Unlock Pb Electron Configuration: The Ultimate Guide!
Understanding pb electron configuration, the arrangement of electrons within Lead (Pb) atoms, is fundamental to grasping its chemical behavior. The Periodic Table, a cornerstone of chemistry, provides a framework for predicting these configurations, which are governed by principles outlined in Aufbau Principle. Analyzing the pb electron configuration reveals insights crucial for fields like materials science, where specific electron arrangements influence properties. This guide offers a comprehensive exploration into pb electron configuration, simplifying complex concepts and equipping you with the knowledge to understand Lead's role in various chemical processes.
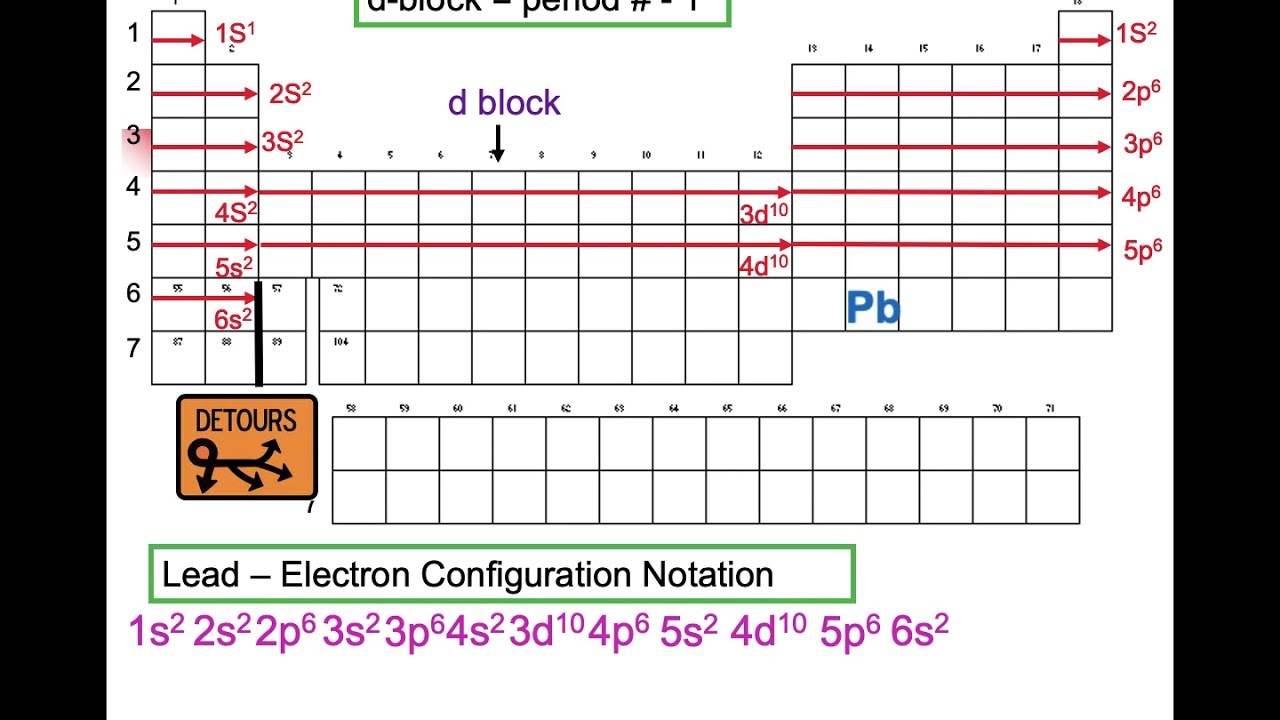
Image taken from the YouTube channel Jennifer G. , from the video titled Using P T for Electron Configuration Ex Lead .
Lead (Pb), a dense, soft, and malleable metal, has been intertwined with human civilization for millennia. From ancient plumbing systems to modern-day batteries, lead's unique properties have made it an indispensable material across various industries. However, beyond its practical applications lies a complex electronic structure that dictates its chemical behavior and ultimately influences its role in the world around us.
A Glimpse into Lead: Properties, Uses, and Significance
Lead is a heavy metal characterized by its high density, softness, and low melting point.
Its resistance to corrosion has made it a staple in construction, while its ability to block radiation finds applications in medical and industrial settings.
Historically, lead was widely used in paints, gasoline, and water pipes, though many of these uses have been phased out due to health concerns.
Despite these concerns, lead remains essential in car batteries, ammunition, cable sheathing, and certain types of solders, highlighting its continued importance in modern technology.
The Significance of Electron Configuration
The electron configuration of an element describes the arrangement of electrons within its atoms.
This arrangement governs how an element interacts with other elements, dictating its chemical properties and reactivity.
Understanding electron configuration is fundamental to comprehending chemical bonding, predicting compound formation, and explaining the periodic trends observed in the periodic table.
For lead, with its complex electronic structure, a detailed understanding of its electron configuration is crucial for explaining its unique properties and predicting its behavior in various chemical environments.
Specifically, lead's electron configuration influences its tendency to form stable compounds in +2 and +4 oxidation states, its metallic character, and its potential toxicity.
Decoding Lead's Electronic Structure: A Comprehensive Guide
This article provides a comprehensive exploration of lead's electron configuration.
We will delve into the fundamental principles governing electron arrangement, systematically derive lead's electron configuration, and explore its implications for lead's chemical properties.
By the end of this guide, you will gain a deeper appreciation for the intricate electronic architecture of this ubiquitous element and its role in shaping the world around us.
Lead's unique interaction with other elements stems directly from how its electrons are arranged. Therefore, before diving into the specifics of lead's electron configuration, it's essential to revisit the fundamental principles that govern electron arrangement within an atom. This quick review will serve as a refresher, ensuring we have a solid foundation for understanding the complexities of lead's electronic structure.
Fundamentals of Electron Configuration: A Quick Review
Electron configuration is the cornerstone of understanding an element's chemical behavior. It dictates how an atom will interact with other atoms, forming chemical bonds and ultimately determining the properties of the resulting compounds.
Defining Electron Configuration
Electron configuration describes the arrangement of electrons within an atom's electron shells and subshells. It essentially maps out where electrons are most likely to be found around the nucleus.
This arrangement is not random; it follows specific rules and principles dictated by quantum mechanics. Understanding electron configuration is critical because it directly relates to an element's chemical properties, such as its reactivity, bonding behavior, and oxidation states. It is the key to predicting how an element will behave in various chemical environments.
Atomic Number and Electron Arrangement
The atomic number of an element is equal to the number of protons in its nucleus. In a neutral atom, the number of protons is equal to the number of electrons. Therefore, the atomic number dictates the total number of electrons that need to be arranged in the electron configuration.
For example, lead (Pb) has an atomic number of 82, meaning a neutral lead atom has 82 electrons to arrange around its nucleus. This number is the starting point for determining the element's electron configuration.
Electron Orbitals: s, p, d, f
Electrons do not orbit the nucleus in fixed paths like planets around the sun. Instead, they occupy specific regions of space called atomic orbitals. These orbitals are characterized by their shapes and energy levels.
The four main types of orbitals are designated as s, p, d, and f. Each orbital can hold a specific maximum number of electrons:
- s orbitals are spherical and can hold up to 2 electrons.
- p orbitals are dumbbell-shaped and can hold up to 6 electrons (3 orbitals, 2 electrons each).
- d orbitals have more complex shapes and can hold up to 10 electrons (5 orbitals, 2 electrons each).
- f orbitals have even more complex shapes and can hold up to 14 electrons (7 orbitals, 2 electrons each).
The Aufbau Principle: Filling Orbitals
The Aufbau principle (from the German word "Aufbauen" meaning "to build up") provides a guiding rule for filling electron orbitals. It states that electrons first occupy the lowest energy orbitals available before filling higher energy orbitals.
The general filling order is: 1s, 2s, 2p, 3s, 3p, 4s, 3d, 4p, 5s, 4d, 5p, 6s, 4f, 5d, 6p, 7s, 5f, 6d, 7p. This order can be visualized using the Madelung rule, which provides a diagonal ordering of orbitals.
Hund's Rule: Maximizing Multiplicity
Hund's rule comes into play when filling degenerate orbitals, which are orbitals of the same energy level (e.g., the three p orbitals). It states that electrons will individually occupy each orbital within a subshell before doubling up in any one orbital.
This minimizes electron-electron repulsion and results in a more stable configuration. All unpaired electrons will also have the same spin (either all spin up or all spin down) before pairing occurs.
The Pauli Exclusion Principle: Orbital Occupancy
The Pauli exclusion principle is a fundamental principle of quantum mechanics that dictates that no two electrons in an atom can have the same set of four quantum numbers.
This implies that each atomic orbital can hold a maximum of two electrons, and these two electrons must have opposite spins (spin up and spin down). This principle is crucial for understanding the structure of the periodic table and the behavior of atoms.
Lead's unique interaction with other elements stems directly from how its electrons are arranged. Therefore, before diving into the specifics of lead's electron configuration, it's essential to revisit the fundamental principles that govern electron arrangement within an atom. This quick review will serve as a refresher, ensuring we have a solid foundation for understanding the complexities of lead's electronic structure.
Decoding Lead's Electron Configuration: A Step-by-Step Guide
Understanding the electron configuration of an element like lead (Pb) might seem daunting, but by breaking it down into manageable steps, we can demystify the process. This section serves as a practical guide, walking you through each step of deriving lead's electron configuration. We will use the building block principles discussed earlier to methodically arrive at the complete electronic structure of lead.
Leveraging the Atomic Number
The starting point for determining any element's electron configuration is its atomic number.
The atomic number represents the total count of protons within the nucleus of an atom. For a neutral atom, the number of protons is equivalent to the number of electrons.
Lead (Pb) has an atomic number of 82.
This tells us that a neutral lead atom possesses 82 electrons, which we need to arrange within the appropriate electron shells and subshells.
Applying the Aufbau Principle
The Aufbau principle, which translates from German to "building up," serves as a guide for filling atomic orbitals in order of increasing energy.
Electrons first occupy the lowest energy levels available before filling higher energy levels.
This principle dictates the order in which we populate the orbitals. The general filling order is: 1s, 2s, 2p, 3s, 3p, 4s, 3d, 4p, 5s, 4d, 5p, 6s, 4f, 5d, 6p, 7s, and so on.
Let's apply this principle to Lead:
- 1s orbital: This orbital can hold a maximum of 2 electrons (1s²).
- 2s orbital: This orbital can also hold a maximum of 2 electrons (2s²).
- 2p orbitals: There are three 2p orbitals, each holding 2 electrons, for a total of 6 electrons (2pā¶).
- 3s orbital: Holds 2 electrons (3s²).
- 3p orbitals: Holds 6 electrons (3pā¶).
- 4s orbital: Holds 2 electrons (4s²).
- 3d orbitals: There are five 3d orbitals, each holding 2 electrons, for a total of 10 electrons (3d¹ā°).
- 4p orbitals: Holds 6 electrons (4pā¶).
- 5s orbital: Holds 2 electrons (5s²).
- 4d orbitals: Holds 10 electrons (4d¹ā°).
- 5p orbitals: Holds 6 electrons (5pā¶).
- 6s orbital: Holds 2 electrons (6s²).
- 4f orbitals: There are seven 4f orbitals, each holding 2 electrons, for a total of 14 electrons (4f¹ā“).
- 5d orbitals: Holds 10 electrons (5d¹ā°).
- 6p orbitals: We've placed 78 electrons so far. We have 4 electrons remaining to reach 82. The 6p orbitals will then hold 2 electrons (6p²).
Visualizing with Orbital Diagrams
While electron configuration notation is concise, orbital diagrams offer a visual representation of electron filling. Each orbital is represented by a box or line, and electrons are depicted as arrows, with the direction of the arrow indicating the electron's spin.
Hund's Rule dictates that within a subshell, electrons will individually occupy each orbital before any orbital is doubly occupied. Furthermore, these single electrons will have the same spin (maximizing total spin).
However, for the purpose of brevity and clarity, we will not be illustrating a full orbital diagram for lead here, given the number of electrons involved. Instead, focus on understanding the electron configuration notation derived using the Aufbau principle.
The Full Electron Configuration of Lead
By meticulously applying the Aufbau principle and considering the electron capacities of each orbital, we arrive at the full electron configuration for lead (Pb):
1s²2s²2pā¶3s²3pā¶4s²3d¹ā°4pā¶5s²4d¹ā°5pā¶6s²4f¹ā“5d¹ā°6p²
This notation provides a complete description of how lead's 82 electrons are arranged within its electron shells and subshells. This forms the foundation for understanding its chemical behavior and properties.
Lead's unique interaction with other elements stems directly from how its electrons are arranged. Therefore, before diving into the specifics of lead's electron configuration, it's essential to revisit the fundamental principles that govern electron arrangement within an atom. This quick review will serve as a refresher, ensuring we have a solid foundation for understanding the complexities of lead's electronic structure.
Noble Gas Configuration: Simplifying Lead's Electron Structure
Writing out the full electron configuration for an element like lead (Pb), with its 82 electrons, can be quite cumbersome. Fortunately, there's a more concise method for representing electron configurations: the noble gas configuration, also known as the condensed electron configuration. This shorthand notation simplifies the process and highlights the valence electrons, which are the ones primarily involved in chemical bonding.
Understanding Noble Gas Configuration
Noble gas configuration leverages the stable electron arrangements of the noble gases (Group 18 elements) as a starting point. Each noble gas has a completely filled outer electron shell, making them exceptionally stable and unreactive. Instead of writing out the entire electron configuration from the beginning, we can use the symbol of the noble gas that precedes the element in question, enclosed in square brackets, to represent the core electrons. Then, we only need to specify the electron configuration of the remaining electrons in the outermost shells.
Identifying the Noble Gas Core for Lead
To determine the noble gas core for lead, we need to identify the noble gas that comes before lead in the periodic table. Looking at the periodic table, we find that Xenon (Xe), with an atomic number of 54, is the noble gas preceding lead (Pb, atomic number 82). Therefore, Xenon will serve as our noble gas core.
Expressing Lead's Condensed Electron Configuration
Now that we've identified Xenon as the noble gas core, we can express lead's electron configuration using the shorthand notation. The full electron configuration of Xenon represents the first 54 electrons of lead. After Xenon, we need to account for the remaining 28 electrons (82 - 54 = 28). These electrons occupy the 6s, 4f, 5d, and 6p orbitals.
Putting it all together, the noble gas configuration for lead (Pb) is:
[Xe] 6s² 4f¹ⓠ5d¹Ⱐ6p²
This notation tells us that lead has the same core electron configuration as Xenon, plus 2 electrons in the 6s subshell, 14 electrons in the 4f subshell, 10 electrons in the 5d subshell, and 2 electrons in the 6p subshell.
Advantages of Using Noble Gas Configuration
Using the noble gas configuration offers several advantages:
- Brevity: It significantly shortens the electron configuration notation, making it easier to write and read. Compare
1s²2s²2pā¶3s²3pā¶4s²3d¹ā°4pā¶5s²4d¹ā°5pā¶6s²4f¹ā“5d¹ā°6p²
with[Xe] 6s² 4f¹ⓠ5d¹Ⱐ6p²
. - Focus on Valence Electrons: It highlights the valence electrons, which are the electrons in the outermost shell and are responsible for the element's chemical behavior. In the case of lead, the 6s² 6p² electrons are clearly emphasized, making it easier to understand its bonding properties.
- Improved Understanding: Noble gas configuration can provide a more intuitive understanding of an element's electronic structure by relating it to the stable electron configuration of a noble gas. This helps to visualize how an element's electron arrangement deviates from a stable configuration and how it might interact with other elements to achieve greater stability.
In summary, the noble gas configuration is a powerful tool for simplifying and understanding the electronic structure of elements, particularly those with a large number of electrons like lead. By using this shorthand notation, we can focus on the valence electrons and gain valuable insights into an element's chemical properties.
Valence Electrons and Lead's Chemical Reactivity
Having navigated the intricacies of lead's electron configuration and its simplified noble gas representation, the spotlight now shifts to the outermost electrons. These are the valence electrons, which dictate how lead interacts with other elements and ultimately determine its chemical behavior.
Understanding Valence Electrons
Valence electrons are defined as the electrons residing in the outermost electron shell of an atom.
These electrons are the primary participants in chemical bonding, as they are the ones that are either shared, donated, or accepted when atoms combine to form molecules or compounds.
The number and arrangement of valence electrons significantly influence an element's reactivity, its preferred oxidation states, and the types of chemical bonds it can form.
Determining Lead's Valence Electrons
Based on lead's electron configuration, [Xe] 6s² 4f¹ⓠ5d¹Ⱐ6p², we can readily identify its valence electrons.
The outermost shell is the 6th shell (n=6), and it contains electrons in the 6s and 6p subshells.
Specifically, lead has two electrons in the 6s subshell (6s²) and two electrons in the 6p subshell (6p²).
Therefore, lead possesses a total of four valence electrons (2 + 2 = 4).
Valence Electrons, Oxidation States, and Chemical Properties
The presence of four valence electrons plays a pivotal role in shaping lead's chemical characteristics and its tendency to form specific oxidation states.
Lead exhibits two common oxidation states: +2 and +4. These oxidation states directly correlate with the behavior of its valence electrons.
Lead(II) Compounds
In its +2 oxidation state, lead tends to lose the two electrons present in its 6p subshell.
This results in the formation of Pb²⺠ions. Lead(II) compounds are relatively stable and commonly encountered.
Examples include lead(II) oxide (PbO) and lead(II) chloride (PbClā).
Lead(IV) Compounds
In its +4 oxidation state, lead loses all four of its valence electrons (both 6s² and 6p²).
This leads to the formation of Pbā“āŗ ions. However, lead(IV) compounds are generally less stable than lead(II) compounds.
This is due to the inert pair effect, which describes the reluctance of the 6s² electrons to participate in bonding.
An example of a lead(IV) compound is lead(IV) oxide (PbOā).
Implications for Chemical Bonding
The number of valence electrons and the resulting oxidation states directly affect lead's ability to form chemical bonds.
Lead can form both ionic and covalent bonds, depending on the electronegativity of the element it interacts with.
With highly electronegative elements like oxygen or chlorine, lead tends to form ionic bonds by losing electrons to achieve a stable electron configuration.
With elements of similar electronegativity, lead may form covalent bonds by sharing electrons.
The electronic configuration of lead, particularly its valence electrons, is therefore fundamental to understanding the wide range of compounds it forms and its diverse chemical applications.
Lead's Position in the Periodic Table: A Structural and Property Perspective
Having deciphered the intricacies of lead's valence electrons, we can now contextualize its place within the grand scheme of chemical elements: the periodic table. Its location is not arbitrary; itās a direct consequence of its electron configuration, deeply influencing its observable properties.
Locating Lead: Group 14, Period 6
Lead (Pb) resides in Group 14 (also known as the carbon group) and Period 6 of the periodic table. This positioning immediately provides valuable insights.
Elements in the same group tend to exhibit similar chemical behaviors due to having the same number of valence electrons. Elements within the same period display trends related to atomic size, ionization energy, and electronegativity.
The Electron Configuration-Position Relationship
Lead's electron configuration, [Xe] 6s² 4f¹ⓠ5d¹Ⱐ6p², directly explains its position.
The fact that its valence electrons occupy the 6s and 6p orbitals places it in Period 6. Having four valence electrons (two in the 6s and two in the 6p orbitals) is characteristic of Group 14 elements.
Implications of Electron Configuration on Properties
The partially filled p orbitals in lead contribute to its ability to form covalent bonds.
The presence of the filled f and d orbitals also influences the behavior of the outer electrons, contributing to relativistic effects (to be discussed later). These effects modify the energies of the orbitals and affect the overall properties of the element.
Periodic Trends and Lead's Characteristics
Analyzing periodic trends in conjunction with lead's location reveals further insights into its properties.
Electronegativity
Electronegativity generally increases across a period and decreases down a group.
As a result, lead has a relatively low electronegativity compared to elements in the upper right of the periodic table, which means it tends to lose electrons rather than gain them when forming compounds.
Atomic Size
Atomic size increases down a group due to the addition of electron shells.
Lead, being in Period 6, is a relatively large atom compared to lighter elements in Group 14 (carbon, silicon, germanium, tin). This larger size affects the strength of the bonds it forms, as larger atoms typically form weaker bonds.
Metallic Character
Metallic character increases down a group and decreases across a period.
Lead exhibits metallic properties, though it is less reactive than some of the alkali and alkaline earth metals. Its metallic nature is evident in its luster, conductivity, and ability to form positive ions.
Inert Pair Effect
Elements toward the bottom of group 14 show reduced stability of the +4 oxidation state, also known as the inert pair effect.
Leadās chemistry is influenced by the inert pair effect, which arises from the reluctance of the 6s² electrons to participate in bonding. This is why lead(II) compounds are usually more stable than lead(IV).
In summary, lead's position in the periodic table is not merely a label but rather a key that unlocks an understanding of its electron configuration, chemical behavior, and characteristic properties. The interplay between its electronic structure and periodic trends defines lead's unique role in chemistry.
Elements are predictably organized by their electron configurations. Understanding why elements sometimes behave unexpectedly takes us beyond the foundational rules of electron filling, especially when considering heavier elements like lead.
Beyond the Basics: Relativistic Effects and the Limits of Simple Models
While the Aufbau principle and Hund's rule provide a solid foundation for understanding electron configuration, they represent simplifications of a far more complex reality. For heavier elements like lead (Pb), these simplified models start to show their limitations, necessitating a deeper dive into advanced concepts. These include relativistic effects and the nuances of electron shielding.
The Curious Case of Relativistic Effects
Relativistic effects arise from the fact that electrons in heavier atoms, particularly those close to the nucleus, move at significant fractions of the speed of light. This is because the increased nuclear charge pulls these electrons in with tremendous force, accelerating them to very high velocities.
Impact on Orbital Energies
As electrons approach relativistic speeds, their mass increases, which in turn affects the energies of their orbitals. Specifically, the s orbitals contract and are stabilized, meaning they become lower in energy and closer to the nucleus. Conversely, the d and f orbitals expand and are destabilized.
Consequences for Lead's Properties
For lead, the relativistic stabilization of the 6s orbital has several important consequences. It makes the 6s electrons less available for bonding. This contributes to the relative inertness of lead compared to lighter elements in Group 14, such as tin (Sn).
Relativistic effects also influence the color of gold. Gold's yellowish color, rather than the silvery color of other metals, is the result of relativistic effects on the electronic transitions within the atom.
Limitations of Simplified Models
The standard electron configuration models we learn in introductory chemistry are based on the non-relativistic Schrƶdinger equation. This equation works well for lighter elements, but it begins to break down as atomic number increases.
Heavy Elements: A Different Ballgame
In heavy elements, the relativistic effects become so significant that they can no longer be ignored. They can substantially alter the orbital energies and shapes, leading to deviations from the predictions based on simple electron configuration rules.
Need for Computational Approaches
Accurately predicting the properties of heavy elements often requires sophisticated computational methods that incorporate relativistic corrections. These methods provide a more accurate picture of the electronic structure and bonding behavior.
Electron Shielding and Effective Nuclear Charge
Another factor that influences electron configuration and atomic properties is electron shielding. The inner electrons shield the outer electrons from the full positive charge of the nucleus.
Shielding Effect
Each electron experiences an effective nuclear charge (Zeff) that is less than the actual nuclear charge (Z) due to the repulsion from the other electrons.
Impact on Orbital Energies and Sizes
The degree of shielding varies depending on the orbital. Electrons in s orbitals experience less shielding than electrons in p, d, or f orbitals, because s orbitals have a higher probability density closer to the nucleus.
This difference in shielding affects the energies and sizes of the orbitals. The stronger the shielding effect, the higher the energy and the larger the orbital size. The Zeff also impacts ionization energy.
Video: Unlock Pb Electron Configuration: The Ultimate Guide!
FAQs: Understanding Lead (Pb) Electron Configuration
Here are some frequently asked questions to further clarify lead's electron configuration and related concepts.
Why is knowing the Pb electron configuration important?
Understanding the Pb electron configuration helps predict its chemical behavior and how it forms bonds with other elements. Knowing this allows chemists and material scientists to manipulate lead in specific ways.
What does the [Xe] 4f14 5d10 6s2 6p2 configuration tell us about Lead?
This condensed electron configuration shows that lead (Pb) has a Xenon core, followed by filled 4f and 5d orbitals, and finally 2 electrons in the 6s and 2 in the 6p orbitals. It emphasizes the valence electrons available for bonding. This Pb electron configuration determines its reactivity.
How does lead's electron configuration relate to its position on the periodic table?
Lead is in the 6th period (row) and the 14th group (column) of the periodic table. Its period reflects the highest energy level occupied by its electrons (n=6), and its group number relates to the number of valence electrons influencing its chemical properties.
Can lead have different electron configurations in different compounds?
Yes, lead can exist in multiple oxidation states (e.g., Pb2+ and Pb4+), resulting in the loss of electrons from its outermost shells. Each oxidation state represents a different Pb electron configuration and affects its properties.