.
For example, the presence of a carbonyl group (C=O) stretching band at around 1720 cm-1 might indicate the presence of an ester-based plasticizer.
Similarly, the appearance of peaks characteristic of inorganic fillers like talc or calcium carbonate can reveal their presence in the polymer matrix.
Spectral subtraction techniques, where a reference spectrum of pure polystyrene is subtracted from the sample spectrum, can help isolate the contributions of these impurities and additives.
Case Studies: Examining Different Polystyrene Types
Let's consider a few example spectra to illustrate these variations.
-
General-Purpose Polystyrene (GPPS): Typically exhibits a clean spectrum with prominent aromatic and C-H stretching/bending bands.
-
High-Impact Polystyrene (HIPS): May show additional peaks or broader bands due to the presence of butadiene rubber, used to enhance impact resistance.
-
Polystyrene with Flame Retardants: Expect to see peaks associated with the specific flame retardant compound used, such as halogenated or phosphorus-containing species.
By comparing these spectra, we can appreciate how the presence of different additives or modifications affects the overall spectral profile.
The Role of Styrene Monomer
While typically minimized in well-processed polystyrene, residual styrene monomer can sometimes be detected in the IR spectrum.
The styrene monomer exhibits characteristic peaks that differ slightly from the polymerized polystyrene, particularly in the fingerprint region (below 1500 cm-1).
A strong vinyl group absorption near 910 cm-1 could indicate the presence of unreacted monomer.
The presence of styrene monomer can be indicative of incomplete polymerization or degradation processes. It is a valuable indicator for quality control.
The ability to discern the subtle nuances within a polystyrene IR spectrum, distinguishing between polymer grades or pinpointing trace contaminants, elevates this analytical technique from a mere laboratory exercise to a powerful tool with profound implications across diverse industries. Let's delve into the tangible applications of polystyrene IR spectral analysis, illustrating its value in quality control, material identification, and degradation studies.
Practical Applications of Polystyrene IR Spectral Analysis
IR spectroscopy, far from being confined to academic research, plays a pivotal role in real-world scenarios involving polystyrene. From ensuring product consistency to unraveling the complexities of polymer degradation, its applications are vast and varied.
Quality Control in Polystyrene Manufacturing
In the realm of polystyrene manufacturing, consistency is paramount. IR spectroscopy serves as a vigilant guardian of product quality, providing a rapid and reliable means of verifying the chemical composition of each batch.
By comparing the spectrum of a newly synthesized batch against a reference standard, manufacturers can quickly identify any deviations from the desired specifications.
This allows for immediate corrective action, preventing the production of substandard material and ensuring that the final product meets stringent quality standards.
Furthermore, IR spectroscopy can be used to monitor the concentration of additives, such as plasticizers or flame retardants, ensuring that they are present in the correct proportions.
This precise control over the material's composition translates directly into improved product performance and reliability.
Identifying Polystyrene in Polymer Blends and Microplastics
Polystyrene is frequently blended with other polymers to achieve specific performance characteristics. Determining the composition of these blends can be challenging, but IR spectroscopy provides a straightforward solution.
By analyzing the spectrum of a polymer blend, it is possible to identify the presence of polystyrene and, in some cases, even quantify its concentration.
This information is invaluable in a variety of applications, including:
- Reverse engineering of existing products
- Developing new polymer blends with tailored properties
- Identifying sources of environmental contamination, especially concerning microplastics.
The increasing awareness of plastic pollution has spurred research into the identification and quantification of microplastics in environmental samples.
IR spectroscopy is emerging as a key tool in this field, enabling researchers to identify polystyrene microplastics and track their distribution in various ecosystems.
Analyzing Degraded Polystyrene Samples
Polystyrene, like all polymers, is susceptible to degradation over time, particularly when exposed to environmental factors such as ultraviolet radiation, heat, or oxygen.
IR spectroscopy can provide valuable insights into the degradation process, helping researchers and engineers understand how the material's chemical structure changes over time.
By analyzing the spectrum of a degraded polystyrene sample, it is possible to identify the formation of new functional groups, such as carbonyl groups or hydroxyl groups, which are indicative of oxidation or chain scission.
This information can be used to:
- Assess the long-term durability of polystyrene products
- Develop strategies to mitigate degradation
- Design more sustainable materials.
Relevance in Polymer Chemistry and Analytical Chemistry
Beyond its specific applications in polystyrene analysis, IR spectroscopy plays a broader role in polymer chemistry and analytical chemistry.
It serves as a fundamental tool for characterizing new polymers, elucidating their structure, and understanding their properties.
IR spectroscopy also contributes significantly to the development of new analytical techniques and methodologies. Its versatility and sensitivity make it an indispensable tool for any chemist or material scientist working with polymers.
It provides a rapid, non-destructive method for identifying a wide range of organic compounds.
Video: Polystyrene IR Spectrum: Decoding Plastics Like a Pro!
Decoding Polystyrene IR Spectra: Your Questions Answered
Here are some common questions about interpreting polystyrene IR spectra and understanding what they reveal about this widely used plastic.
What are the key peaks to look for in a polystyrene IR spectrum?
When analyzing a polystyrene ir spectrum, focus on peaks around 3060-3085 cm-1 (aromatic C-H stretch), 1600 and 1490 cm-1 (aromatic ring vibrations), and 700 and 750 cm-1 (monosubstituted benzene ring). These are characteristic of the polystyrene structure.
Can an IR spectrum differentiate between different types of polystyrene?
While a basic polystyrene ir spectrum will show the fundamental polystyrene structure, subtle variations, such as the intensity or presence of minor peaks, might hint at differences in molecular weight, tacticity, or the presence of additives. However, more advanced techniques might be needed for definitive differentiation.
What does the absence of expected peaks mean in a polystyrene IR spectrum?
If expected peaks are missing or significantly diminished in a polystyrene ir spectrum, it might indicate the presence of impurities, degradation of the sample, or that the material isn't actually polystyrene. Sample preparation and instrument calibration should be checked.
How does the solvent used for analysis affect the polystyrene IR spectrum?
The solvent used can introduce its own peaks that overlap with or obscure peaks in the polystyrene ir spectrum. It's crucial to choose a solvent that is transparent in the region of interest or to analyze the sample as a solid film if possible to avoid solvent interference.