Peroxide Ion: What It Is & Why You Should Care 🧪
Understanding the behavior of peroxide ion is essential in various scientific disciplines. Hydrogen peroxide, a common chemical compound, is directly related to the peroxide ion's stability. Research institutions such as the National Institutes of Health (NIH) often investigate the effects of this ion in biological systems. Furthermore, analytical techniques like spectrophotometry are frequently used to quantify the presence of peroxide ions in solutions. Gaining deeper knowledge will allow readers to understand why the peroxide ion plays such a huge role in many industrial and research practices.
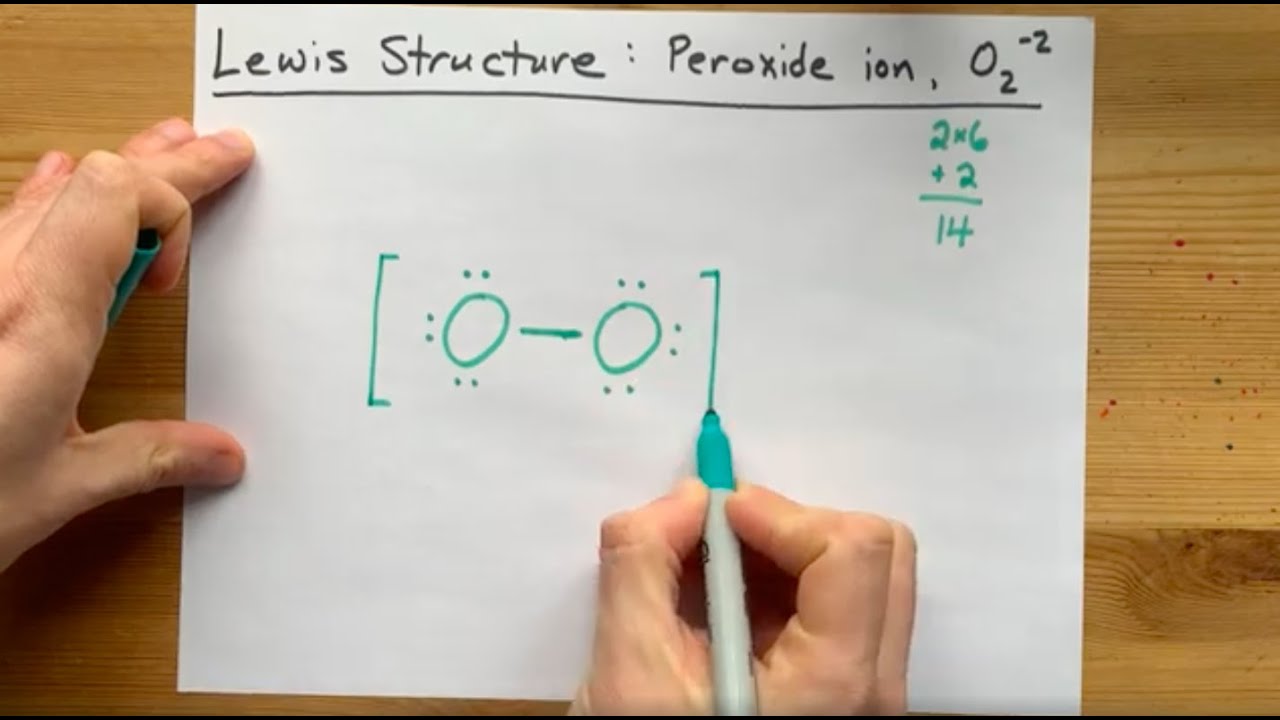
Image taken from the YouTube channel chemistNATE , from the video titled Lewis Structure of the Peroxide Ion, O2(-2) .
Peroxides: the name itself might conjure images of hair bleaching or antiseptic solutions. But beneath these common associations lies a fascinating world of chemistry, impacting fields from medicine to environmental science. Prepare to have your perceptions challenged as we delve into the intriguing nature of the peroxide ion (O22-).
The Peroxide Ion: A Definition and its Significance
At its core, the peroxide ion is a diatomic molecular anion composed of two oxygen atoms covalently bonded, carrying a total charge of -2. This seemingly simple structure belies its profound significance in chemistry. The peroxide ion acts as a powerful oxidizing agent, readily accepting electrons from other substances. This property is the key to its diverse applications.
From bleaching agents that brighten our clothes to disinfectants that protect us from harmful pathogens, the peroxide ion plays a crucial role in countless chemical processes. Its presence is also central to many biological systems, where it participates in enzyme catalysis and cellular signaling. Understanding its behavior is crucial to appreciating its functionality.
Charting Our Course: A Look Ahead
This article aims to provide a comprehensive exploration of the peroxide ion, moving beyond surface-level observations to uncover its underlying principles and real-world implications. We will examine its chemical structure, formation, and properties.
We will explore the quintessential example of hydrogen peroxide (H2O2), discussing its synthesis and stability. Then we will move on to consider its versatile applications as an oxidizing agent. We will also differentiate the peroxide ion from similar oxygen-containing species such as the superoxide ion.
Finally, we will address critical safety considerations for handling peroxides and discuss the factors influencing their decomposition. By the end of this exploration, you will gain a deeper appreciation for the power and complexity of the peroxide ion.
Peroxides in Everyday Life: More Than Meets the Eye
Perhaps the most striking aspect of peroxides is their ubiquitous presence in our daily routines. Hydrogen peroxide, readily available at your local pharmacy, serves as a disinfectant for minor cuts and scrapes. Benzoyl peroxide, another common peroxide, is a staple ingredient in acne treatments.
In the realm of household cleaning, peroxides are frequently employed as bleaching agents in laundry detergents and stain removers. Even in the food industry, peroxides play a role in sanitizing packaging materials and processing certain food products.
These examples merely scratch the surface of the peroxide ion's pervasive influence, highlighting its importance in both industrial applications and consumer products. By unraveling the mysteries of this remarkable chemical entity, we can better understand the world around us.
Delving into the Peroxide Ion: Structure, Formation, and Properties
Having established the peroxide ion’s significance, it's time to explore the underlying chemistry that governs its behavior. Understanding its structure, formation, and properties is crucial to grasping its versatility and diverse applications. This section unpacks the peroxide ion's intricacies, revealing its unique characteristics and how they contribute to its chemical reactivity.
The Peroxide Ion's Structure: A Covalent Bond with a Negative Charge
The peroxide ion (O22-) is a diatomic molecule, meaning it consists of two oxygen atoms. These oxygen atoms are joined by a covalent bond, sharing electrons to achieve stability.
Each oxygen atom carries a partial negative charge, resulting in a total charge of -2 for the entire ion.
Diagrams and illustrations often depict this structure with a single line representing the covalent bond between the two oxygen atoms, along with the overall negative charge indicated as a superscript. This visual representation helps clarify the ion's composition and charge distribution.
From Elemental Oxygen to the Peroxide Ion: A Tale of Electron Gain
The journey from elemental oxygen (O2) to the peroxide ion (O22-) involves a significant change in electron count. Elemental oxygen is a neutral molecule.
To transform into the peroxide ion, the oxygen molecule gains two electrons. This electron acquisition fundamentally alters its properties and reactivity.
The addition of these electrons creates the negative charge and strengthens the oxidizing power characteristic of peroxides.
Oxidation-Reduction Reactions: The Driving Force Behind Peroxide Formation
Oxidation-reduction (redox) reactions are fundamental to understanding peroxide formation. Oxidation involves the loss of electrons, while reduction involves the gain of electrons.
In the context of peroxide formation, a substance must donate electrons (be oxidized) to elemental oxygen, causing it to be reduced into the peroxide ion.
For example, alkali metals readily react with oxygen to form peroxides, as the metal atoms lose electrons (are oxidized) and oxygen gains electrons (is reduced). This electron transfer is the key to creating the peroxide ion.
Peroxide vs. Superoxide: Distinguishing Oxygen-Containing Ions
While the peroxide ion features two oxygen atoms bonded together with a -2 charge, other oxygen-containing ions exist, each with distinct structures and reactivity. A prime example is the superoxide ion (O2-).
The superoxide ion carries a single negative charge and contains one unpaired electron. This difference in charge and electron configuration significantly impacts its chemical behavior.
Superoxide is often considered a free radical, making it highly reactive and playing a role in oxidative stress within biological systems. Peroxide, while also reactive, tends to participate in different types of reactions compared to superoxide.
Unveiling the Chemical Properties: Stability, Reactivity, and Potential Hazards
The peroxide ion exhibits several key chemical properties that determine its applications and associated risks. Stability is a crucial factor; peroxides are generally unstable compounds, prone to decomposition.
Factors such as temperature, light exposure, and the presence of catalysts can accelerate this decomposition process.
Reactivity stems from the peroxide ion's strong oxidizing power. This ability to readily accept electrons makes it effective as a bleaching agent and disinfectant.
However, this reactivity also poses potential hazards. Concentrated peroxides can be corrosive and may react violently with certain organic materials, leading to fire or explosion risks. Therefore, careful handling and storage are essential when working with peroxides.
Having established the peroxide ion’s significance, it's time to explore the underlying chemistry that governs its behavior. Understanding its structure, formation, and properties is crucial to grasping its versatility and diverse applications. This section unpacks the peroxide ion's intricacies, revealing its unique characteristics and how they contribute to its chemical reactivity.
Hydrogen Peroxide (H2O2): The Quintessential Peroxide
Among the diverse family of peroxides, hydrogen peroxide (H2O2) reigns supreme as the most ubiquitous and extensively utilized. Its accessibility, coupled with its potent chemical properties, has cemented its position across a wide spectrum of industries and everyday applications.
This section will delve into the synthesis, stability, and inherent characteristics of hydrogen peroxide. A key aspect is understanding how these properties are intrinsically linked to the presence and behavior of the peroxide ion itself.
The Pervasive Presence of H2O2
Hydrogen peroxide is not merely a laboratory curiosity. It's a workhorse chemical woven into the fabric of modern life. From the familiar brown bottle in your medicine cabinet to large-scale industrial processes, H2O2 plays a crucial role.
Its versatility stems from its relatively simple molecular structure and its ability to readily decompose, releasing oxygen and water. This decomposition underpins many of its applications, particularly those related to oxidation and disinfection.
Synthesizing Hydrogen Peroxide: From Industry to Lab
The production of hydrogen peroxide occurs through various methods, tailored to the scale and purity required. Industrial processes prioritize efficiency and cost-effectiveness. While laboratory techniques focus on producing small quantities of high-purity H2O2.
Industrial Production Methods
The anthraquinone process dominates industrial production. This cyclic process involves the oxidation of an anthrahydroquinone derivative followed by extraction of H2O2 with water. The anthraquinone is then regenerated, creating a continuous loop.
This method offers a high yield and is economically viable for large-scale production, meeting the demands of various industries.
Laboratory Synthesis
In laboratory settings, hydrogen peroxide can be synthesized through the reaction of barium peroxide with sulfuric acid.
Alternatively, electrolysis of sulfuric acid solutions can also yield H2O2. These methods, while less efficient than the anthraquinone process, are suitable for producing small, controlled quantities for research and specific applications.
The Delicate Stability of Hydrogen Peroxide
Despite its widespread use, hydrogen peroxide is inherently unstable. It readily decomposes into water and oxygen, especially under certain conditions. Understanding and controlling these factors is crucial for maintaining its effectiveness and safety.
Factors Influencing Stability
Temperature plays a significant role. Elevated temperatures accelerate the decomposition process.
Similarly, pH affects stability. H2O2 is most stable in slightly acidic conditions. Alkaline conditions promote decomposition.
The presence of impurities, particularly transition metal ions like iron or copper, can act as catalysts, speeding up decomposition. This is why stabilizers are often added to commercial H2O2 solutions.
Strategies for Stabilization
To prolong the shelf life and maintain the potency of hydrogen peroxide, stabilizers are added. These stabilizers typically chelate metal ions, preventing them from catalyzing decomposition.
Proper storage in cool, dark conditions also minimizes decomposition. Containers should be vented to prevent pressure buildup from released oxygen.
The Peroxide Ion's Influence on H2O2 Properties
The unique properties of hydrogen peroxide, particularly its oxidizing power and bleaching capabilities, are directly attributable to the presence of the peroxide ion within its molecular structure.
The O-O bond in H2O2 is relatively weak. This bond is easily broken, leading to the formation of highly reactive oxygen species. These species readily accept electrons from other molecules, thus acting as potent oxidizing agents.
This oxidizing power is the foundation for H2O2's use in bleaching applications. It breaks down colored compounds by disrupting their chemical bonds, rendering them colorless.
Furthermore, the oxidizing properties of hydrogen peroxide enable its use as a disinfectant. It damages the cellular components of microorganisms, leading to their inactivation.
In essence, the peroxide ion is the engine driving the reactivity and utility of hydrogen peroxide, making it an indispensable chemical in countless applications.
Peroxides as Powerful Oxidizing Agents: Applications in Bleaching and Disinfection
Having established the peroxide ion’s significance, it's time to explore the underlying chemistry that governs its behavior. Understanding its structure, formation, and properties is crucial to grasping its versatility and diverse applications.
This section unpacks the peroxide ion's intricacies, revealing its unique characteristics and how they contribute to its chemical reactivity.
The Oxidizing Power of Peroxides: A Deep Dive
Peroxides owe their utility in bleaching and disinfection to their potent oxidizing capabilities. But what exactly makes them such effective oxidizing agents? The key lies in the peroxide ion's electronic structure and its eagerness to accept electrons from other molecules.
The peroxide ion (O22-) readily undergoes reduction, meaning it gains electrons. In doing so, it forces other molecules to undergo oxidation, losing electrons. This electron transfer process is the foundation of its oxidizing action.
The Chemistry of Oxidation: Electron Transfer Explained
At the core of the peroxide ion's oxidizing ability is its unique arrangement of oxygen atoms bound together. This configuration makes the peroxide ion inherently unstable and eager to achieve a more stable state by acquiring electrons.
When a peroxide encounters a substance that can donate electrons, a redox (reduction-oxidation) reaction occurs. The peroxide accepts electrons (reduction), while the other substance loses electrons (oxidation).
This electron transfer destabilizes the target molecule, leading to its breakdown or alteration.
Bleaching: How Peroxides Eradicate Color
One of the most recognizable applications of peroxides is bleaching. Peroxides are employed in various bleaching processes, from whitening clothes to lightening hair. Their effectiveness stems from their ability to disrupt the chemical bonds within colored compounds.
Colored substances owe their hues to specific molecules called chromophores, which absorb certain wavelengths of light. Peroxides, acting as oxidizing agents, attack these chromophores.
By disrupting the chromophores' structure through oxidation, peroxides alter their light-absorbing properties. This alteration results in the fading or elimination of the color, achieving the desired bleaching effect.
Different types of peroxides are used depending on the application. Hydrogen peroxide (H2O2) is commonly used in laundry and hair bleaching. Other peroxides, like sodium percarbonate, are found in laundry detergents and stain removers.
Disinfection: Peroxides as Microbial Warriors
Beyond bleaching, peroxides play a critical role in disinfection. Their oxidizing power makes them effective at eliminating a wide range of microorganisms, including bacteria, viruses, and fungi.
Peroxides achieve disinfection by attacking essential components of microbial cells. This includes disrupting cell membranes, oxidizing proteins, and damaging DNA or RNA.
The oxidation of these vital cellular components leads to the inactivation or death of the microorganism.
Hydrogen peroxide is a common disinfectant used in household cleaning products, wound care solutions, and sterilization processes. Other peroxides, such as peracetic acid, are used in healthcare settings and the food industry for sterilizing equipment and surfaces.
Broad-Spectrum Activity and Environmental Considerations
One significant advantage of peroxides as disinfectants is their broad-spectrum activity. They can effectively target a wide variety of microorganisms, making them versatile for various disinfection needs.
Moreover, peroxides like hydrogen peroxide decompose into water and oxygen, minimizing their environmental impact compared to other harsh chemicals.
This makes them a more sustainable option for disinfection in certain applications.
Peroxides owe their utility in bleaching and disinfection to their potent oxidizing capabilities. But what exactly makes them such effective oxidizing agents? The key lies in the peroxide ion's electronic structure and its eagerness to accept electrons from other molecules.
Now, let’s journey beyond these well-known applications to uncover the extensive versatility of peroxides in various sectors.
Diverse Applications of Peroxides: From Medicine to Wastewater Treatment
Peroxides, beyond their common uses, demonstrate remarkable adaptability across a spectrum of industries. Their unique chemical properties make them invaluable in applications ranging from sterilizing medical equipment to purifying wastewater and even processing food.
Let's explore the multifaceted roles peroxides play in these critical sectors.
Peroxides in the Medical Field: Sterilization and Wound Care
In the medical field, sterility is paramount, and peroxides play a crucial role in achieving this. Hydrogen peroxide (H2O2), in particular, is widely used as a disinfectant and antiseptic.
Its oxidizing properties allow it to effectively eliminate bacteria, viruses, and fungi from surfaces and medical instruments.
Wound Disinfection and Antiseptic Applications
When applied to wounds, hydrogen peroxide helps to clean the area by killing bacteria and removing dead tissue. This promotes healing and reduces the risk of infection.
However, it's important to note that while effective, hydrogen peroxide can also damage healthy cells, so its use is generally limited to initial cleaning and should not be used for prolonged or repeated applications.
Sterilization of Medical Equipment
Beyond direct wound care, peroxides are essential for sterilizing medical equipment.
Vaporized hydrogen peroxide, for example, is used in sterilization systems to ensure that surgical instruments and other sensitive equipment are free from microbial contamination.
This method is particularly effective because the vapor can reach areas that are difficult to access with traditional liquid disinfectants.
Textile Industry: Bleaching and Fabric Whitening
The textile industry relies heavily on peroxides, particularly hydrogen peroxide, for bleaching fabrics. The goal is to remove natural colors and impurities from raw materials like cotton and wool.
This prepares the fabric for dyeing or printing, ensuring vibrant and consistent color results.
Effective Stain Removal
Peroxides are highly effective at removing stains from fabrics, thanks to their oxidizing action.
They break down the chemical bonds of colored compounds, rendering them colorless.
This makes peroxides indispensable in the production of clean, white textiles.
Environmentally Friendly Bleaching
Compared to some traditional bleaching agents like chlorine, peroxides are often considered a more environmentally friendly option.
Hydrogen peroxide, for instance, decomposes into water and oxygen, minimizing the release of harmful byproducts into the environment.
Wastewater Treatment: Removing Pollutants and Contaminants
Peroxides play a vital role in wastewater treatment by helping to remove pollutants and contaminants.
Advanced Oxidation Processes (AOPs) using hydrogen peroxide are employed to degrade organic compounds that are difficult to remove through conventional treatment methods.
Degrading Persistent Organic Pollutants
Many industrial and agricultural processes release persistent organic pollutants (POPs) into wastewater.
These compounds, resistant to natural degradation, pose a significant threat to aquatic ecosystems and human health. Peroxides, in combination with UV light or ozone, can effectively break down these pollutants into less harmful substances.
Disinfection and Odor Control
In addition to removing pollutants, peroxides are also used for disinfection and odor control in wastewater treatment plants.
They can eliminate harmful bacteria and viruses, ensuring the safety of treated water before it is discharged back into the environment.
Furthermore, peroxides can oxidize odor-causing compounds, improving air quality around treatment facilities.
Food Industry: Sanitation and Food Processing
The food industry utilizes peroxides for a variety of applications, primarily related to sanitation and food processing.
Hydrogen peroxide is used to sanitize equipment and surfaces, helping to prevent the spread of foodborne illnesses.
It is also employed in certain food processing applications, such as bleaching flour and modifying starch.
Equipment Sanitation and Safety
Maintaining a clean and sanitary environment is crucial in food production.
Peroxides are used to disinfect processing equipment, storage containers, and other surfaces that come into contact with food.
This helps to reduce the risk of contamination and ensure the safety of food products.
Bleaching and Modifying Food Products
In some cases, peroxides are used to bleach or modify certain food products. For example, hydrogen peroxide can be used to whiten flour, improving its appearance and baking qualities.
It is also used to modify starch, altering its properties for specific food applications.
However, the use of peroxides in food processing is carefully regulated to ensure that residual levels are safe for consumption.
Peroxides owe their utility in bleaching and disinfection to their potent oxidizing capabilities. But what exactly makes them such effective oxidizing agents? The key lies in the peroxide ion's electronic structure and its eagerness to accept electrons from other molecules.
Now, let’s journey beyond these well-known applications to uncover the extensive versatility of peroxides in various sectors.
Peroxide vs. Superoxide: Understanding the Differences
While the term "peroxide" is relatively familiar, understanding its nuances compared to other oxygen species is crucial for a comprehensive grasp of its chemical behavior and biological relevance. One such species that often gets conflated with the peroxide ion is the superoxide ion.
Let's delve into the structural and reactivity distinctions between these two important oxygen-containing ions.
Structural Distinctions: A Matter of Electrons
The key difference between peroxide and superoxide lies in their electronic structure and, consequently, their overall charge and reactivity. The peroxide ion (O22-) features two oxygen atoms covalently bonded to each other with an overall charge of -2. This means each oxygen atom effectively has an oxidation state of -1.
In contrast, the superoxide ion (O2-) also comprises two covalently bonded oxygen atoms, but it carries a single negative charge. This gives each oxygen atom an average oxidation state of -1/2. This seemingly small difference in charge has profound implications for their chemical behavior.
Reactivity Differences: Oxidizing vs. Reducing
The differing electronic structures directly influence the reactivity of peroxide and superoxide. Peroxides are primarily known as strong oxidizing agents. They readily accept electrons from other molecules, causing their oxidation. This oxidizing power is harnessed in applications like bleaching and disinfection.
Superoxide, on the other hand, exhibits a more complex reactivity profile. It can act as both an oxidizing and a reducing agent, depending on the specific reaction conditions and the other reactants involved. This dual nature stems from its unpaired electron, making it a radical species.
Distinct Roles in Biological Systems
Both peroxide and superoxide play critical, yet distinct, roles within biological systems. Superoxide is a key player in the immune response. It is produced by phagocytes (immune cells that engulf and destroy pathogens) to kill invading bacteria and other microorganisms. However, uncontrolled superoxide production can also lead to oxidative stress and cellular damage.
Peroxides, particularly hydrogen peroxide (H2O2), also participate in cellular signaling and regulation. While it can be toxic at high concentrations, H2O2 acts as a signaling molecule at lower levels, influencing various cellular processes, including gene expression and cell growth. Enzymes like catalase and glutathione peroxidase tightly control peroxide levels to prevent oxidative damage.
In summary, while both peroxide and superoxide are oxygen-containing ions with important roles, their structural and electronic differences lead to distinct chemical reactivities and biological functions. Understanding these distinctions is essential for appreciating the diverse chemistry of oxygen and its impact on various scientific disciplines.
Safety First: Handling Peroxides with Care
Peroxides, for all their utility in diverse applications, demand respect. Their inherent reactivity, the very characteristic that makes them so useful, also poses potential hazards. Understanding and adhering to strict safety protocols is paramount when handling these compounds, ensuring the well-being of individuals and preventing accidents. Neglecting these precautions can lead to severe consequences, underscoring the critical importance of a safety-conscious approach.
Recognizing Potential Hazards
The dangers associated with peroxides range from relatively mild irritations to severe, life-threatening events. It's crucial to recognize and understand these potential hazards to mitigate risks effectively.
Irritation and Corrosion
Direct contact with peroxides can cause skin irritation and eye damage. The severity depends on the concentration and duration of exposure. Concentrated solutions can cause chemical burns, necessitating immediate and thorough rinsing with water.
Explosion and Fire Risks
Certain peroxides, particularly organic peroxides, are highly unstable and prone to explosive decomposition. This risk is amplified by heat, friction, impact, or contamination with incompatible materials. Even seemingly stable peroxides can become hazardous over time as they decompose, generating heat and pressure within their containers.
Essential Safety Precautions
Handling peroxides safely requires a multi-faceted approach, encompassing personal protective equipment, proper handling techniques, and awareness of potential hazards.
Personal Protective Equipment (PPE)
Always wear appropriate PPE, including chemical-resistant gloves, eye protection (goggles or face shield), and a lab coat or apron. This creates a barrier against direct contact and minimizes the risk of injury in case of spills or splashes. The specific type of glove should be selected based on the specific peroxide being handled and its concentration.
Safe Handling Practices
Avoid generating friction, impact, or heat when handling peroxides. Use non-sparking tools and ensure adequate ventilation. Never mix peroxides with incompatible materials, such as strong acids, bases, or reducing agents, as this can trigger rapid decomposition or explosion. Work in a well-ventilated area to prevent the buildup of hazardous vapors.
First Aid Measures
Know the appropriate first aid measures in case of accidental exposure. For skin contact, immediately flush the affected area with copious amounts of water for at least 15 minutes. For eye contact, irrigate with water for at least 20 minutes, holding the eyelids open. Seek medical attention immediately in either case.
Proper Storage Methods
Correct storage is crucial to maintaining the stability and safety of peroxides. Improper storage can accelerate decomposition and increase the risk of accidents.
Temperature Control
Store peroxides in a cool, dry, and well-ventilated area, away from direct sunlight and sources of heat. Elevated temperatures significantly increase the rate of decomposition. Refer to the manufacturer's recommendations for specific temperature limits.
Container Selection
Use only approved containers made of materials compatible with peroxides. Avoid metal containers, as they can catalyze decomposition. Ensure containers are properly labeled with the name of the peroxide, concentration, hazard warnings, and date of receipt.
Segregation
Store peroxides separately from incompatible materials, such as flammable substances, strong acids, bases, and reducing agents. This prevents accidental contact and minimizes the risk of a hazardous reaction. Keep a safe distance between different types of peroxides as well, as some can react with each other.
Regulations and Guidelines
Various regulatory bodies and organizations provide guidelines for the safe handling and disposal of peroxides. Adhering to these regulations is essential for legal compliance and promoting a safe working environment.
Occupational Safety and Health Administration (OSHA)
OSHA sets standards for workplace safety, including the handling of hazardous chemicals like peroxides. These standards cover topics such as hazard communication, personal protective equipment, and emergency response.
Safety Data Sheets (SDS)
Always consult the SDS for the specific peroxide being handled. The SDS provides detailed information on the chemical's properties, hazards, handling precautions, and first aid measures. It is a critical resource for understanding and mitigating risks.
Local and National Regulations
Be aware of and comply with all applicable local and national regulations regarding the storage, handling, and disposal of peroxides. These regulations may vary depending on the specific type of peroxide and its intended use. Following these ensures not only safety but also legal compliance.
The Decomposition of Peroxides: Factors and Implications
Having established a strong understanding of peroxide safety, it’s vital to consider what happens to these compounds over time. Peroxide decomposition is an unavoidable process, influenced by a variety of factors that can significantly impact their stability and effectiveness. Understanding the mechanisms and consequences of this breakdown is crucial for safe storage, handling, and maximizing the utility of peroxides in various applications.
Understanding Peroxide Decomposition
Decomposition refers to the chemical breakdown of peroxides, specifically the disintegration of the peroxide ion (O22-). This process typically involves the generation of other, more stable compounds, such as water and oxygen in the case of hydrogen peroxide.
The general equation for hydrogen peroxide decomposition is:
2 H2O2 (aq) → 2 H2O (l) + O2 (g)
However, this deceptively simple equation hides a complex process. The rate of decomposition, and the products formed, can be affected by several environmental factors.
Key Factors Influencing Decomposition
Several factors accelerate or decelerate the decomposition of peroxides. Controlling these factors is critical for maintaining peroxide stability and preventing hazardous situations.
Temperature
Temperature is a primary driver of peroxide decomposition. Higher temperatures increase the rate of decomposition, as the increased thermal energy provides the activation energy needed to break the bonds within the peroxide molecule. Consequently, storing peroxides in cool, controlled environments is vital.
Light Exposure
Exposure to light, especially ultraviolet (UV) radiation, can also catalyze decomposition. Light provides energy that can initiate the breakdown of the peroxide bond. Opaque or amber-colored containers are often used to protect peroxides from light exposure, minimizing this effect.
Catalysts
Certain substances act as catalysts, significantly accelerating the decomposition process even at moderate temperatures. These catalysts can include:
-
Transition Metals: Even trace amounts of metals like iron, copper, and manganese can drastically increase the rate of peroxide decomposition. This is why it's crucial to use high-purity containers and avoid contact with metallic surfaces.
-
Alkalis: Alkaline or basic conditions can also promote decomposition. Maintaining a slightly acidic pH often helps to stabilize peroxide solutions.
-
Rough Surfaces: The container itself can be a factor. Rough or etched surfaces can sometimes catalyze decomposition, compared to smooth, unreactive materials such as certain plastics or glass.
Concentration
Higher concentrations of peroxides can lead to accelerated decomposition rates. This is because the higher the concentration, the more peroxide molecules are available to react and initiate a chain reaction. Careful dilution and controlled concentration are, therefore, important aspects of proper handling.
Implications of Decomposition
The decomposition of peroxides has significant implications for their storage, application, and safety.
Reduced Effectiveness
As peroxides decompose, their concentration decreases, leading to reduced effectiveness in their intended applications. For example, a diluted and partially decomposed hydrogen peroxide solution will be less effective as a disinfectant or bleaching agent. Proper storage and monitoring of peroxide concentration are, therefore, essential to ensure optimal performance.
Potential Hazards
Decomposition can also pose significant safety hazards. As peroxides break down, they may release oxygen, which can increase the risk of fire or explosion in confined spaces. Furthermore, the heat generated during decomposition can accelerate the process, leading to a runaway reaction.
Pressure Buildup
Decomposition in closed containers can lead to a build-up of pressure due to the release of oxygen. This pressure can cause containers to rupture or explode, especially if the container is not designed to vent gases. Proper ventilation and the use of containers with pressure-release mechanisms are crucial for safe storage.
Mitigating Decomposition
Several strategies can be employed to minimize peroxide decomposition and ensure their stability and safety.
-
Proper Storage: Store peroxides in cool, dry, and well-ventilated areas, away from direct sunlight and heat sources.
-
Appropriate Containers: Use opaque or amber-colored containers made of materials that do not react with or catalyze the decomposition of peroxides.
-
Avoid Contamination: Prevent contamination with metals, alkalis, and other substances that can accelerate decomposition.
-
Stabilizers: Certain stabilizers can be added to peroxide solutions to inhibit decomposition.
-
Regular Monitoring: Periodically check the concentration of peroxide solutions to ensure they are within acceptable limits and have not significantly decomposed.
Understanding the factors influencing peroxide decomposition and implementing appropriate safety measures is crucial for maximizing their effectiveness and minimizing potential hazards. By controlling temperature, light exposure, and contamination, and by implementing proper storage and monitoring procedures, it is possible to significantly extend the shelf life and ensure the safe use of these valuable chemical compounds.
Video: Peroxide Ion: What It Is & Why You Should Care 🧪
FAQs About Peroxide Ions
Here are some frequently asked questions about the peroxide ion and its importance.
What exactly is a peroxide ion?
A peroxide ion is a reactive chemical species with the formula O₂²⁻. This means it's a molecule of two oxygen atoms with an overall charge of negative two. This negative charge makes it very reactive.
Why should I care about peroxide ions?
Peroxide ions are important because they play a role in various chemical processes, both natural and industrial. They're used in bleaching agents, antiseptics, and even some rocket fuels. Understanding them helps understand how those products work.
How is the peroxide ion different from regular oxygen?
Regular oxygen (O₂) is a neutral molecule that's essential for breathing. The peroxide ion (O₂²⁻) has two extra electrons, giving it a negative charge and making it much more reactive than ordinary oxygen. This difference in reactivity is key to its applications.
Are peroxide ions dangerous?
In concentrated forms, substances containing peroxide ions can be corrosive and irritating. However, in diluted solutions, such as those used in household cleaning products, they are generally safe when used as directed. Always follow safety precautions when handling any chemical containing a peroxide ion.