Physical Traits: Decoding Characteristics
Human behavioral genetics studies explore the degree to which inheritance explains the origin of characteristics, indicating that some physical traits of an individual are inheritable. Mendelian Genetics explains how phenotypes can express or be influenced by underlying genetic predispositions. An individual's Genotype, the total genetic makeup, strongly influences the physical characteristics of a trait. The Human Genome Project, an international scientific research project, has mapped the entire human genome, providing detailed information that aids in the comprehension of the hereditary basis of physical traits and disease susceptibility.
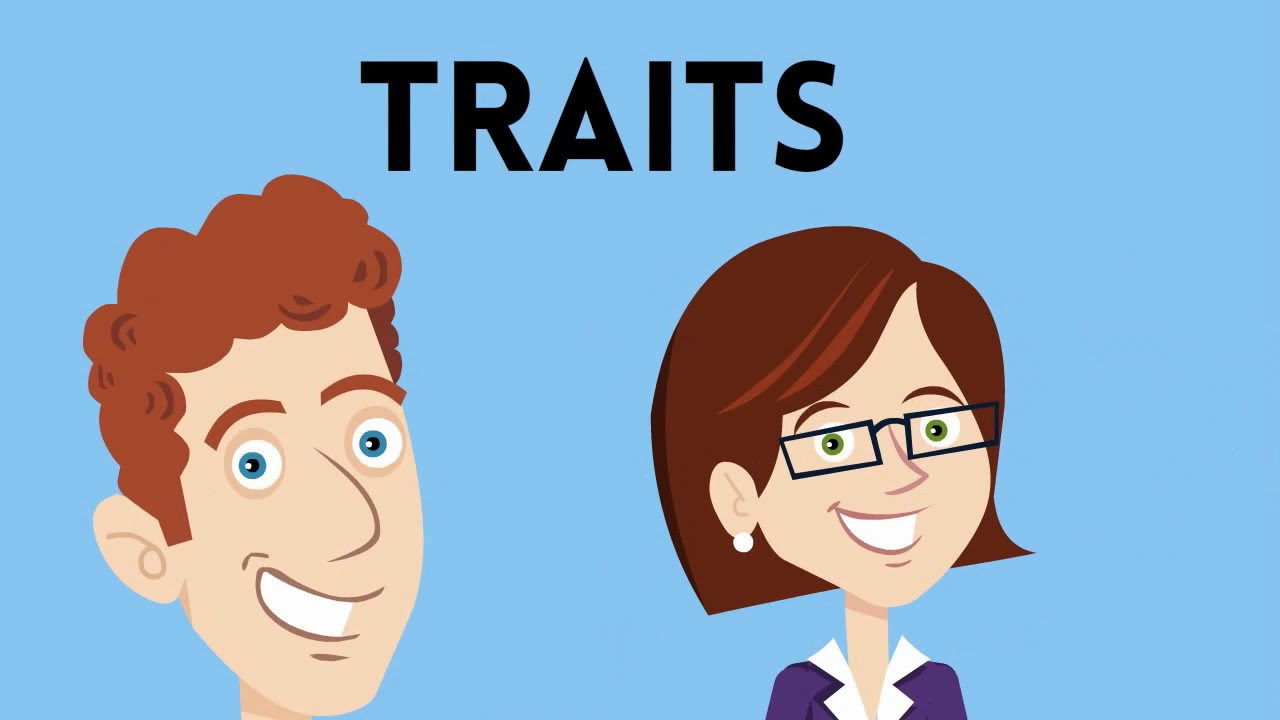
Image taken from the YouTube channel MooMooMath and Science , from the video titled What is a trait?-Genetics and Inherited Traits .
Unraveling the Genetic Blueprint of Physical Traits
The tapestry of human diversity is woven with threads of genetics, each strand representing a physical trait that contributes to our individual uniqueness. From the color of our eyes to the contours of our face, our physical characteristics are, in large part, a reflection of our genetic inheritance. Understanding this intricate relationship between genes and observable traits is a journey into the very essence of what makes us human.
Genetics: The Foundation of Physical Traits
Genetics, the study of heredity and variation, provides the framework for understanding how physical traits are passed down through generations. It is the science that deciphers the code within our DNA, revealing the secrets of our biological inheritance.
At its core, genetics explores the mechanisms by which information is encoded, replicated, and expressed, shaping the physical attributes that define us.
Heredity: Passing Down the Legacy
Heredity is the process by which traits are transmitted from parents to offspring. Through the union of sperm and egg, genetic material is combined, creating a unique blend of characteristics that are inherited by the next generation.
This inheritance is governed by the principles of Mendelian genetics, where genes, the fundamental units of heredity, are passed down according to specific rules. Understanding these rules allows us to predict, to some extent, the likelihood of certain traits appearing in future generations.
Nature vs. Nurture: The Interplay of Genes and Environment
While genetics lays the foundation for our physical traits, it is not the sole determinant. Environmental factors also play a crucial role in shaping our characteristics. From nutrition and exposure to sunlight to lifestyle choices and social interactions, the environment can influence gene expression and modify the development of physical traits.
The interplay between genetics and environment, often referred to as "nature versus nurture," is a dynamic and complex interaction. It is not a matter of one dominating the other, but rather a continuous dialogue between our genes and the world around us.
Exploring the Landscape Ahead
In the sections that follow, we will embark on a comprehensive exploration of the genetic underpinnings of physical traits. We will delve into the foundational principles of genetics, unravel the concepts of phenotype and genotype, and examine the role of epigenetics.
We will also explore the disciplines of genomics and anthropometry, which provide powerful tools for studying the genetic basis of human variation. Finally, we will address the ethical considerations that arise from our growing understanding of the human genome, ensuring that genetic information is used responsibly and ethically.
Foundational Principles: The Building Blocks of Inheritance
To truly grasp the intricate dance between genes and physical traits, we must first establish a solid foundation in the core principles of genetics. This involves understanding how genetic information is transmitted across generations and how it is ultimately expressed to shape our observable characteristics. This section will delve into Mendelian genetics, explore the molecular mechanisms of DNA, elucidate the central dogma of molecular biology, and contextualize genetic variation within the framework of evolution.
Mendelian Genetics: Unveiling the Laws of Inheritance
Gregor Mendel's meticulous experiments with pea plants in the 19th century laid the groundwork for our understanding of heredity. By carefully tracking traits across generations, Mendel formulated laws that govern how genes are passed from parents to offspring. His work, initially overlooked, has become the cornerstone of modern genetics.
Mendel's Experimental Approach
Mendel's brilliance lay in his controlled experimental design. He focused on traits with clearly defined variations (e.g., flower color: purple or white) and used true-breeding plants, which consistently produced the same traits in successive generations. By crossing these plants and observing the resulting offspring, he was able to deduce fundamental principles of inheritance.
Dominant and Recessive Traits
Mendel observed that some traits mask the expression of others. He termed the expressed trait dominant and the masked trait recessive. For instance, if a plant with purple flowers is crossed with a plant with white flowers, and all the offspring have purple flowers, then purple is the dominant trait, and white is recessive.
Segregation and Independent Assortment
Mendel's law of segregation states that each individual carries two copies of each gene (alleles), and these alleles separate during gamete formation, with each gamete receiving only one allele. This ensures that offspring inherit one allele from each parent.
The law of independent assortment states that the alleles of different genes assort independently of one another during gamete formation. This means that the inheritance of one trait does not influence the inheritance of another, assuming the genes for those traits are located on different chromosomes.
Molecular Genetics: The Indispensable Role of DNA
While Mendel's work provided the conceptual framework for inheritance, it was the discovery of DNA's structure that revealed the physical basis of genetic information. DNA, deoxyribonucleic acid, is the molecule that carries the genetic instructions for all living organisms.
The Structure of DNA
DNA is a double helix, resembling a twisted ladder. The sides of the ladder are made of sugar and phosphate molecules, while the rungs are formed by pairs of nitrogenous bases: adenine (A) with thymine (T), and guanine (G) with cytosine (C). This specific pairing is crucial for DNA replication and information transfer.
The Discovery of the Double Helix
The elucidation of DNA's structure was a landmark achievement in science. While James Watson and Francis Crick are often credited with the discovery, it's essential to acknowledge the critical contributions of Rosalind Franklin and Maurice Wilkins. Franklin's X-ray diffraction images provided crucial evidence about DNA's helical structure. Her work, often overlooked, was instrumental in solving the puzzle.
The Central Dogma: From Genotype to Phenotype
The central dogma of molecular biology describes the flow of genetic information within a biological system. It provides a framework for understanding how the information encoded in DNA is used to create proteins, which carry out most of the functions in a cell and ultimately determine our physical traits.
DNA Replication, Transcription, and Translation
DNA replication is the process by which DNA makes copies of itself, ensuring that genetic information is passed on during cell division.
Transcription is the process by which the information encoded in DNA is copied into RNA (ribonucleic acid), specifically messenger RNA (mRNA).
Translation is the process by which the information encoded in mRNA is used to assemble proteins. This process occurs on ribosomes, which read the mRNA sequence and link amino acids together to form a polypeptide chain, which then folds into a functional protein.
Genotype and Phenotype
The genotype refers to the genetic makeup of an organism, the specific alleles it carries for each gene.
The phenotype refers to the observable characteristics of an organism, resulting from the interaction of its genotype with the environment. For example, an individual's genotype might predispose them to a certain eye color, while the actual shade of their eyes is the phenotype.
Evolutionary Context of Genetic Variation
Genetic variation is the raw material for evolution. Without differences in genes, there would be no natural selection, and life as we know it would not exist. Understanding the evolutionary context of genetic variation is essential for comprehending the diversity of physical traits we observe in the natural world.
Natural Selection and Inherited Traits
Charles Darwin's theory of natural selection proposes that individuals with traits that are better suited to their environment are more likely to survive and reproduce, passing on those advantageous traits to their offspring.
Over time, this process can lead to the evolution of new species. Natural selection acts on the phenotype, but it is the underlying genetic variation that allows for phenotypic differences to arise.
Genetic Mutations: The Source of Novelty
Mutations are changes in the DNA sequence. They can arise spontaneously during DNA replication or be induced by environmental factors such as radiation or chemicals. While many mutations are harmful or neutral, some can be beneficial, providing a selective advantage in a particular environment.
Mutations are the ultimate source of new genetic variation, providing the raw material for evolutionary change. Without mutations, natural selection would have nothing to act upon.
Adaptation and the Inheritance of Advantageous Traits
Adaptation is the process by which populations become better suited to their environment through natural selection. This involves the inheritance of advantageous traits that increase an organism's survival and reproductive success.
For example, the peppered moth's color evolved from light to dark during the industrial revolution in England, due to increased pollution darkening the tree bark. Moths with darker wings were better camouflaged and less likely to be eaten by birds, leading to an increase in the frequency of the dark-winged allele in the population.
Key Concepts: Phenotype, Genotype, Heritability, and Epigenetics
The intricate relationship between genes and physical characteristics necessitates a firm understanding of several key concepts. These concepts provide the framework for dissecting the complex interplay between our genetic inheritance, environmental influences, and the non-DNA sequence-based mechanisms that shape our traits.
This section will clarify the concepts of phenotype, genotype, heritability, and epigenetics. It aims to highlight the dynamic interactions that ultimately determine the physical attributes that define us.
Phenotype vs. Genotype: Understanding the Dichotomy
The terms phenotype and genotype are fundamental to understanding the relationship between genes and observable traits. The genotype refers to the genetic makeup of an organism. It describes the specific alleles (versions of a gene) that an individual possesses. The phenotype, on the other hand, encompasses the observable characteristics or traits of an organism.
These traits can range from easily visible attributes like eye color and height to more complex characteristics such as disease susceptibility and behavioral tendencies.
Examples of Phenotypic Traits
Examples of physical traits are abundant and diverse.
-
Eye color, determined by the amount and type of pigment in the iris.
-
Height, influenced by multiple genes and environmental factors like nutrition.
-
Susceptibility to certain diseases, such as type 2 diabetes, which has a genetic component but is also significantly affected by lifestyle.
The Interplay Between Genes and Environment
The link between a specific gene and its phenotypic expression isn't always direct. Some genes have a one-to-one correspondence. However, most traits are influenced by multiple genes, a phenomenon known as polygenic inheritance. Moreover, environmental factors often play a crucial role in modifying how a genotype is expressed as a phenotype.
For instance, an individual might have a genetic predisposition for tallness (genotype). However, inadequate nutrition during childhood (environment) could prevent them from reaching their full potential height (phenotype).
Gene-Environment Interactions
Gene-environment interactions refer to the ways in which the effects of genes and environment on a phenotype are interdependent. A classic example is the interaction between the MAOA gene and childhood maltreatment in predicting antisocial behavior. Individuals with a low-activity variant of the MAOA gene are more likely to exhibit antisocial behavior if they experienced abuse as children.
However, those with the same genotype who did not experience abuse are not more likely to show this behavior. This illustrates how a genotype can influence a phenotype differently depending on the environment.
Heritability: Quantifying Genetic Influence
Heritability is a statistical measure that estimates the proportion of phenotypic variation in a population that can be attributed to genetic factors. It is important to note that heritability is a population-level measure and does not indicate the degree to which a trait is genetically determined in any particular individual.
Estimating Heritability
Heritability is often estimated using twin studies. Identical twins share nearly 100% of their genes, while fraternal twins share approximately 50%.
By comparing the similarity of traits between identical and fraternal twins, researchers can estimate the relative contributions of genetic and environmental factors to the variation of those traits.
Another method involves adoption studies, which compare adopted individuals to their biological and adoptive parents.
Implications of Heritability Estimates
Heritability estimates can provide insights into the potential for traits to respond to selection pressures, either natural or artificial. If a trait has high heritability, it suggests that selection can lead to rapid changes in that trait across generations.
It's vital to avoid misinterpreting high heritability as evidence that environmental factors are unimportant. Even highly heritable traits are still influenced by the environment. Heritability simply quantifies the relative contribution of genetic factors to variation within a specific population and environment.
Epigenetics: Beyond the DNA Sequence
Epigenetics refers to changes in gene expression that do not involve alterations to the underlying DNA sequence. These changes can be inherited across cell divisions, and in some cases, across generations. Epigenetic mechanisms provide a way for the environment to influence gene activity without changing the genetic code itself.
Mechanisms of Epigenetic Inheritance
Several epigenetic mechanisms have been identified. The most well-studied include:
-
DNA methylation: The addition of a methyl group to a DNA base, typically cytosine. Methylation often leads to reduced gene expression.
-
Histone modification: Chemical modifications to histone proteins around which DNA is wrapped. These modifications can affect the accessibility of DNA to transcription factors, thereby influencing gene expression.
-
Non-coding RNAs: RNA molecules that are not translated into protein but can regulate gene expression.
Epigenetics and Phenotypic Traits
Epigenetic modifications can play a significant role in shaping physical traits and influencing disease susceptibility. For example, studies have shown that early-life experiences, such as maternal care, can alter DNA methylation patterns in offspring, affecting their stress response and behavior later in life.
Epigenetic changes have also been implicated in the development of various diseases, including cancer, cardiovascular disease, and neurological disorders. Understanding epigenetic mechanisms is crucial for developing targeted therapies that can reverse or prevent disease-related epigenetic modifications.
Disciplines in Action: Genomics and Anthropometry
The quest to decipher the genetic underpinnings of physical traits has been significantly advanced by two powerful disciplines: genomics and anthropometry. These fields offer complementary approaches, with genomics providing a holistic view of the genome and anthropometry offering precise measurements of the human form.
By integrating the insights from both disciplines, researchers are gaining a deeper understanding of the complex interplay between genes and physical characteristics.
Genomics: Unveiling the Blueprint
Genomics represents a paradigm shift in biological research. It enables scientists to study the entire genome of an organism.
This capability contrasts with traditional genetic approaches. These often focus on individual genes or small sets of genes.
Whole-Genome Analysis and its Applications
Whole-genome analysis involves sequencing and analyzing the complete DNA sequence of an individual or organism. This comprehensive approach allows researchers to identify genetic variations, structural anomalies, and gene expression patterns that may be associated with specific physical traits.
Genome-wide association studies (GWAS), for example, compare the genomes of individuals with and without a particular trait to identify genetic variants that are more common in those with the trait. These variants are then flagged as potential genetic contributors.
Beyond identifying specific genes, whole-genome analysis can reveal broader patterns of genetic influence. This can include gene-gene interactions and the cumulative effects of multiple genes on a single trait.
Identifying Genes Associated with Physical Traits
Genomics plays a crucial role in identifying genes that influence physical traits. The study of genes such as MC1R exemplifies this. MC1R is linked to skin and hair pigmentation.
GWAS have successfully pinpointed numerous genes associated with a wide range of physical traits, from height and eye color to susceptibility to certain diseases. This is helping to connect our genetic makeup to the observable traits that define us.
Comparative Genomics and Trait Differences
Comparative genomics extends the power of genomics by comparing the genomes of different species. This helps scientists understand the genetic basis of trait differences between species.
By identifying genes that are conserved across species (genes that have remained relatively unchanged throughout evolution) and genes that have diverged, researchers can pinpoint the genetic changes that have contributed to the evolution of specific traits.
Conserved and Divergent Regions of the Genome
The comparison of genomes reveals regions that are highly conserved. It also shows regions that exhibit significant divergence.
Conserved regions often contain genes that are essential for basic cellular functions and development. Divergent regions, on the other hand, may contain genes that are responsible for species-specific traits or adaptations.
Identifying these regions provides valuable insights into the genetic mechanisms that underlie both the similarities and differences between species.
Anthropometry: Quantifying the Human Form
Anthropometry is the scientific study of the measurement of the human body. It involves a range of techniques for assessing body size, shape, proportion, composition, and function.
Anthropometry provides quantitative data that can be used to study human variation, growth, and development.
Techniques in Anthropometry
Anthropometric techniques include measurements of height, weight, limb lengths, circumferences, skinfold thickness, and body composition.
Advanced imaging techniques, such as magnetic resonance imaging (MRI) and dual-energy X-ray absorptiometry (DEXA), are also used to assess body composition and internal organ size.
These measurements provide a detailed profile of an individual's physical characteristics. They also offer insights into their nutritional status, health, and developmental trajectory.
Studying Human Variation and Growth
Anthropometry is a valuable tool for studying human variation across populations and over time. By collecting anthropometric data from diverse groups, researchers can identify patterns of variation in body size, shape, and composition.
These patterns can be related to genetic factors, environmental influences, and lifestyle factors.
Anthropometric data is also used to track growth and development in children and adolescents, allowing researchers to identify factors that promote healthy growth and prevent developmental problems.
Anthropometric Measures, Genetic Factors, and Health
Anthropometric measures are closely linked to genetic factors and health outcomes. For example, height and body mass index (BMI) have been shown to be heritable traits. This means that genetic factors play a significant role in determining an individual's height and weight.
Anthropometric measures are also associated with a range of health outcomes. This includes cardiovascular disease, diabetes, and cancer.
By studying the relationship between anthropometric measures, genetic factors, and health outcomes, researchers can gain insights into the genetic and environmental factors that contribute to disease risk.
Studying the Genetic Basis of Body Size and Shape
Anthropometry provides the quantitative data needed to study the genetic basis of body size and shape. By combining anthropometric measurements with genomic data, researchers can identify genes that influence these traits.
For example, GWAS have identified numerous genes associated with height, BMI, and waist-to-hip ratio. These genes provide potential targets for interventions aimed at promoting healthy body size and shape.
Integrating Genomics and Anthropometry
The integration of genomics and anthropometry offers a powerful approach for understanding the complex interplay between genes and physical traits.
By combining genomic data with detailed measurements of the human form, researchers can gain a more complete picture of the genetic and environmental factors that shape our physical characteristics and influence our health. This synergistic approach holds great promise for advancing our understanding of human biology and for developing personalized strategies for preventing and treating disease.
Trait Examples: Unpacking the Genetics of Appearance
Physical traits, those observable characteristics that define our individuality, are a testament to the intricate interplay between our genes and the environment. Understanding the genetic underpinnings of these traits requires a nuanced approach, one that acknowledges both the discrete categories some traits fall into and the continuous spectrum of variation seen in others. Examining specific examples, such as eye color, height, hair characteristics, and skin pigmentation, reveals the complexities of genetic influence.
Categorizing Traits: Qualitative vs. Quantitative
One fundamental way to approach the study of physical traits is to categorize them as either qualitative or quantitative.
Qualitative traits are those that fall into distinct categories, with no intermediate values.
A classic example is blood type in the ABO system: an individual can have type A, B, AB, or O, but nothing in between.
These traits are often governed by one or a few major genes with strong effects.
Quantitative traits, on the other hand, exhibit continuous variation within a population.
Height is a prime example; individuals can fall anywhere along a spectrum of heights, from very short to very tall.
Quantitative traits are typically influenced by many genes, each with a small effect, as well as environmental factors.
The distinction between qualitative and quantitative traits provides a useful framework for understanding the genetic architecture of physical characteristics.
Specific Trait Examples: Decoding the Genetics of Appearance
Delving into the genetics of specific physical traits offers a glimpse into the mechanisms that shape our appearance.
Eye Color: A Tale of Multiple Genes
The determination of eye color, once thought to be a simple Mendelian trait, is now recognized as a more complex phenomenon involving multiple genes.
While the OCA2 gene plays a major role, influencing the amount of melanin in the iris, other genes also contribute to the final shade of blue, green, hazel, or brown.
Variations in these genes can lead to a wide spectrum of eye colors, showcasing the polygenic nature of this trait.
Height: A Complex Polygenic Trait
Height is a quintessential example of a quantitative trait, influenced by hundreds, if not thousands, of genetic variants scattered throughout the genome.
These variants, each with a subtle effect, collectively contribute to an individual's stature.
Environmental factors, such as nutrition during childhood, also play a significant role in determining final height.
Genome-wide association studies (GWAS) have identified many of these height-associated genes, providing insights into the biological pathways that regulate skeletal growth.
Hair Color and Texture: A Diverse Spectrum of Genes
Hair color and texture are determined by a complex interplay of genetic factors.
Genes such as MC1R play a crucial role in determining hair color. Variations in this gene can lead to a range of colors, from red and blonde to brown and black.
Other genes influence hair texture, determining whether it is straight, wavy, curly, or coiled.
The combination of these genetic factors creates the remarkable diversity of hair characteristics observed in human populations.
Skin Color: Adaptation and Genetic Variation
Skin color is a highly variable trait that reflects adaptation to different levels of ultraviolet (UV) radiation.
Melanin, a pigment produced by melanocytes, protects the skin from UV damage.
Genes that regulate melanin production, such as SLC24A5, show variation across populations, leading to differences in skin pigmentation.
Individuals from regions with high UV radiation tend to have darker skin, while those from regions with low UV radiation tend to have lighter skin. This reflects the evolutionary pressures that have shaped skin color over time.
Ethical Considerations: Navigating the Complexities of Genetic Information
The rapid advancement of genetic technologies brings immense potential for understanding and improving human health. However, with this progress comes the critical responsibility to address the ethical implications that arise from the use of genetic information. The potential for misuse, particularly in the realms of genetic discrimination and privacy violations, necessitates careful consideration and proactive measures to ensure equitable and ethical application of these powerful tools. Ensuring equality and protecting sensitive data are paramount.
Genetic Discrimination: Ensuring Equality and Fairness
The possibility of genetic discrimination represents a significant ethical challenge. This occurs when genetic information is used unfairly to disadvantage individuals or groups. Such discrimination can manifest in various forms, creating barriers to opportunities and perpetuating societal inequalities.
The Potential for Unfair Use
Genetic information, revealing predispositions to certain diseases or conditions, can be misinterpreted or misused. This could lead to prejudiced decisions in areas like employment, insurance, and even social contexts. For instance, an employer might hesitate to hire someone with a genetic predisposition to a late-onset disease, fearing future healthcare costs or reduced productivity. Similarly, insurance companies could deny coverage or charge exorbitant premiums based on genetic risk factors, irrespective of an individual's current health status. The fear of such misuse can create anxiety and discourage individuals from undergoing genetic testing, even when it could be beneficial for their health.
Impact on Employment, Insurance, and Social Interactions
The consequences of genetic discrimination can be far-reaching and devastating. In the workplace, individuals might face limited career advancement opportunities or outright denial of employment based on their genetic profile. In the insurance sector, higher premiums or denial of coverage can create significant financial burdens, especially for those already facing health challenges. Socially, genetic information could lead to stigmatization or exclusion, particularly if individuals are perceived as being at higher risk for certain conditions. This can erode trust and social cohesion, further marginalizing vulnerable populations. Protecting individuals from these potential harms requires a comprehensive approach that combines legal safeguards, ethical guidelines, and public education.
Measures to Prevent Genetic Discrimination
Several measures can be implemented to prevent genetic discrimination and promote equality. Legislative protections are crucial, such as the Genetic Information Nondiscrimination Act (GINA) in the United States, which prohibits genetic discrimination in employment and health insurance. Strong enforcement mechanisms are needed to ensure compliance and hold perpetrators accountable. Ethical guidelines for the use of genetic information are also essential. These guidelines should emphasize the principles of fairness, non-discrimination, and respect for individual autonomy. Public education and awareness campaigns can help dispel misconceptions about genetics and promote a more inclusive and understanding society. Furthermore, promoting diversity and inclusion in research and clinical settings can help address potential biases and ensure that genetic technologies benefit all populations equitably.
Privacy: Protecting Sensitive Data and Confidentiality
The protection of genetic privacy is another critical ethical imperative. Genetic information is deeply personal and sensitive, and its unauthorized access or disclosure can have profound consequences for individuals and their families. Safeguarding this information requires robust privacy protections and ethical guidelines.
The Importance of Protecting Genetic Information
Genetic data reveals not only an individual's predispositions but also information about their ancestry and potential health risks for their relatives. Unauthorized access to this data could be used for malicious purposes, such as identity theft, fraud, or discrimination. Even seemingly innocuous uses, like sharing genetic data with third-party companies without informed consent, can raise serious privacy concerns. Individuals have a fundamental right to control their genetic information and decide how it is used. Protecting this right is essential for maintaining trust in the healthcare system and promoting responsible innovation in the field of genetics.
Risks of Unauthorized Access to Genetic Data
The risks of unauthorized access to genetic data are multifaceted and potentially severe. Data breaches at genetic testing companies or healthcare institutions can expose sensitive information to hackers and malicious actors. Law enforcement agencies might seek access to genetic databases for investigative purposes, raising concerns about surveillance and potential abuses of power. Furthermore, the increasing use of direct-to-consumer genetic testing services raises questions about the privacy policies and data security practices of these companies. Individuals may not fully understand how their data is being used or shared, and they may not have adequate control over its dissemination. Addressing these risks requires a multi-pronged approach that includes robust cybersecurity measures, transparent data governance policies, and strong legal protections for genetic privacy.
Regulations and Guidelines for Safeguarding Genetic Privacy
Several regulations and guidelines are in place to safeguard genetic privacy and protect individuals' rights. The Health Insurance Portability and Accountability Act (HIPAA) in the United States provides some protection for genetic information held by healthcare providers and health plans. However, HIPAA does not cover all types of genetic data, such as information held by direct-to-consumer testing companies. The European Union's General Data Protection Regulation (GDPR) sets a higher standard for data protection, including genetic data. GDPR requires explicit consent for the processing of genetic information and gives individuals greater control over their data. Ethical guidelines and best practices for genetic research and clinical practice also play a crucial role in protecting genetic privacy. These guidelines emphasize the importance of informed consent, data security, and confidentiality. Ongoing efforts are needed to strengthen and update these regulations and guidelines to keep pace with the rapid evolution of genetic technologies and ensure that genetic privacy is adequately protected in the digital age.
Video: Physical Traits: Decoding Characteristics
FAQs: Physical Traits: Decoding Characteristics
What does it mean to "decode" physical characteristics?
Decoding physical characteristics refers to understanding the relationship between observed physical traits and the underlying genetic and environmental factors that influence them. It involves analyzing how the physical characteristics of a trait, like eye color or height, are determined by genes and shaped by environment.
How are physical traits passed down?
Physical traits are passed down from parents to offspring through genes. Genes contain the instructions for developing the physical characteristics of a trait, such as hair texture or skin pigmentation. Offspring inherit a combination of genes from each parent, resulting in variations in inherited physical traits.
Can environmental factors affect physical traits?
Yes, environmental factors can significantly influence physical traits. While genes provide the blueprint, environmental factors such as nutrition, climate, and lifestyle can alter how the physical characteristics of a trait are expressed. For instance, diet influences height, and sun exposure affects skin tone.
What can studying physical traits tell us?
Studying physical traits can provide insights into ancestry, genetic predispositions to certain diseases, and adaptations to different environments. Understanding the physical characteristics of a trait also helps us track evolutionary changes and understand the complexities of human variation.
So, next time you're people-watching, remember there's likely more to that furrowed brow or confident gait than meets the eye. While not a foolproof science, understanding the potential links between personality and those subtle physical characteristics of a trait can offer intriguing insights into the people around us – and maybe even ourselves!