Nerve Endings in Skin: Sensitivity & Function
Nerve endings in skin, acting as crucial components of the somatosensory system, facilitate the body's interaction with its environment. Specifically, mechanoreceptors located in the dermis enable the perception of tactile stimuli, contributing significantly to the sense of touch. These specialized nerve endings in skin, studied extensively using advanced techniques in neurophysiology, are vital for understanding sensory transduction. Moreover, conditions such as peripheral neuropathy can impair the function of nerve endings in skin, resulting in altered sensory experiences.
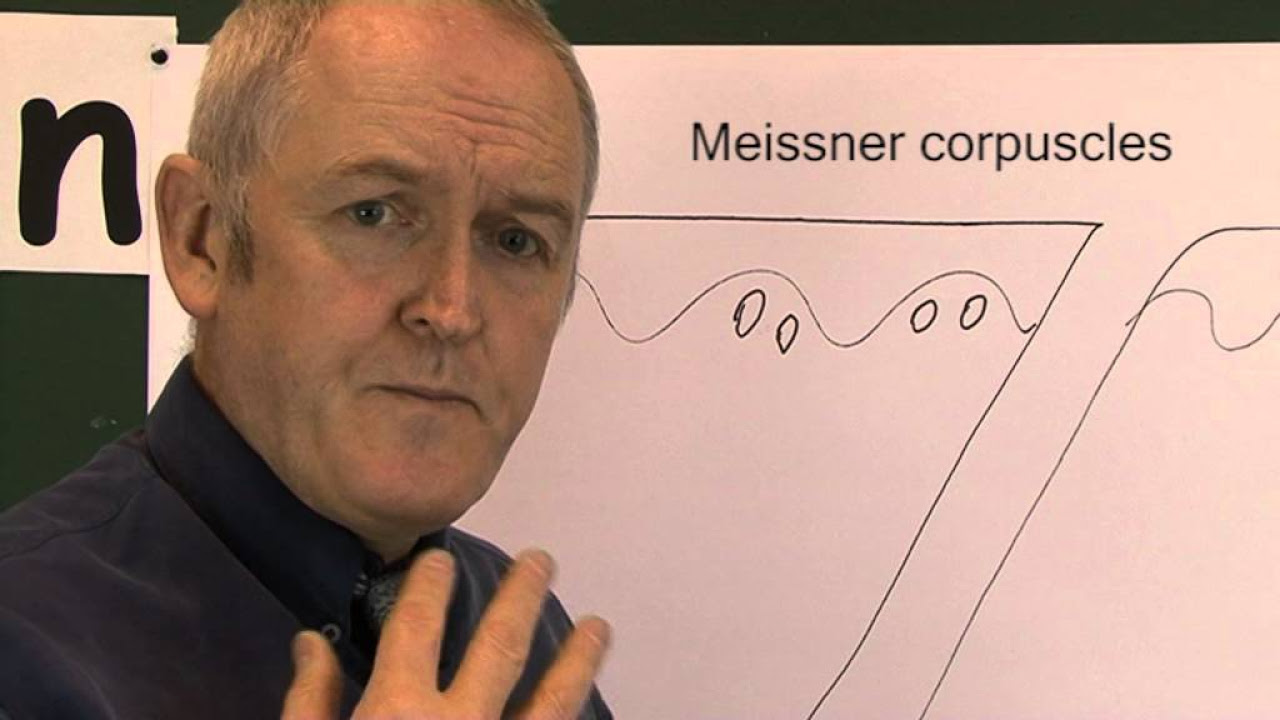
Image taken from the YouTube channel Dr. John Campbell , from the video titled Sensory nerve receptors in the skin .
Somatosensation, a critical aspect of our interaction with the environment, encompasses a wide array of sensory modalities. It's far more than just "touch".
This intricate system allows us to perceive and interpret stimuli acting upon our body's surface and internal tissues.
It provides a constant stream of information crucial for navigation, protection, and overall survival.
Defining the Scope of Somatosensation
Somatosensation is not a single sense, but a collection of distinct sensory experiences. These include:
-
Touch sensation: The ability to perceive pressure, texture, and vibration.
-
Temperature sensation: The capacity to detect warmth and cold.
-
Pain sensation (nociception): The perception of tissue damage or potentially harmful stimuli.
-
Itch sensation (pruritus): The sensation that provokes the desire to scratch.
-
Proprioception: The sense of body position and movement in space.
Each of these modalities relies on specialized receptors and neural pathways to transmit information to the central nervous system.
The Importance of Somatosensation in Daily Life
Somatosensation plays a vital role in nearly every aspect of our daily lives.
From the simple act of walking without stumbling, to expertly playing an instrument, or quickly reacting to a hot stove, this sensory system is constantly at work.
Without it, we would be severely limited in our ability to interact with the world around us.
-
Navigation and Interaction: We use touch to grasp objects, determine their texture, and manipulate them effectively.
-
Protection: Pain and temperature sensation alert us to potential dangers, allowing us to avoid injury.
-
Balance and Coordination: Proprioception is essential for maintaining balance and coordinating movements.
-
Social Interaction: Touch plays a crucial role in social bonding and communication.
A Glimpse at the Anatomical Structures Involved
The anatomical basis of somatosensation is complex and multifaceted. It involves a network of structures working in concert.
The primary sensory organ is the skin, which houses a variety of specialized receptors.
These receptors convert mechanical, thermal, and chemical stimuli into electrical signals.
These signals are then transmitted via sensory neurons to the spinal cord and brain.
The brain, in turn, interprets these signals to create our conscious perception of touch, temperature, pain, itch, and body position. Further exploration of these structures will be addressed in the next section.
Anatomy of Touch: The Skin as a Sensory Gateway
Somatosensation, a critical aspect of our interaction with the environment, encompasses a wide array of sensory modalities. It's far more than just "touch." This intricate system allows us to perceive and interpret stimuli acting upon our body's surface and internal tissues. It provides a constant stream of information crucial for navigating the world and maintaining our well-being. Our journey into understanding somatosensation begins with an exploration of the skin, the primary sensory organ that acts as the initial gateway for tactile information.
The Skin: A Multi-Layered Sensory Organ
The skin, the body's largest organ, is a complex, multi-layered structure responsible for a vast array of functions, including protection, temperature regulation, and, crucially, sensory perception. Its ability to detect and transmit tactile information arises from its intricate organization into three primary layers: the epidermis, the dermis, and the hypodermis (subcutaneous layer).
Epidermis: The Outer Barrier
The epidermis, the outermost layer, is primarily composed of epithelial cells arranged in multiple layers. While it lacks blood vessels, it plays a crucial role in sensory perception through specialized nerve endings that extend into this layer. These nerve endings are responsible for detecting fine touch, temperature, and pain. The epidermis acts as a protective barrier and a sensory interface.
Dermis: The Sensory Hub
Beneath the epidermis lies the dermis, a thicker layer composed of connective tissue. This layer houses a rich network of blood vessels, nerve endings, and specialized sensory receptors. It is within the dermis that we find the mechanoreceptors responsible for detecting pressure, vibration, and texture. The dermis is essential for our ability to discriminate between various tactile stimuli.
Hypodermis: Anchoring and Insulation
The hypodermis, or subcutaneous layer, is the deepest layer of the skin, primarily composed of adipose tissue and connective tissue. While its main functions are insulation and energy storage, it also contains nerve endings that contribute to the perception of deep pressure and vibration. The hypodermis anchors the skin to underlying tissues.
Skin Appendages: Amplifying Sensory Input
Beyond the primary layers of the skin, specialized structures known as skin appendages further enhance our sensory capabilities. These appendages include hair follicles, sweat glands, and sebaceous glands.
Hair Follicles
Hair follicles are involved in tactile sensation. Nerve endings surrounding the base of each hair follicle are exquisitely sensitive to movement, allowing us to detect even the slightest displacement of a hair. This mechanism is particularly important for detecting insects crawling on the skin or changes in airflow.
Sweat Glands
While primarily involved in temperature regulation, sweat glands are innervated by nerve fibers. These fibers can contribute to sensory input, particularly in response to changes in skin hydration.
Sebaceous Glands
Sebaceous glands secrete sebum, an oily substance that lubricates the skin and hair. While their primary function is not sensory, they may contribute indirectly to tactile perception by affecting the skin's texture and pliability.
Neural Pathways: From Skin to Brain
The sensory information gathered by the skin's receptors must be transmitted to the central nervous system for processing and interpretation. This occurs via a network of neural pathways that relay signals from the periphery to the brain. Sensory neurons in the skin possess axons that bundle together to form larger peripheral nerves. These nerves then travel towards the spinal cord.
Upon reaching the spinal cord, sensory axons enter the dorsal horn, the posterior gray matter. From here, the sensory information ascends to the brain via specific pathways such as the dorsal column-medial lemniscus pathway (for fine touch, vibration, and proprioception) and the spinothalamic tract (for pain and temperature). These pathways ultimately lead to the somatosensory cortex, located in the parietal lobe of the brain. Within this cortical region, the incoming sensory information is processed, allowing us to consciously perceive and interpret tactile stimuli.
The Senses Within: Exploring Sensory Receptors in the Skin
Having explored the skin's structure and its role as a sensory gateway, let's delve deeper into the specific sensory receptors residing within its layers. These specialized cells are responsible for detecting a wide array of stimuli, from the gentle caress of a breeze to the sharp sting of pain, each playing a critical role in how we perceive and interact with the world.
Mechanoreceptors: The Body's Tactile Sensors
Mechanoreceptors are sensory receptors that respond to mechanical pressure or distortion. They are essential for our sense of touch, enabling us to perceive texture, pressure, vibration, and stretch.
Types of Mechanoreceptors
-
Meissner's Corpuscles: Located in the dermal papillae of glabrous (hairless) skin, such as fingertips and lips, Meissner's corpuscles are highly sensitive to light touch and texture. Their abundance in these areas contributes to our refined ability to discriminate fine details.
-
Pacinian Corpuscles: Found deep within the dermis and subcutaneous tissue, Pacinian corpuscles are specialized for detecting deep pressure and high-frequency vibrations. Their rapid adaptation makes them particularly well-suited for sensing changes in pressure rather than sustained stimuli.
-
Merkel Discs: Located in the basal epidermis, Merkel discs are responsible for detecting sustained touch and pressure. They are slow-adapting receptors, providing continuous information about the pressure being applied to the skin.
-
Ruffini Endings: Also slow-adapting, Ruffini endings are found in the dermis and joint capsules. They are sensitive to skin stretch and sustained pressure, playing a role in proprioception and the perception of joint movement.
-
Hair Follicle Receptors: These receptors are located around hair follicles and detect movement across the skin. They are particularly sensitive to the deflection of hairs, providing an early warning system for potential threats.
Thermoreceptors: Sensing Hot and Cold
Thermoreceptors are specialized sensory receptors that respond to changes in temperature. They allow us to detect both hot and cold stimuli, helping us maintain a stable internal body temperature and avoid potentially harmful temperature extremes.
Types of Thermoreceptors
-
Cold Receptors: These receptors are activated by cooling sensations, typically in the range of 10-40°C (50-104°F). They are more numerous than heat receptors, reflecting our greater sensitivity to cold stimuli.
-
Heat Receptors: These receptors are activated by warming sensations, typically in the range of 30-45°C (86-113°F). Beyond this range, nociceptors are activated, signaling the potential for tissue damage.
Nociceptors: The Body's Pain Alarms
Nociceptors are sensory receptors that respond to noxious or potentially damaging stimuli. They are essential for pain perception, alerting us to potential threats and triggering protective reflexes.
Types of Nociceptors
Nociceptors are classified based on the types of stimuli they respond to:
-
Polymodal Nociceptors: These receptors respond to a variety of stimuli, including mechanical, thermal, and chemical irritants. Their broad sensitivity makes them effective at detecting a wide range of potential threats.
-
Mechanical Nociceptors: These receptors are activated by intense pressure or mechanical deformation. They are particularly important for detecting injuries to the skin and underlying tissues.
-
Thermal Nociceptors: These receptors respond to extreme temperatures, both hot and cold, that could cause tissue damage.
-
Silent Nociceptors: These receptors are typically unresponsive to stimuli under normal conditions. However, they become sensitized and activated during inflammation, contributing to the increased pain sensitivity associated with inflammatory conditions.
Role in Pain Perception
Nociceptors play a crucial role in distinguishing between acute and chronic pain:
-
Acute Pain: This is sudden, short-lived pain that is typically caused by a specific injury or stimulus. It serves as an immediate warning signal, prompting us to take action to avoid further harm.
-
Chronic Pain: This is persistent, long-lasting pain that can continue for weeks, months, or even years. It can be caused by a variety of factors, including nerve damage, inflammation, and psychological factors. Chronic pain often involves complex changes in the nervous system, leading to altered pain processing.
Pruriceptors: Detecting Itch
Pruriceptors are specialized sensory receptors that respond to itch stimuli. While the mechanisms underlying itch perception are not fully understood, pruriceptors play a crucial role in triggering the sensation of itch, prompting us to scratch and remove potential irritants from the skin.
Pruriceptors and Itch Detection
- Pruriceptors: Specifically attuned to detecting itch-inducing substances, these receptors trigger the neural pathways leading to the sensation of itch.
Understanding the diverse array of sensory receptors within the skin is fundamental to comprehending the complexity of somatosensation. Each type of receptor contributes unique information about our environment, enabling us to interact with the world in a meaningful and adaptive way.
From Skin to Brain: Neural Pathways and Signal Transduction
Having explored the skin's structure and its role as a sensory gateway, let's delve deeper into how the body transmits sensory information from the periphery to the brain. This intricate process involves a complex network of sensory neurons, specialized nerve fibers, and the critical conversion of stimuli into electrical signals. Understanding these mechanisms is key to unraveling the complexities of somatosensation.
Sensory Neurons: The Messengers of Touch
Sensory neurons are the primary cells responsible for relaying sensory information from the periphery to the central nervous system (CNS). These neurons are pseudo-unipolar, meaning they have a single process that splits into two branches: one extending to the periphery to receive sensory input and the other projecting to the spinal cord or brainstem. They act as conduits, bridging the gap between external stimuli and the brain's interpretive centers.
The cell bodies of sensory neurons are located in the dorsal root ganglia (DRG) for sensations from the body and in the trigeminal ganglia for sensations from the face. This strategic placement allows for efficient transmission of signals without the need for the cell body to be directly involved in signal propagation.
Afferent Nerve Fibers: Speed and Specificity
Afferent nerve fibers, acting as biological wires, carry sensory signals from the skin to the spinal cord and brain. The speed at which these fibers conduct signals, and the types of sensations they transmit, depend largely on their diameter and degree of myelination. The main types include:
-
A-beta fibers: These are large, myelinated fibers that transmit information about touch, pressure, and vibration at high speeds.
Their rapid transmission enables us to quickly perceive and react to tactile stimuli.
-
A-delta fibers: These are smaller, myelinated fibers that transmit sharp, localized pain and temperature sensations.
They play a crucial role in alerting us to acute injuries or temperature extremes.
-
C fibers: These are small, unmyelinated fibers that transmit dull, aching pain, temperature, and itch sensations.
Their slower conduction velocity contributes to the lingering and diffuse nature of these sensations.
-
Free Nerve Endings: These are not technically fibers, but rather the terminal branches of sensory neurons that are found throughout the skin and other tissues.
They act as receptors. These detect a wide range of stimuli, including pain, temperature, and itch, and can be associated with both A-delta and C fibers.
The Importance of Neural Structures: Myelin and Nodes
Neural structures such as the myelin sheath and Nodes of Ranvier are critical to the efficiency and speed of nerve signal transmission. The myelin sheath is a fatty, insulating layer that surrounds the axons of many nerve fibers, allowing electrical signals to jump from one node of Ranvier to the next in a process called saltatory conduction.
This increases the speed of transmission significantly compared to unmyelinated fibers. Nodes of Ranvier are the gaps in the myelin sheath where the axon membrane is exposed.
These gaps are rich in ion channels. This allows for the regeneration of the action potential, which ensures that the signal remains strong as it travels along the nerve fiber.
Sensory Transduction: Converting Stimuli into Electrical Signals
Sensory transduction is the fundamental process by which sensory stimuli are converted into electrical signals that can be interpreted by the nervous system. This process occurs at the sensory receptors, where mechanical, thermal, or chemical stimuli activate specialized ion channels in the receptor cell membrane.
For example, mechanoreceptors in the skin respond to mechanical stimuli like pressure or vibration by opening ion channels that allow ions to flow into the cell, generating a receptor potential. If the receptor potential is strong enough, it will trigger an action potential in the sensory neuron.
Action Potential: The Language of the Nervous System
The action potential is a rapid, transient change in the electrical potential across the nerve cell membrane. It travels along the nerve fiber and serves as the primary means of communication within the nervous system.
When the receptor potential reaches a certain threshold, it triggers the opening of voltage-gated sodium channels, causing a rapid influx of sodium ions into the cell and depolarizing the membrane. This depolarization triggers the action potential, which then propagates along the axon to the next neuron in the pathway.
Dermatomes: Mapping the Body's Sensory Landscape
A dermatome is an area of skin innervated by a single spinal nerve. Dermatomes provide a map of the body's sensory surface, allowing clinicians to assess the integrity of specific spinal nerves by testing sensation in corresponding areas of the skin.
Damage to a spinal nerve can result in altered or absent sensation in the dermatome innervated by that nerve. Dermatome maps are valuable diagnostic tools in identifying the level and extent of spinal cord injuries or nerve compression.
Receptive Fields: The Sensory Neuron's Area of Expertise
Each sensory neuron has a receptive field, which is the area of skin that, when stimulated, activates that neuron. Receptive fields vary in size and sensitivity, depending on the type and location of the receptor.
For example, receptive fields in the fingertips are small and densely packed with receptors, allowing for high tactile acuity. In contrast, receptive fields on the back are larger and less densely packed, resulting in lower tactile acuity. The size and overlap of receptive fields contribute to our ability to discriminate fine details and localize stimuli on the skin.
Central Processing: How the Brain Interprets Sensory Information
Having explored the skin's structure and its role as a sensory gateway, let's delve deeper into how the body transmits sensory information from the periphery to the brain. This intricate process involves a complex network of sensory neurons, specialized nerve fibers, and the critical conversion of stimuli into interpretable signals.
The journey of somatosensory information to the brain is a fascinating illustration of neural efficiency and organization. It involves several key relays and processing centers.
Spinal Cord Pathways: Relaying Sensory Signals
The spinal cord acts as the initial relay station, receiving afferent sensory information from the body. Two primary pathways are responsible for transmitting distinct types of somatosensory data: the dorsal column-medial lemniscus pathway and the spinothalamic tract.
Dorsal Column-Medial Lemniscus Pathway
This pathway is primarily responsible for conveying fine touch, vibration, and proprioceptive information. Its high degree of spatial resolution is essential for discriminating subtle tactile details and accurately perceiving body position.
Ascending sensory fibers from touch and proprioceptive receptors enter the spinal cord and ascend ipsilaterally in the dorsal columns (fasciculus gracilis and fasciculus cuneatus) without synapsing.
These fibers then synapse in the medulla, and secondary neurons decussate (cross over) and ascend via the medial lemniscus to the thalamus.
Spinothalamic Tract
In contrast to the dorsal column pathway, the spinothalamic tract primarily transmits pain, temperature, and crude touch sensations.
This pathway is crucial for alerting the brain to potentially harmful stimuli and mediating reflexive responses to pain.
Primary afferent fibers synapse in the dorsal horn of the spinal cord, and secondary neurons decussate and ascend contralaterally in the spinothalamic tract to the thalamus.
Thalamic Relay and Cortical Processing
The thalamus serves as a critical relay station, filtering and directing sensory information to appropriate cortical areas. The ventral posterolateral (VPL) nucleus of the thalamus is the primary recipient of somatosensory input from the body.
From the thalamus, sensory information is projected to the cerebral cortex, specifically the somatosensory cortex.
Cortical Representation of Somatosensation
The somatosensory cortex, located in the parietal lobe, is the primary cortical area responsible for processing somatosensory information. It is organized into distinct regions, including the primary somatosensory cortex (S1) and the secondary somatosensory cortex (S2).
Primary Somatosensory Cortex (S1)
S1 is further subdivided into four cytoarchitectonic areas: 3a, 3b, 1, and 2. Each area processes distinct aspects of somatosensory information, such as touch, pressure, and proprioception.
S1 exhibits a somatotopic organization, often depicted as a "sensory homunculus," where different body regions are represented in a spatially organized manner.
The size of cortical representation for a given body part is proportional to its sensory acuity, reflecting the density of sensory receptors and the importance of that region for tactile discrimination.
Secondary Somatosensory Cortex (S2)
S2 receives input from S1 and integrates somatosensory information from both sides of the body.
It plays a role in more complex somatosensory processing, such as object recognition by touch (stereognosis) and tactile memory.
The Role of the Cortex in Sensory Perception and Discrimination
The somatosensory cortex is essential for our ability to perceive and discriminate a wide range of tactile stimuli. It allows us to identify objects by touch, judge the size and shape of objects, and detect subtle differences in texture and temperature.
Moreover, the cortex contributes to our sense of body ownership and spatial awareness. Through the integration of somatosensory information with other sensory modalities, the brain constructs a coherent and unified representation of the body and its environment.
Damage to the somatosensory cortex can result in a variety of sensory deficits, including impaired tactile discrimination, loss of proprioception, and neglect of one side of the body. Understanding the complex cortical processing of somatosensory information is essential for developing effective treatments for these debilitating conditions.
When Sensation Goes Wrong: Clinical Aspects of Somatosensation
Having explored how the brain interprets sensory information, it is crucial to consider what happens when this intricate system malfunctions. The clinical aspects of somatosensation encompass a range of disorders, from debilitating pain conditions to profound sensory loss, significantly impacting the lives of affected individuals. Understanding these conditions is paramount for effective diagnosis and management.
Pain Disorders: A Deep Dive
Pain, while a protective mechanism, can become a chronic and debilitating condition when somatosensory pathways are disrupted. Various pain disorders highlight the complexity of this sensory experience.
Neuropathic Pain: The Tangled Wires
Neuropathic pain arises from damage or dysfunction within the nervous system itself. This can occur due to injury, disease, or infection affecting the peripheral nerves, spinal cord, or brain. The resulting pain is often described as burning, shooting, or stabbing, and it may be accompanied by other sensory disturbances like tingling or numbness.
Conditions like diabetic neuropathy, postherpetic neuralgia (shingles), and nerve compression syndromes (e.g., carpal tunnel syndrome) are common causes of neuropathic pain. The underlying mechanisms involve aberrant signaling, increased excitability of neurons, and changes in the brain's pain processing centers.
Hyperalgesia and Allodynia: Heightened Sensitivity
Hyperalgesia refers to an increased sensitivity to pain. A stimulus that would normally cause mild discomfort now elicits intense pain. This can be a consequence of tissue damage, inflammation, or nerve injury, leading to sensitization of nociceptors and amplified pain signals.
Relatedly, allodynia is pain caused by a stimulus that is normally not painful. For example, light touch on the skin might trigger a sharp, burning sensation. Allodynia is often associated with neuropathic pain conditions and involves abnormal processing of sensory input in the central nervous system.
Phantom Limb Pain: A Ghostly Sensation
Phantom limb pain is a particularly intriguing phenomenon experienced by amputees. Despite the absence of a limb, individuals may continue to feel pain, tingling, or other sensations originating from the missing body part.
The exact mechanisms underlying phantom limb pain are not fully understood, but several theories exist. These theories involve the reorganization of the brain's sensory cortex, nerve damage at the amputation site, and psychological factors. Managing phantom limb pain can be challenging, often requiring a multidisciplinary approach.
Fibromyalgia: Widespread Pain and Sensory Amplification
Fibromyalgia is a chronic condition characterized by widespread musculoskeletal pain accompanied by fatigue, sleep disturbances, and cognitive difficulties. A key feature of fibromyalgia is central sensitization, where the brain amplifies pain signals, leading to heightened sensitivity to touch, pressure, and other stimuli.
The exact cause of fibromyalgia remains unknown, but genetic predisposition, environmental factors, and psychological stress are thought to play a role. Treatment typically involves a combination of medications, physical therapy, and lifestyle modifications.
Sensory Loss and Dysfunction: When Pathways are Blocked
Beyond pain disorders, somatosensory dysfunction can also manifest as a loss or alteration of sensation.
Anesthesia: The Absence of Feeling
Anesthesia, in the context of somatosensation, refers to a complete loss of sensation in a specific area of the body. This can result from nerve damage, spinal cord injury, or brain lesions. Anesthesia can affect touch, temperature, pain, and proprioception, depending on the extent and location of the damage.
The consequences of anesthesia can be significant, increasing the risk of injury, infection, and impaired motor control. Protective sensation is lost, making individuals susceptible to burns, pressure sores, and other hazards.
Paresthesia: The Tingling Intrusion
Paresthesia is an abnormal sensation, often described as tingling, prickling, or numbness. It can occur transiently, such as when a limb "falls asleep," or it can be a chronic symptom associated with nerve compression, nerve damage, or certain medical conditions.
Paresthesia can be caused by a variety of factors, including nerve entrapment, vitamin deficiencies, metabolic disorders, and multiple sclerosis. While paresthesia is not always painful, it can be bothersome and may indicate an underlying neurological problem.
Assessing Somatosensation: Tools and Techniques for Diagnosis
Having explored how the brain interprets sensory information, it is crucial to consider what happens when this intricate system malfunctions. The clinical aspects of somatosensation encompass a range of disorders, from debilitating pain conditions to profound sensory loss, significantly impacting an individual's quality of life. Accurate diagnosis and management of these conditions rely heavily on comprehensive assessment techniques that evaluate the integrity and function of the somatosensory system. A variety of tools are employed to determine whether the sensory pathways are intact.
Diagnostic Modalities for Somatosensory Evaluation
Several diagnostic modalities are used to assess somatosensation, each offering unique insights into the function of different components of the sensory system. These tools range from simple, bedside assessments to sophisticated electrophysiological and histological investigations. The selection of appropriate diagnostic tests depends on the patient's symptoms, the suspected underlying pathology, and the clinical context.
Von Frey Filaments: Mapping Tactile Perception
Von Frey filaments are a cornerstone in somatosensory assessment, particularly for evaluating tactile sensitivity and pain thresholds. These simple yet effective tools consist of a series of calibrated monofilaments of varying thicknesses, each designed to exert a specific force when applied perpendicularly to the skin.
During the assessment, the filament is pressed against the skin until it bends, delivering a defined mechanical stimulus. The patient is then asked to report whether they feel the stimulus or if it elicits a pain response. By systematically applying filaments of increasing force, clinicians can determine the threshold at which the patient perceives touch or pain.
This method is invaluable for identifying areas of reduced or heightened sensitivity, aiding in the diagnosis of conditions such as peripheral neuropathy, diabetic neuropathy, and other pain disorders. Von Frey filaments provide a quantitative and standardized measure of tactile and pain perception, making them an essential tool in somatosensory evaluation.
Quantitative Sensory Testing (QST): A Comprehensive Sensory Profile
Quantitative Sensory Testing (QST) represents a more comprehensive approach to somatosensory assessment, providing a detailed profile of sensory function across various modalities. QST employs specialized equipment to deliver precisely controlled stimuli, including thermal, mechanical, and vibratory stimuli.
These stimuli are presented in a standardized manner, and the patient's responses are recorded to determine sensory thresholds for each modality.
QST allows for the assessment of a wide range of sensory parameters, including:
- Thermal detection thresholds: The ability to detect warm and cold stimuli.
- Vibration detection thresholds: The sensitivity to vibratory stimuli.
- Mechanical detection thresholds: The ability to perceive light touch and pressure.
- Pain thresholds: The level of stimulus intensity at which pain is perceived.
By comparing a patient's sensory thresholds to normative data, clinicians can identify sensory deficits or abnormalities, providing valuable information for diagnosis and treatment planning. QST is particularly useful in characterizing complex pain syndromes and identifying subtle sensory changes that may not be detected by routine clinical examination.
Electrophysiological Studies: Assessing Nerve and Muscle Function
Nerve Conduction Studies (NCS): Tracing Neural Pathways
Nerve Conduction Studies (NCS) are electrophysiological tests that evaluate the function of peripheral nerves by measuring the speed and amplitude of electrical signals as they travel along a nerve. During an NCS, electrodes are placed on the skin over a nerve, and electrical stimuli are delivered to the nerve.
The resulting electrical activity is recorded at another location along the nerve, allowing clinicians to calculate the nerve conduction velocity and amplitude of the signal.
Reduced nerve conduction velocity or amplitude can indicate nerve damage or dysfunction, such as in cases of peripheral neuropathy, carpal tunnel syndrome, or nerve entrapment. NCS are essential for localizing nerve lesions and determining the severity of nerve damage, guiding treatment decisions and monitoring disease progression.
Electromyography (EMG): Evaluating Muscle Response
Electromyography (EMG) is another electrophysiological technique used to assess the electrical activity of muscles. EMG involves inserting a needle electrode into a muscle to record its electrical activity at rest and during contraction.
The patterns of electrical activity can reveal information about muscle function, nerve supply to the muscle, and the presence of muscle disorders. EMG is often performed in conjunction with NCS to provide a comprehensive assessment of the neuromuscular system.
EMG can help differentiate between nerve and muscle disorders, identify specific muscle involvement in nerve injuries, and assess the severity of muscle damage or weakness.
Skin Biopsy: A Microscopic View of Nerve Endings
Skin biopsy is an invasive diagnostic procedure that involves removing a small sample of skin for microscopic examination. In the context of somatosensory assessment, skin biopsies are primarily used to evaluate the density and morphology of nerve fibers in the skin, particularly small-diameter nerve fibers involved in pain and temperature sensation.
Skin biopsies are especially valuable in diagnosing small fiber neuropathy, a condition characterized by damage to small nerve fibers that can cause chronic pain, burning sensations, and other sensory disturbances. By quantifying the number and appearance of nerve fibers in the skin, clinicians can confirm the presence of small fiber neuropathy and assess its severity.
Integrating Assessment Tools for Comprehensive Diagnosis
Effective assessment of somatosensation often requires a combination of different diagnostic tools and techniques. By integrating the information obtained from clinical examination, Von Frey filaments, QST, electrophysiological studies, and skin biopsies, clinicians can develop a comprehensive understanding of the patient's sensory deficits or abnormalities.
This integrated approach allows for accurate diagnosis, targeted treatment planning, and effective management of somatosensory disorders. The precise and targeted assessment of a patient's somatosensory system is paramount to properly managing and restoring balance in their lives.
Restoring Balance: Modulation and Treatment of Somatosensory Disorders
Having assessed the methods for diagnosing somatosensory dysfunctions, the focus shifts to the therapeutic interventions designed to restore balance. Modulation and treatment strategies for somatosensory disorders are diverse, reflecting the complexity of the system itself. Effective management often requires a multimodal approach, integrating pharmacological and non-pharmacological techniques. This section delves into the nuances of these interventions, emphasizing pain management and sensory retraining.
Pharmacological Strategies
Pharmacological interventions form a cornerstone of somatosensory disorder management. The selection of appropriate medication is contingent on the underlying cause and specific symptoms.
Topical Anesthetics
Topical anesthetics, such as lidocaine and benzocaine, provide localized pain relief by blocking nerve signals in the immediate area of application. These agents are particularly useful for managing superficial pain conditions, like postherpetic neuralgia or minor skin irritations. Their efficacy is limited by their inability to penetrate deeply into tissues.
Systemic Pain Medications
Systemic pain medications offer a broader approach to pain management. This category includes:
-
Analgesics: Such as acetaminophen and nonsteroidal anti-inflammatory drugs (NSAIDs), address mild to moderate pain by reducing inflammation and inhibiting prostaglandin synthesis.
-
Opioids: Reserved for severe pain, opioids act on opioid receptors in the central nervous system. They modulate pain perception, providing potent analgesia. However, their use is carefully considered due to the risk of dependence and adverse side effects.
-
Neuropathic Pain Medications: These medications, including gabapentinoids (gabapentin, pregabalin) and certain antidepressants (e.g., tricyclic antidepressants, SNRIs), target the underlying mechanisms of neuropathic pain. They modulate neuronal excitability and neurotransmitter levels.
Non-Pharmacological Interventions
Non-pharmacological interventions offer valuable alternatives or adjuncts to medication. They address the physical and psychological aspects of somatosensory disorders.
Physical Therapy
Physical therapy plays a critical role in pain management and sensory retraining. Tailored exercise programs, manual therapy techniques, and modalities like heat or cold therapy can reduce pain, improve function, and restore normal movement patterns.
Sensory retraining techniques, such as desensitization exercises, help patients adapt to altered sensory input and reduce pain hypersensitivity. These strategies are particularly beneficial for conditions like complex regional pain syndrome.
Alternative and Complementary Therapies
A range of other therapies are employed to manage somatosensory disorders. These include:
-
Transcutaneous Electrical Nerve Stimulation (TENS): This involves applying mild electrical currents to the skin to stimulate nerve fibers, thereby reducing pain signals.
-
Acupuncture: This traditional Chinese medicine technique involves inserting thin needles into specific points on the body. It aims to modulate pain pathways and promote healing.
-
Cognitive-Behavioral Therapy (CBT): CBT addresses the psychological aspects of chronic pain by helping patients develop coping strategies, manage stress, and alter maladaptive thought patterns.
The holistic approach of non-pharmacological interventions complements pharmacological strategies. They improve overall well-being and quality of life.
The Inflammatory Response: How Inflammation Affects Sensation
Having assessed the methods for diagnosing somatosensory dysfunctions, the focus shifts to the therapeutic interventions designed to restore balance. Modulation and treatment strategies for somatosensory disorders are diverse, reflecting the complexity of the system itself. Effective management hinges on a thorough understanding of how inflammation, a fundamental biological response, intricately modulates sensory perception.
The body's inflammatory response, a complex cascade of biological events, is initiated in response to tissue injury, infection, or irritation. This response, while essential for healing and defense, profoundly impacts sensory nerve endings, leading to altered sensations and heightened pain sensitivity. This intricate relationship warrants closer examination to fully appreciate its role in both acute and chronic pain conditions.
Inflammation's Cascade: A Brief Overview
Inflammation is characterized by the release of various inflammatory mediators. These include cytokines, chemokines, and growth factors, which act to recruit immune cells to the site of injury.
These mediators also directly interact with sensory neurons. This interaction causes the neurons to become more excitable, a process known as peripheral sensitization.
Peripheral Sensitization: Amplifying the Pain Signal
Peripheral sensitization is a key mechanism by which inflammation increases sensitivity to pain. Inflammatory mediators bind to receptors on nociceptors, the sensory neurons responsible for detecting painful stimuli.
This binding leads to a reduction in the threshold required to activate these neurons. As a result, stimuli that would normally be perceived as innocuous are now interpreted as painful. This phenomenon, known as allodynia, is a hallmark of inflammatory pain.
Hyperalgesia, an increased sensitivity to painful stimuli, also arises from peripheral sensitization. The inflammatory milieu enhances the response of nociceptors to noxious stimuli. This results in an exaggerated pain sensation.
The Role of Specific Inflammatory Mediators
Prostaglandins: Key Players in Pain Amplification
Prostaglandins, synthesized via the cyclooxygenase (COX) pathway, are potent pain-enhancing molecules. They directly sensitize nociceptors and contribute to the inflammatory cascade. Non-steroidal anti-inflammatory drugs (NSAIDs) exert their analgesic effects by inhibiting COX enzymes.
Cytokines: Orchestrating the Inflammatory Response
Cytokines, such as interleukin-1β (IL-1β) and tumor necrosis factor-α (TNF-α), play a crucial role in the inflammatory response. These cytokines amplify inflammation by recruiting immune cells and promoting the release of other inflammatory mediators.
They also directly sensitize nociceptors, contributing to both allodynia and hyperalgesia. Targeting cytokines has become a focus for treating chronic inflammatory pain conditions.
Nerve Growth Factor (NGF): Promoting Neuronal Plasticity and Pain
Nerve Growth Factor (NGF), is a key player in neuronal survival and development, which is upregulated during inflammation. Elevated NGF levels can lead to neuronal sprouting and increased nociceptor excitability. This contributes to the chronification of pain.
Implications for Chronic Pain
The acute inflammatory response, normally a self-limiting process, can transition into a chronic state. The persistent release of inflammatory mediators leads to long-lasting sensitization of sensory neurons. This leads to chronic pain conditions such as arthritis, neuropathic pain, and inflammatory bowel disease. Understanding the specific inflammatory pathways involved in these conditions is crucial for developing targeted therapies.
Pioneers of Perception: Researchers Who Shaped Our Understanding
Having assessed the methods for diagnosing somatosensory dysfunctions, the focus shifts to the therapeutic interventions designed to restore balance. Modulation and treatment strategies for somatosensory disorders are diverse, reflecting the complexity of the system itself. Effective management hinges on a deep understanding of the individuals who laid the groundwork for our current knowledge. This section highlights some key researchers and their invaluable contributions to the field of somatosensation.
Foundations Laid: Early Contributors to Somatosensory Science
The understanding of somatosensation, like any scientific field, is built upon the contributions of numerous researchers, each adding a piece to the puzzle. Recognizing their work provides context and appreciation for the current state of knowledge.
Max von Frey: Quantifying Touch and Pain
Perhaps one of the most recognizable names in somatosensory research is Max von Frey. His development of the von Frey filaments revolutionized the study of touch and pain perception.
These simple yet elegant tools, consisting of calibrated filaments of varying stiffness, allowed researchers to apply controlled mechanical stimuli to the skin and objectively assess sensory thresholds.
Von Frey's work provided a standardized method for quantifying tactile sensitivity and pain thresholds, paving the way for countless studies on sensory function and dysfunction. His contribution remains a cornerstone in clinical sensory testing.
Beyond Von Frey: Expanding the Sensory Landscape
While von Frey's work stands as a monumental achievement, many other researchers have significantly contributed to our understanding of somatosensation. Identifying them is crucial to painting a comprehensive picture of the development of this field.
Vernon Mountcastle: Mapping the Somatosensory Cortex
Vernon Mountcastle made landmark contributions to understanding the organization of the somatosensory cortex. Through meticulous electrophysiological studies, he demonstrated that the cortex is organized into columnar units, each responding to a specific type of sensory input.
Mountcastle's work revealed the modular architecture of the cortex and provided insights into how sensory information is processed and represented in the brain. His findings have had a profound impact on our understanding of cortical function in general.
Patrick Wall and Ronald Melzack: The Gate Control Theory of Pain
Patrick Wall and Ronald Melzack revolutionized the understanding of pain with their Gate Control Theory. This groundbreaking theory proposed that pain perception is not simply a direct transmission of nociceptive signals from the periphery to the brain.
Rather, it is modulated by a "gate" in the spinal cord, which can be influenced by both peripheral and central factors. The Gate Control Theory helped explain phenomena such as the placebo effect and the effectiveness of non-pharmacological pain management strategies. It shifted the focus from a purely biomedical model of pain to a more biopsychosocial approach.
A Legacy of Discovery
These pioneers, along with many others, have shaped our current understanding of somatosensation. Their discoveries have not only advanced scientific knowledge, but have also led to improved diagnostic tools and treatment strategies for individuals suffering from sensory disorders.
Recognizing their contributions is essential for appreciating the complexity and richness of the field. As research continues, future generations of scientists will undoubtedly build upon their legacy, pushing the boundaries of our understanding even further.
Video: Nerve Endings in Skin: Sensitivity & Function
FAQs: Nerve Endings in Skin: Sensitivity & Function
What are the main types of nerve endings in skin and what do they sense?
The main types are mechanoreceptors (touch, pressure), thermoreceptors (temperature), nociceptors (pain), and proprioceptors (body position). Each type of nerve ending in skin is specialized to detect a specific sensation.
How do nerve endings in skin allow us to feel different textures?
Different textures activate various mechanoreceptors within our skin. For example, Meissner's corpuscles are sensitive to light touch, while Pacinian corpuscles respond to deep pressure and vibrations. The brain interprets the combination of signals from these nerve endings in skin to perceive texture.
Why are some areas of the body more sensitive than others?
Sensitivity varies depending on the concentration of nerve endings in skin. Areas like fingertips and lips have a higher density of nerve endings, making them more sensitive to touch and other stimuli compared to areas like the back.
What happens if nerve endings in skin are damaged?
Damage to nerve endings in skin can result in a range of issues. This can include numbness, tingling, increased sensitivity, or chronic pain, depending on the type and extent of the damage to the nerve endings in skin.
So, the next time you feel a gentle breeze or a painful prick, remember the incredible network of nerve endings in skin working tirelessly to keep you informed and safe. It's a pretty amazing system, isn't it?