Oxygen Bohr Model: Demystifying Atomic Structure in 60!
The Bohr model, a foundational concept in quantum mechanics, provides a simplified yet insightful representation of atomic structure. This model, particularly relevant when examining the oxygen bohr model, posits that electrons orbit the nucleus in defined energy levels. Understanding the oxygen bohr model also requires familiarity with the principles outlined by the Rutherford model, which preceded Bohr's advancements. Scientists at institutions like MIT utilize spectroscopic analysis to empirically validate and refine atomic models, including that of oxygen. Further analysis through computational chemistry using software like Gaussian provides deeper insight into electron configuration which help solidify comprehension of oxygen bohr model: Demystifying Atomic Structure in 60!
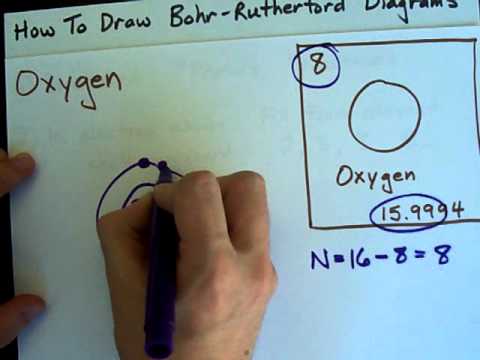
Image taken from the YouTube channel chemistNATE , from the video titled How to Draw Bohr-Rutherford Diagrams - Oxygen .
Oxygen, the very air we breathe, is far more than just a life-sustaining gas.
It's a cornerstone of our understanding of chemistry and biology, a pivotal element that drives countless reactions and processes essential to life as we know it.
But to truly grasp oxygen's role, we must delve into the realm of atomic structure.
The ability to decipher the architecture of atoms has been instrumental in unlocking the secrets of matter itself, paving the way for advancements across diverse scientific fields.
And at the heart of this journey lies a model that, while not perfect, provided a crucial stepping stone: the Bohr Model.
The Oxygen Atom: A Fundamental Building Block
Oxygen (O) is ubiquitous, comprising roughly 21% of Earth's atmosphere and playing a crucial role in respiration, combustion, and a vast array of chemical reactions.
Its ability to readily form bonds with other elements makes it an indispensable component of water, organic molecules, and countless minerals.
Understanding oxygen's atomic structure is, therefore, paramount to understanding the world around us.
Unveiling the Secrets of Atomic Structure
The quest to understand atomic structure has been a driving force in scientific discovery for over a century.
By peering into the infinitesimally small, scientists have unlocked the secrets of chemical bonding, material properties, and the very nature of matter.
Each new insight into atomic arrangements brings a better understanding of the universe, with oxygen being no exception.
This pursuit has not only fueled technological advancements but has also deepened our fundamental understanding of the cosmos.
The Bohr Model: A Foundation for Atomic Theory
The Bohr Model, proposed by Niels Bohr in 1913, revolutionized our understanding of the atom.
It presented a simplified, yet remarkably insightful, picture of atomic structure, depicting electrons orbiting the nucleus in discrete energy levels or shells.
While later superseded by more sophisticated quantum mechanical models, the Bohr Model remains a valuable tool for visualizing and understanding the basic principles of atomic behavior.
Its intuitive nature makes it an excellent starting point for anyone seeking to explore the fascinating world of atoms.
Niels Bohr: A Pioneer of Atomic Physics
Niels Bohr's contribution to atomic theory cannot be overstated.
His model, though later refined, provided the first concrete framework for understanding how electrons are arranged within atoms and how they interact with energy.
Bohr's work not only earned him the Nobel Prize in Physics in 1922 but also laid the groundwork for the development of quantum mechanics and our modern understanding of atomic structure.
His insights continue to inspire scientists and shape our understanding of the fundamental building blocks of matter.
The Bohr Model: A Simplified Solar System for Atoms
The Bohr model, conceived by Niels Bohr in 1913, marked a pivotal moment in our understanding of atomic structure. It boldly proposed a model that, while ultimately superseded, laid the groundwork for future advancements in quantum mechanics. By introducing revolutionary concepts, it bridged the gap between classical physics and the emerging quantum world, providing a tangible, if simplified, image of the atom.
Quantized Energy Levels: A Quantum Leap
One of the most groundbreaking aspects of the Bohr model was the idea of quantized energy levels. This concept dictates that electrons within an atom can only exist in specific, discrete energy states. Unlike classical physics, which allows for a continuous range of energy values, the Bohr model postulates that electrons are restricted to well-defined energy levels, much like steps on a staircase.
This quantization of energy has profound implications. It explains why atoms absorb and emit light at specific wavelengths, corresponding to the energy differences between these allowed energy levels. When an electron absorbs energy, it jumps to a higher energy level; when it falls back down, it emits energy in the form of a photon with a characteristic wavelength.
Electron Orbits: A Planetary Analogy
The Bohr model further simplifies the atom by envisioning electrons orbiting the nucleus in discrete, circular paths or shells. These shells are analogous to the orbits of planets around the sun in our solar system, hence the model's common moniker as a "solar system" model of the atom.
Each orbit corresponds to a specific energy level. Electrons in orbits closer to the nucleus possess lower energy, while those in outer orbits have higher energy. The model postulates that electrons can only exist in these defined orbits and cannot occupy the space in between.
This planetary analogy, while visually intuitive, is a simplification of reality. It suggests that electrons follow well-defined trajectories, which is not entirely accurate according to modern quantum mechanics.
Limitations of the Bohr Model: A Stepping Stone to Quantum Mechanics
Despite its significant contributions, the Bohr model is not without limitations. Its simplicity, while helpful for initial understanding, falls short when attempting to describe more complex atoms with multiple electrons. The model struggles to accurately predict the behavior of these multi-electron atoms.
The Bohr model also fails to account for the wave-particle duality of electrons, a fundamental principle of quantum mechanics. It treats electrons solely as particles orbiting the nucleus, neglecting their wave-like properties, which play a crucial role in their behavior.
Furthermore, the model struggles to explain the fine details of atomic spectra, such as the splitting of spectral lines in the presence of magnetic fields (the Zeeman effect). These limitations underscored the need for a more sophisticated model of the atom.
To overcome these shortcomings, scientists developed more advanced models based on quantum mechanics. These models, such as the Schrödinger model, provide a more accurate and complete picture of atomic structure, incorporating the wave-like nature of electrons and the complex interactions between them.
While quantum mechanics offers a more comprehensive description, the Bohr model remains valuable as an introductory tool. It provides a simplified and intuitive framework for understanding the fundamental principles of atomic structure and the quantization of energy, making it a crucial stepping stone in the journey towards mastering quantum mechanics.
The Bohr model, with its planetary analogy, offers a simplified yet insightful lens through which we can examine the structure of individual atoms. This framework, while not perfectly mirroring reality, allows us to visualize the arrangement of electrons and understand the fundamental properties of elements. Let's now turn our attention to a particularly vital element: oxygen.
Oxygen Under the Bohr Microscope: Unveiling Its Atomic Arrangement
Applying the Bohr model specifically to the oxygen atom allows us to visualize its atomic structure and understand its key characteristics. Let's take a closer look.
Defining the Oxygen Atom in the Bohr Model
Within the Bohr model, the oxygen atom is defined by its constituent particles. It comprises 8 protons and typically 8 neutrons in its nucleus. Orbiting this nucleus are 8 electrons, arranged in specific energy levels or shells.
Oxygen's Atomic Number: A Defining Characteristic
The atomic number of oxygen is 8. This signifies that every oxygen atom possesses 8 protons in its nucleus.
This number is not arbitrary; it is what unequivocally defines oxygen as an element, distinguishing it from all others. Any atom with a different number of protons is, by definition, a different element.
Electron Distribution in Shells: The K and L Shells
According to the Bohr model, electrons reside in specific energy levels or shells around the nucleus. The first shell, closest to the nucleus, is the K shell; the second is the L shell.
Oxygen, with its 8 electrons, arranges them with 2 electrons in the K shell and the remaining 6 electrons in the L shell. This configuration is crucial to understanding oxygen's behavior.
Electron Configuration: Filling the Energy Levels
The electron configuration of oxygen, expressed as 1s² 2s² 2p⁴, details how electrons are distributed among the available energy levels and sublevels.
Within the Bohr model framework, this notation reveals that the K shell (represented by 1s²) is completely filled with 2 electrons. The L shell, however, contains 6 electrons distributed among the 2s (2s²) and 2p (2p⁴) sublevels.
Valence Electrons: The Key to Chemical Bonding
The valence electrons are those residing in the outermost shell of an atom. For oxygen, these are the 6 electrons in the L shell.
These valence electrons are critically important because they dictate how oxygen interacts with other atoms, determining its chemical properties and bonding behavior. Oxygen's tendency to form two chemical bonds stems directly from its need to gain two more electrons to achieve a stable octet in its valence shell.
The Nucleus: Protons and Neutrons
While the Bohr model focuses primarily on electron arrangement, it's essential to acknowledge the role of the nucleus.
Oxygen's nucleus contains 8 protons, as defined by its atomic number, and typically 8 neutrons. Protons contribute to the positive charge of the nucleus, while neutrons contribute to its mass. The total number of protons and neutrons determines the atomic mass of the oxygen atom.
The Bohr model, with its planetary analogy, offers a simplified yet insightful lens through which we can examine the structure of individual atoms. This framework, while not perfectly mirroring reality, allows us to visualize the arrangement of electrons and understand the fundamental properties of elements. Let's now turn our attention to a particularly vital element: oxygen.
Energy Leaps and Light: Oxygen's Atomic Dance
The Bohr model elegantly illustrates how atoms interact with energy, specifically focusing on the behavior of electrons within the atom. This interaction is a dynamic dance, where electrons absorb or release energy, moving between distinct energy levels. Understanding this process is key to grasping how oxygen, and indeed all elements, interact with light and other forms of electromagnetic radiation.
Energy Absorption and Emission in Oxygen Atoms
Electrons within an oxygen atom don't remain static. They can absorb energy from external sources like light or heat.
When an electron absorbs a specific amount of energy, it instantaneously jumps from its current, lower energy level to a higher energy level.
This jump is not gradual; it's an abrupt transition to a more energetic state.
Conversely, an electron in a higher energy level can spontaneously drop back down to a lower energy level. This transition results in the emission of energy, often in the form of light.
Ground State and Excited States: Defining Electron Energy Levels
An electron typically resides in its ground state, which is the lowest possible energy level it can occupy within the atom. Think of it as its "default" position.
However, when an electron absorbs energy, it transitions to an excited state.
This excited state is temporary. The electron will eventually return to its ground state, releasing the excess energy it absorbed.
The critical distinction is that the ground state is stable, while the excited state is inherently unstable, prompting the electron to seek a lower energy configuration.
Photons: The Currency of Energy Exchange
The Bohr model connects electron transitions to the absorption and emission of photons.
A photon is a quantum of electromagnetic radiation, essentially a discrete packet of light energy.
When an electron jumps to a higher energy level, it absorbs a photon with precisely the right amount of energy to match the energy difference between the two levels.
When an electron drops to a lower energy level, it emits a photon with an energy equal to the energy difference between the levels.
Because energy levels are quantized, only photons of specific energies (and therefore specific wavelengths) can be absorbed or emitted by an oxygen atom.
This principle explains why oxygen absorbs and emits light at specific wavelengths, creating its unique spectral fingerprint.
Oxygen's Place in the Periodic Table
Oxygen's electron configuration dictates its position in the periodic table.
As element number 8, oxygen belongs to Group 16 (also known as the chalcogens).
Its electron configuration (1s² 2s² 2p⁴) reveals that it has six valence electrons, meaning six electrons in its outermost shell.
This valence electron count determines its chemical properties and its ability to form bonds with other elements.
The Valence of Oxygen: A Two-Bonding Affinity
The valence of an element refers to its capacity to form chemical bonds.
Oxygen has a valence of 2, meaning it typically forms two chemical bonds.
This stems from its need to gain two more electrons to achieve a stable octet (eight electrons) in its outermost shell.
Oxygen's strong tendency to form two bonds is crucial for its role in countless chemical compounds, including water (H₂O) and carbon dioxide (CO₂).
Energy absorption and emission in oxygen, viewed through the lens of the Bohr model, provides a foundational understanding of how atoms interact with light. However, the Bohr model is, as we've noted, a simplification. It’s time to acknowledge the model's limitations and peek into the more nuanced world of modern atomic theory.
Beyond Bohr: A Modern Perspective on the Atom
The Bohr model, despite its pedagogical value, operates under several key assumptions that ultimately limit its accuracy and applicability. While it provides a visually intuitive picture of atomic structure, it falls short when confronted with the complexities of real-world atomic behavior.
The Bohr Model's Boundaries
One significant limitation is its inability to accurately predict the behavior of atoms with more than one electron. The model works reasonably well for hydrogen, with its single electron, but its predictions become increasingly inaccurate as the number of electrons increases. This stems from its failure to account for the intricate interactions between multiple electrons within an atom.
Furthermore, the Bohr model treats electrons as particles orbiting the nucleus in well-defined paths, akin to planets around the sun. However, modern physics has revealed that electrons exhibit a wave-particle duality, meaning they behave as both particles and waves. The Bohr model completely disregards this wave-like nature, a critical oversight.
Quantum Mechanics: A More Complete Picture
The limitations of the Bohr model paved the way for the development of more sophisticated models rooted in quantum mechanics. Quantum mechanics offers a fundamentally different perspective on atomic structure, one that embraces the wave-like behavior of electrons and replaces the concept of fixed orbits with electron orbitals.
Electron Orbitals and Probability Distributions
Instead of orbiting the nucleus in defined paths, electrons exist in regions of space called orbitals. Orbitals are not physical pathways but rather mathematical descriptions of the probability of finding an electron in a particular location around the nucleus.
These orbitals have distinct shapes and energy levels, and they are described by a set of quantum numbers. The concept of probability distributions is central to this model; we can only predict the likelihood of finding an electron at a given point, not its precise location at any given time.
A Foundation for Understanding
While quantum mechanics provides a far more accurate and comprehensive understanding of atomic structure, it is also significantly more complex. The mathematical underpinnings of quantum mechanics can be daunting, requiring advanced knowledge of physics and mathematics.
However, the Bohr model, despite its limitations, provides a valuable and intuitive starting point for grasping the fundamental concepts of atomic structure. It allows us to visualize the arrangement of electrons within an atom and to understand the basic principles of energy quantization. Even though it's not the full story, it sets the stage for further exploration into the fascinating world of quantum mechanics.
Video: Oxygen Bohr Model: Demystifying Atomic Structure in 60!
Oxygen Bohr Model FAQs
Need a quick recap or have questions about the oxygen Bohr model? Here are some frequently asked questions:
What are the key features of the oxygen Bohr model?
The oxygen Bohr model depicts the atom with a nucleus containing protons and neutrons, surrounded by electrons orbiting in specific energy levels or shells. Oxygen has 8 electrons, with 2 in the first shell and 6 in the second shell. These shells are quantized, meaning electrons can only exist at discrete energy levels.
How does the Bohr model help understand oxygen's reactivity?
The oxygen Bohr model visually represents oxygen's electron configuration. The 6 electrons in its outer shell indicate it needs 2 more electrons to achieve a stable octet. This high electron affinity explains oxygen's strong tendency to form chemical bonds, making it highly reactive.
Is the Bohr model an accurate representation of oxygen's atomic structure?
The Bohr model is a simplified representation and has limitations. While it effectively illustrates electron shells and energy levels, it doesn't fully account for the complex behavior of electrons or the shapes of atomic orbitals described by quantum mechanics. It provides a foundational understanding but isn't a complete depiction of the oxygen Bohr model.
What are the limitations of using the oxygen Bohr model for more complex molecules?
For simple atoms like hydrogen, the Bohr model is reasonably accurate. However, when dealing with more complex molecules involving oxygen, the model struggles to explain bonding types, molecular shapes, and electron sharing in covalent bonds. More advanced models like the quantum mechanical model are required for accurate predictions of molecular behavior.