Minute Volume: The Ultimate Guide You Need Right Now
Understanding minute volume is critical for assessing respiratory function, a cornerstone of clinical evaluation championed by organizations like the American Thoracic Society. Accurate measurement of minute volume, often achieved using a spirometer, helps clinicians understand the quantity of gas moving in and out of the lungs. Conditions affecting tidal volume, a significant component determining minute volume, can reveal underlying pulmonary disorders. Therefore, proper assessment of minute volume, which often requires the clinical expertise of a pulmonologist, is invaluable for effective patient care and understanding the efficacy of various respiratory therapies.
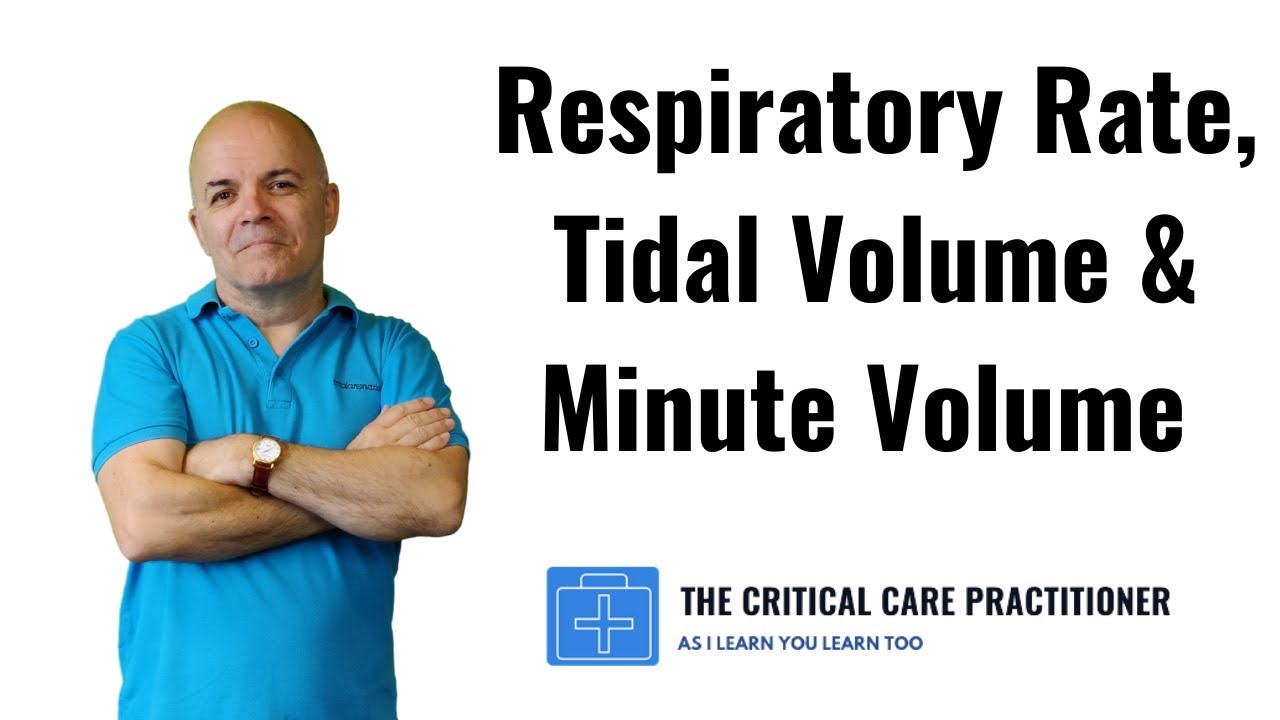
Image taken from the YouTube channel The Critical Care Practitioner , from the video titled Respiratory Rate, Tidal Volume and Minute Volume .
Minute volume (MV), a cornerstone of respiratory physiology, represents the total volume of air inhaled or exhaled per minute. It's a fundamental measurement that reflects the efficiency of ventilation. Understanding minute volume is not merely an academic exercise. It's a crucial skill for healthcare professionals. It's also valuable for anyone seeking to understand their own respiratory health, or the respiratory health of those around them.
Defining Minute Volume
Minute volume is specifically defined as the amount of gas either inhaled or exhaled from a person's lungs within a one-minute interval.
This volume reflects the combined effect of each individual breath and the frequency of those breaths. It’s a dynamic measurement that can change rapidly in response to various physiological demands and pathological conditions.
The Significance of Minute Volume in Respiratory Assessment
Minute volume serves as a vital sign for assessing respiratory function. Deviations from the normal range can indicate underlying respiratory problems, even before other symptoms become apparent.
By monitoring MV, clinicians can gain valuable insights into a patient's ability to effectively ventilate. It helps clinicians detect subtle changes that might signal the onset of respiratory distress. Minute volume is particularly valuable in the early detection of respiratory compromise.
Key Determinants: Tidal Volume and Respiratory Rate
Minute volume is determined by two primary factors:
- Tidal Volume: The volume of air inhaled or exhaled with each breath.
- Respiratory Rate: The number of breaths taken per minute.
Both tidal volume and respiratory rate contribute to the overall minute volume. Understanding the interplay between these two components is essential for interpreting MV values. Changes in either tidal volume or respiratory rate directly impact the minute volume, affecting the body's ability to maintain adequate gas exchange.
Goal of This Guide
This guide aims to provide a comprehensive understanding of minute volume. We will explore its determinants, its clinical significance, and how it is measured. By the end of this guide, you will have a solid foundation in the principles of minute volume and its application in respiratory health assessment. You will also gain insights into how to recognize and interpret changes in minute volume.
Minute volume provides a global overview of ventilation, but to truly understand its significance, we must delve into its fundamental components. Like dissecting a complex equation to understand its variables, examining tidal volume and respiratory rate gives crucial insights into the mechanics of breathing. Understanding these "building blocks" is essential to appreciate the dynamic nature of minute volume and its implications for respiratory health.
The Building Blocks: Tidal Volume and Respiratory Rate
Minute volume doesn't operate in isolation; it's the product of two key elements: tidal volume and respiratory rate. Each contributes uniquely to the overall effectiveness of ventilation. Understanding them individually, and how they interact, is essential for interpreting minute volume effectively.
Decoding Tidal Volume (TV)
Tidal volume (TV) is defined as the volume of air inhaled or exhaled during a single, normal breath at rest. It represents the "size" of each breath. It's a dynamic measurement influenced by a number of factors.
Factors Influencing Tidal Volume
Several factors affect a person's tidal volume. Body size plays a significant role. Larger individuals generally have larger lung capacities and, consequently, larger tidal volumes.
Activity level also impacts TV. During exercise, the body requires more oxygen. Tidal volume increases to meet this demand.
Other factors include:
- Posture
- Lung compliance
- Airway resistance
- Overall respiratory muscle strength
Normal Ranges for Tidal Volume
In healthy adults, normal tidal volume typically falls within the range of 6-8 mL/kg of ideal body weight. This means a person weighing 70 kg (approximately 154 lbs) would have a normal tidal volume of roughly 420-560 mL. However, it's crucial to remember that this is just a guideline. Individual variations exist. Clinical context always trumps textbook values.
Understanding Respiratory Rate (RR)
Respiratory Rate (RR), also known as breathing frequency, is the number of breaths a person takes per minute. It reflects how frequently the lungs are being filled and emptied.
Factors Influencing Respiratory Rate
Respiratory rate is far from a fixed value. Many physiological and psychological factors can cause it to fluctuate.
Anxiety and stress often lead to an increased respiratory rate. This is due to the body's "fight or flight" response.
Pain can also trigger a faster breathing rate as the body attempts to compensate for discomfort.
Exercise, as with tidal volume, significantly elevates respiratory rate to meet the increased oxygen demands of the muscles.
Other influences include:
- Body Temperature
- Medications
- Underlying medical conditions
Normal Ranges for Respiratory Rate
The normal respiratory rate for adults at rest is typically between 12 and 20 breaths per minute. Outside this range may indicate an underlying respiratory issue. Again, clinical context is critical. Trained athletes, for example, may have resting respiratory rates below 12 breaths per minute.
The Interplay: TV, RR, and Minute Volume
The relationship between tidal volume, respiratory rate, and minute volume is fundamental. Minute volume is the product of tidal volume and respiratory rate.
Minute Volume (MV) = Tidal Volume (TV) x Respiratory Rate (RR)
This equation highlights a crucial point: A change in either tidal volume or respiratory rate will directly impact minute volume.
For example: If a person's tidal volume decreases (perhaps due to restrictive lung disease), their body might compensate by increasing respiratory rate to maintain an adequate minute volume. Conversely, if someone has a slow respiratory rate, their tidal volume might increase to ensure sufficient ventilation. Understanding this dynamic interplay is crucial for interpreting minute volume in clinical settings.
Minute volume provides a global overview of ventilation, but to truly understand its significance, we must delve into its fundamental components. Like dissecting a complex equation to understand its variables, examining tidal volume and respiratory rate gives crucial insights into the mechanics of breathing. Understanding these "building blocks" is essential to appreciate the dynamic nature of minute volume and its implications for respiratory health.
The MV Formula: Calculating Minute Volume
Now that we have a solid grasp of tidal volume and respiratory rate and how they influence ventilation, we can explore how these factors combine to form minute volume. Understanding how to calculate minute volume is a cornerstone of respiratory assessment, providing a quantifiable measure of ventilatory performance. This simple calculation can unlock a wealth of information about a patient's respiratory status and the effectiveness of their breathing.
The Formula Unveiled
The formula for calculating minute volume is straightforward:
Minute Volume (MV) = Tidal Volume (TV) x Respiratory Rate (RR)
This deceptively simple equation is the foundation for understanding how much air is moving in and out of the lungs each minute. Let's break down each component and its role in the calculation.
Units of Measurement: Ensuring Accuracy
Accuracy requires standardization, so it's crucial to express these values in the correct units:
- Tidal Volume (TV) is typically measured in milliliters (mL) or liters (L).
- Respiratory Rate (RR) is measured in breaths per minute (breaths/min).
- Minute Volume (MV) is therefore expressed in liters per minute (L/min). If TV is measured in mL, the result must be divided by 1000 to express MV in L/min.
Using the correct units ensures that the final calculation is accurate and clinically relevant. Consistency is paramount.
Real-World Application: Example Calculations
Let's put the formula into practice with several examples. These examples will illustrate how changes in either tidal volume or respiratory rate affect the overall minute volume.
Scenario 1: Normal Ventilation
A patient has a tidal volume of 500 mL (0.5 L) and a respiratory rate of 12 breaths/min.
MV = 0.5 L x 12 breaths/min = 6 L/min
This value falls within the normal range for minute volume in adults.
Scenario 2: Hypoventilation
A patient with a drug overdose has a tidal volume of 300 mL (0.3 L) and a respiratory rate of 8 breaths/min.
MV = 0.3 L x 8 breaths/min = 2.4 L/min
This significantly reduced minute volume indicates hypoventilation, which could lead to hypercapnia and hypoxia.
Scenario 3: Hyperventilation
A patient experiencing an anxiety attack has a tidal volume of 600 mL (0.6 L) and a respiratory rate of 20 breaths/min.
MV = 0.6 L x 20 breaths/min = 12 L/min
This elevated minute volume suggests hyperventilation. While it can temporarily increase oxygen delivery, it can also lead to hypocapnia and other complications.
Clinical Relevance: Monitoring and Intervention
The calculation of minute volume extends beyond simple arithmetic; it serves as a critical tool for monitoring patients in various clinical settings. In mechanically ventilated patients, for example, monitoring the minute volume allows clinicians to fine-tune ventilator settings to ensure adequate ventilation.
Adjusting tidal volume or respiratory rate on the ventilator directly impacts the patient's minute volume. This allows for precise control over gas exchange.
Furthermore, monitoring changes in minute volume can alert clinicians to potential problems, such as declining respiratory function. It guides timely interventions to prevent respiratory distress.
Beyond Minute Volume: Alveolar Ventilation and Dead Space
While minute volume provides a snapshot of overall ventilation, it doesn't tell the whole story. To truly assess respiratory effectiveness, we must consider where that air is going and whether it's actively participating in gas exchange. That's where the concepts of alveolar ventilation and dead space ventilation come into play. Understanding these concepts allows for a more nuanced interpretation of minute volume and its impact on oxygenation and carbon dioxide removal.
Alveolar Ventilation: Where Gas Exchange Happens
Alveolar ventilation refers to the volume of fresh gas that reaches the alveoli—the tiny air sacs in the lungs—per minute and is available for gas exchange. It represents the effective ventilation, as it's the portion of inspired air that directly contributes to oxygenating the blood and removing carbon dioxide.
Effective alveolar ventilation ensures the efficient transfer of oxygen into the bloodstream and carbon dioxide out of it. Several factors affect its effectiveness. For example, conditions that reduce lung surface area or impair alveolar function (such as emphysema or pneumonia) will reduce alveolar ventilation even if minute volume remains adequate.
Dead Space Ventilation: Air That Goes Nowhere
In contrast to alveolar ventilation, dead space ventilation is the volume of gas that enters the respiratory system but does not participate in gas exchange.
This volume ventilates the conducting airways (trachea, bronchi, bronchioles). These areas don't have alveoli. Therefore, they don't facilitate gas exchange.
Anatomical dead space refers to the volume of the conducting airways, which is relatively constant. Alveolar dead space, on the other hand, refers to alveoli that are ventilated but not perfused with blood, rendering them ineffective for gas exchange. Conditions like pulmonary embolism can increase alveolar dead space.
The Interplay: MV, Alveolar Ventilation, and Dead Space
Minute volume represents the total volume of air moved in and out of the lungs per minute, but it's the sum of both alveolar and dead space ventilation. The relationship can be expressed as follows:
MV = Alveolar Ventilation + Dead Space Ventilation
This equation highlights a critical point: a normal minute volume does not guarantee adequate alveolar ventilation. If dead space ventilation is significantly increased, a substantial portion of the minute volume is wasted, leading to reduced efficiency of gas exchange.
The Impact of Increased Dead Space
Increased dead space ventilation reduces the efficiency of gas exchange, leading to a need for higher minute ventilation to maintain adequate alveolar ventilation.
Imagine a scenario where a patient has a normal minute volume, but a large portion of it is directed to dead space. In such cases, the amount of fresh gas reaching the alveoli is reduced, leading to hypoxemia (low blood oxygen) and hypercapnia (high blood carbon dioxide).
Strategies to reduce dead space or improve alveolar ventilation are crucial for optimizing respiratory function in these situations. This may involve interventions such as bronchodilators to reduce airway resistance, mechanical ventilation to increase tidal volume, or treatments to address underlying conditions causing alveolar dead space.
Clinical Significance: Minute Volume in Health and Disease
Having explored the fundamental components of minute volume and its relationship to alveolar ventilation and dead space, it's time to examine its clinical relevance. Minute volume is not merely a number; it's a vital sign that reflects the effectiveness of ventilation and can provide critical insights into a patient's respiratory status. Deviations from the normal range can signal underlying health issues, and understanding these implications is crucial for effective clinical decision-making.
Decoding Abnormal Minute Volume Values
Minute volume (MV), when outside the normal physiological range, serves as a red flag. Interpreting whether it's too low (hypoventilation) or too high (hyperventilation) is paramount.
Hypoventilation: When Breathing is Insufficient
Hypoventilation, characterized by a low minute volume, signifies that the lungs aren't adequately removing carbon dioxide from the body. This can lead to a dangerous buildup of CO2 in the blood, a condition known as hypercapnia.
Concurrently, hypoventilation often results in hypoxia, a deficiency in oxygen reaching the tissues.
Several factors can contribute to hypoventilation. Drug overdoses, particularly with opioids or sedatives, can depress the respiratory center in the brain, slowing breathing and reducing tidal volume.
Neuromuscular disorders, such as muscular dystrophy or amyotrophic lateral sclerosis (ALS), can weaken respiratory muscles, impairing their ability to generate sufficient inspiratory force.
Obesity hypoventilation syndrome (OHS) is another cause, where excess weight on the chest wall reduces lung expansion and respiratory drive regulation.
The consequences of untreated hypoventilation can be severe. Hypercapnia can lead to respiratory acidosis, a dangerous acid-base imbalance. Hypoxia can damage vital organs, including the brain and heart, potentially leading to long-term complications or even death.
Hyperventilation: Breathing Excessively
Hyperventilation, or high minute volume, indicates that the lungs are expelling carbon dioxide too rapidly. This leads to a decrease in blood CO2 levels, a condition called hypocapnia.
While seemingly beneficial, hyperventilation can have its own set of adverse effects. Anxiety and panic attacks are common culprits, as they trigger the sympathetic nervous system, leading to rapid, shallow breaths.
Pain can also stimulate hyperventilation, as the body attempts to compensate for perceived distress. Metabolic acidosis, a condition where the body produces too much acid, can trigger hyperventilation as the lungs try to expel excess CO2 to restore acid-base balance.
The primary consequence of hyperventilation is hypocapnia, which can cause a variety of symptoms. These include dizziness, lightheadedness, tingling sensations, and even muscle spasms.
In severe cases, hypocapnia can lead to cerebral vasoconstriction, reducing blood flow to the brain and potentially causing loss of consciousness.
Minute Volume in Specific Disease States
Minute volume assessment is invaluable in a wide array of respiratory and systemic diseases. Changes in MV often reflect the severity of the underlying condition and the effectiveness of treatment.
Asthma and COPD: The Impact of Airflow Obstruction
Asthma and chronic obstructive pulmonary disease (COPD) are characterized by airflow obstruction, making it difficult to exhale fully. In asthma, inflammation and bronchospasm narrow the airways, while in COPD, structural damage to the lungs impairs airflow.
Initially, patients with asthma or COPD might maintain a normal minute volume by increasing their respiratory rate. However, as the disease progresses, they may struggle to maintain adequate ventilation, leading to decreased tidal volume and, ultimately, a reduced minute volume.
This hypoventilation contributes to CO2 retention and respiratory acidosis.
Pneumonia and ARDS: Lung Injury and Gas Exchange
Pneumonia and acute respiratory distress syndrome (ARDS) cause inflammation and fluid accumulation in the lungs, impairing gas exchange. The increased work of breathing and reduced lung compliance can lead to changes in minute volume.
In the early stages, patients may hyperventilate to compensate for the reduced oxygen levels in the blood. As the condition worsens, the lungs become stiffer and less efficient, leading to a decrease in tidal volume and subsequent hypoventilation.
This combination of impaired gas exchange and reduced minute volume can result in severe hypoxia and respiratory failure.
Neuromuscular Disorders: Respiratory Muscle Weakness
Neuromuscular disorders such as Guillain-Barré syndrome, myasthenia gravis, and spinal cord injuries can weaken the respiratory muscles, compromising their ability to generate adequate inspiratory and expiratory force.
This muscle weakness directly impacts minute volume by reducing both tidal volume and respiratory rate. Patients with neuromuscular disorders are at high risk for hypoventilation, leading to hypercapnia, hypoxia, and respiratory failure.
Regular monitoring of minute volume is crucial in these patients to detect early signs of respiratory compromise.
Heart Failure: Pulmonary Edema and Ventilation
Heart failure can lead to pulmonary edema, where fluid accumulates in the lungs, impairing gas exchange and increasing the work of breathing.
The presence of pulmonary edema reduces lung compliance, making it harder to inflate the lungs. Patients with heart failure may initially hyperventilate to compensate for the reduced oxygen levels.
However, as the edema worsens, the increased work of breathing can lead to respiratory muscle fatigue and a decrease in tidal volume, resulting in hypoventilation.
Minute Volume Monitoring in Critical Care
In critically ill patients, continuous monitoring of minute volume is essential for guiding respiratory support strategies. Minute volume is closely monitored in patients receiving mechanical ventilation.
Ventilator settings, such as tidal volume and respiratory rate, are adjusted to achieve a target minute volume that ensures adequate gas exchange.
Deviations from the target minute volume can indicate a variety of problems, such as changes in lung compliance, increased dead space ventilation, or patient-ventilator asynchrony.
By continuously monitoring minute volume, clinicians can promptly identify and address these issues, optimizing respiratory support and improving patient outcomes.
Having explored the critical implications of minute volume deviations in various disease states, the next logical step is to understand how this vital parameter is actually quantified. Accurate measurement of minute volume relies on specific tools and techniques, and understanding these methodologies is essential for interpreting the data they provide.
Measuring Minute Volume: Tools and Techniques
Minute volume, a cornerstone of respiratory assessment, isn't just a theoretical value; it's a measurable parameter that provides tangible insights into a patient's respiratory function. Several tools and techniques are available to measure MV, each with its own strengths and applications.
Spirometry: A Direct Measurement Approach
Spirometry stands as a foundational method for directly measuring minute volume. This non-invasive technique utilizes a device called a spirometer to assess lung function, including the quantification of inhaled and exhaled air volumes.
During spirometry, the patient breathes into a mouthpiece connected to the spirometer. The device then measures the volume of air exhaled with each breath (tidal volume) and the number of breaths per minute (respiratory rate).
By multiplying these two values, the spirometer calculates the patient's minute volume. Spirometry is valuable in evaluating patients with suspected or known respiratory conditions, such as asthma, COPD, and restrictive lung diseases.
Ventilators: Monitoring and Controlling MV in Mechanically Ventilated Patients
In the critical care setting, where patients require mechanical ventilation, ventilators themselves serve as sophisticated tools for both measuring and controlling minute volume. Modern ventilators are equipped with sensors that continuously monitor the patient's respiratory parameters, including tidal volume, respiratory rate, and, consequently, minute volume.
These ventilators not only display real-time MV values but also allow clinicians to set target MV ranges based on the patient's individual needs. The ability to precisely control MV is crucial in optimizing ventilation strategies, ensuring adequate gas exchange, and preventing ventilator-induced lung injury.
Various ventilation modes offer different approaches to MV management. For instance, volume-controlled ventilation delivers a set tidal volume with each breath, guaranteeing a minimum MV. Pressure-controlled ventilation, on the other hand, delivers a set pressure, and the resulting tidal volume (and thus MV) depends on the patient's lung mechanics.
Capnography: An Indirect Window into Ventilation
While not a direct measurement of minute volume, capnography offers valuable insights into ventilation and can indirectly reflect changes in MV. Capnography measures the concentration of carbon dioxide (CO2) in the exhaled breath, providing a continuous waveform that reflects the effectiveness of ventilation.
The end-tidal CO2 (ETCO2) value, the CO2 concentration at the end of exhalation, is closely related to the patient's arterial CO2 (PaCO2). In general, hypoventilation (low MV) leads to an increase in ETCO2 and PaCO2, while hyperventilation (high MV) leads to a decrease.
By monitoring the capnography waveform and ETCO2 values, clinicians can assess the adequacy of ventilation and identify potential problems, such as airway obstruction or changes in respiratory drive. Capnography is a valuable tool for monitoring patients during anesthesia, sedation, and critical care.
However, it's crucial to remember that capnography provides an indirect assessment of MV. Factors other than MV, such as changes in metabolism or cardiac output, can also affect ETCO2 values.
The Importance of Accuracy and Calibration
Regardless of the chosen method, accurate measurement of minute volume hinges on proper technique and meticulous equipment calibration. Spirometers, ventilators, and capnographs must be regularly calibrated according to the manufacturer's instructions to ensure reliable and valid results.
Clinicians must also be trained in the proper use of these devices and understand the potential sources of error. Patient cooperation is also essential for accurate spirometry measurements.
By adhering to these principles, healthcare professionals can confidently utilize these tools to gain valuable insights into their patients' respiratory status.
Having explored the critical implications of minute volume deviations in various disease states, the next logical step is to understand how this vital parameter is actually quantified. Accurate measurement of minute volume relies on specific tools and techniques, and understanding these methodologies is essential for interpreting the data they provide.
Interpreting the Results: Minute Volume and Arterial Blood Gases
Minute volume (MV) provides a snapshot of overall ventilation, but to fully understand its clinical significance, we must correlate it with arterial blood gas (ABG) analysis. ABGs offer a detailed picture of the gas exchange occurring in the lungs and the acid-base balance in the bloodstream. When viewed in conjunction, MV and ABGs provide clinicians with a comprehensive understanding of a patient's respiratory status.
The Inverse Relationship Between Minute Volume and PaCO2
One of the most direct relationships exists between minute volume and the partial pressure of carbon dioxide in arterial blood (PaCO2). PaCO2 is a direct reflection of the adequacy of alveolar ventilation.
In essence, if the lungs are effectively removing CO2, the PaCO2 will be within the normal range (typically 35-45 mmHg). Hyperventilation, characterized by a high MV, leads to increased CO2 removal, resulting in a decreased PaCO2 (hypocapnia).
Conversely, hypoventilation, characterized by a low MV, results in reduced CO2 removal, leading to an increased PaCO2 (hypercapnia).
The relationship isn't always linear due to buffering mechanisms in the body, and changes in metabolic rate (CO2 production) also influence PaCO2.
However, significant deviations in PaCO2 often warrant an investigation into minute volume adequacy.
Minute Volume and PaO2: A More Complex Relationship
The relationship between minute volume and the partial pressure of oxygen in arterial blood (PaO2) is more complex than that of PaCO2. While adequate minute volume is essential for maintaining adequate oxygenation, it is not the only determinant of PaO2.
Several other factors influence PaO2, including:
- Fraction of Inspired Oxygen (FiO2): The concentration of oxygen the patient is breathing.
- Diffusion Capacity: The ability of oxygen to move from the alveoli into the bloodstream.
- Ventilation-Perfusion (V/Q) Matching: The balance between airflow and blood flow in the lungs.
- Hemoglobin Concentration: The amount of hemoglobin available to carry oxygen.
Therefore, a patient may have an adequate minute volume but still exhibit a low PaO2 (hypoxemia) due to issues with diffusion, V/Q mismatch, or other factors.
For example, in cases of pulmonary edema or acute respiratory distress syndrome (ARDS), the diffusion of oxygen across the alveolar-capillary membrane is impaired, resulting in hypoxemia despite potentially adequate or even elevated minute volume.
ABG Analysis: Evaluating Ventilation and Acid-Base Balance
Arterial blood gas (ABG) analysis is an invaluable tool for assessing the effectiveness of ventilation and identifying acid-base imbalances. Beyond PaO2 and PaCO2, ABGs provide information on pH, bicarbonate (HCO3-), and base excess, which are all critical for understanding the patient's overall acid-base status.
By evaluating these parameters in conjunction with minute volume, clinicians can determine whether the patient's respiratory system is effectively maintaining homeostasis.
For instance, a patient with a low minute volume, elevated PaCO2, and low pH is likely experiencing respiratory acidosis due to inadequate ventilation.
Conversely, a patient with a high minute volume, low PaCO2, and high pH may be experiencing respiratory alkalosis due to excessive ventilation.
ABG analysis allows clinicians to differentiate between respiratory and metabolic causes of acid-base disturbances, guiding appropriate treatment strategies.
Examples of ABG Results in Patients with MV Abnormalities
To illustrate the clinical application of MV and ABG interpretation, consider the following examples:
- Hypercapnic Respiratory Failure due to Hypoventilation: A patient with a drug overdose presents with a respiratory rate of 8 breaths per minute and a tidal volume of 300 mL, resulting in a minute volume of 2.4 L/min (significantly below the normal range).
- ABG results reveal: pH 7.20, PaCO2 68 mmHg, PaO2 55 mmHg.
- Interpretation: The low MV is causing CO2 retention (hypercapnia) and hypoxemia, leading to respiratory acidosis.
- Anxiety-Induced Hyperventilation: A patient experiencing an anxiety attack presents with a respiratory rate of 30 breaths per minute and a tidal volume of 400 mL, resulting in a minute volume of 12 L/min (significantly above the normal range).
- ABG results reveal: pH 7.50, PaCO2 28 mmHg, PaO2 95 mmHg.
- Interpretation: The high MV is causing excessive CO2 removal (hypocapnia), leading to respiratory alkalosis. While PaO2 is adequate, prolonged hyperventilation can have adverse effects.
These examples highlight the importance of interpreting minute volume in the context of ABG results to accurately diagnose and manage respiratory conditions. Understanding this relationship is crucial for effective respiratory care.
Minute Volume and Respiratory Support: Ventilators and Oxygen Therapy
Having explored the critical implications of minute volume deviations in various disease states, the next logical step is to understand how this vital parameter is actually quantified. Accurate measurement of minute volume relies on specific tools and techniques, and understanding these methodologies is essential for interpreting the data they provide.
Respiratory support strategies, including mechanical ventilation and oxygen therapy, are cornerstones of treatment for patients with respiratory compromise. The effectiveness of these interventions is intrinsically linked to minute volume (MV) and its optimization. Understanding the interplay between these therapies and MV is crucial for clinicians aiming to improve patient outcomes.
Ventilator Management and Minute Volume Control
Mechanical ventilators are sophisticated devices designed to assist or replace a patient's spontaneous breathing. A key function of these machines is the precise control of minute volume, ensuring adequate alveolar ventilation.
Ventilator settings are meticulously adjusted to achieve a target MV based on the patient's individual needs, considering factors such as body weight, metabolic rate, and underlying disease.
Ventilation Modes and Their Influence on Minute Volume
Different ventilation modes offer varying degrees of control over MV.
-
Volume-controlled ventilation (VCV) guarantees a set tidal volume with each breath, ensuring a consistent MV if the respiratory rate remains constant. This mode is useful when a guaranteed minute ventilation is needed.
-
Pressure-controlled ventilation (PCV) delivers breaths at a set pressure, allowing the tidal volume (and consequently MV) to vary based on the patient's lung mechanics. MV can be more variable in PCV, which requires close monitoring.
-
Synchronized Intermittent Mandatory Ventilation (SIMV) combines mandatory breaths (either volume or pressure-controlled) with spontaneous breaths initiated by the patient. MV is influenced by both the ventilator settings and the patient's effort.
-
Pressure Support Ventilation (PSV) provides pressure augmentation during spontaneous inspiration. MV relies almost entirely on the patient’s respiratory drive and effort, with the pressure support assisting to reduce the work of breathing.
The choice of ventilation mode depends on the patient's condition and the goals of respiratory support. Regardless of the mode, careful monitoring of MV and arterial blood gases is essential to ensure effective ventilation and prevent complications.
Oxygen Therapy: Addressing Hypoxemia Beyond Minute Volume
While adequate minute volume is necessary for gas exchange, it doesn't guarantee adequate oxygenation. Oxygen therapy is frequently employed to increase the fraction of inspired oxygen (FiO2), thereby raising the partial pressure of oxygen in arterial blood (PaO2).
Oxygen therapy is crucial in patients with hypoxemia (low PaO2), even when MV is within the normal range. Conditions such as pneumonia, pulmonary edema, or acute respiratory distress syndrome (ARDS) can impair oxygen diffusion across the alveolar-capillary membrane, necessitating supplemental oxygen.
Different methods of oxygen delivery, such as nasal cannulas, masks, and high-flow nasal cannula (HFNC), are used to achieve desired oxygen saturation levels. The goal is to maintain adequate oxygenation while avoiding oxygen toxicity, which can occur with prolonged exposure to high FiO2 levels.
Monitoring Minute Volume to Optimize Respiratory Support
Continuous monitoring of minute volume is a critical component of respiratory management. It provides valuable information about the effectiveness of ventilation and helps guide adjustments to ventilator settings or oxygen therapy.
Deviations from the target MV can indicate a variety of problems, such as:
- Changes in lung mechanics (e.g., increased airway resistance or decreased lung compliance).
- Changes in the patient's metabolic rate or carbon dioxide production.
- Ventilator malfunction or circuit leaks.
- The patient’s level of respiratory drive.
By closely monitoring MV and correlating it with other clinical parameters (e.g., arterial blood gases, respiratory rate, work of breathing), clinicians can make informed decisions to optimize respiratory support and improve patient outcomes. The dynamic interplay between MV, ventilation strategies, and oxygenation should always be considered to provide individualized and appropriate respiratory care.
Case Studies: Applying Your Minute Volume Knowledge
The true value of understanding minute volume (MV) lies in its practical application. By examining real-world case studies, we can see how MV assessments inform clinical decision-making and contribute to improved patient outcomes. These examples illustrate the importance of considering MV alongside other clinical data for a comprehensive understanding of a patient's respiratory status.
Case Study 1: Acute Asthma Exacerbation
A 25-year-old male presents to the emergency department with a severe asthma exacerbation. He is visibly distressed, using accessory muscles to breathe, and complaining of significant shortness of breath.
Initial assessment reveals:
- Respiratory rate (RR): 30 breaths/min
- Tidal volume (TV): 0.3 L (300 mL)
- Calculated minute volume (MV): 9 L/min
- Oxygen saturation (SpO2): 88% on room air
- Arterial blood gas (ABG): pH 7.30, PaCO2 50 mmHg, PaO2 60 mmHg
Analysis
While the calculated MV appears within the lower end of the normal range, the ABG reveals hypercapnia (elevated PaCO2), indicating ineffective ventilation. The rapid respiratory rate and reduced tidal volume suggest that the patient is breathing shallowly and rapidly, leading to increased dead space ventilation and inadequate alveolar ventilation.
In this case, the elevated PaCO2 despite a seemingly adequate MV highlights the importance of considering alveolar ventilation and dead space. The asthma exacerbation is causing airway obstruction, leading to air trapping and reduced effective gas exchange.
Management and Outcome
The patient was treated with bronchodilators and corticosteroids to relieve airway obstruction. Supplemental oxygen was administered to improve oxygen saturation.
Continuous monitoring of MV and ABGs helped guide treatment. As the airway obstruction improved, the patient's tidal volume increased, PaCO2 decreased, and oxygen saturation improved.
This case illustrates that MV alone is not sufficient to assess respiratory function. It must be interpreted in conjunction with ABGs and other clinical findings.
Case Study 2: Opioid Overdose
A 60-year-old female is brought to the emergency department after being found unresponsive at home. Her respirations are slow and shallow.
Initial assessment reveals:
- Respiratory rate (RR): 8 breaths/min
- Tidal volume (TV): 0.4 L (400 mL)
- Calculated minute volume (MV): 3.2 L/min
- Oxygen saturation (SpO2): 75% on room air
- Arterial blood gas (ABG): pH 7.20, PaCO2 70 mmHg, PaO2 50 mmHg
Analysis
The patient's minute volume is significantly below normal, indicating hypoventilation. The ABG confirms respiratory acidosis with hypercapnia and hypoxemia. The clinical presentation strongly suggests opioid overdose, which depresses the respiratory center in the brain, leading to decreased respiratory rate and tidal volume.
Hypoventilation is the primary problem in this scenario. The reduced MV is directly responsible for the elevated PaCO2 and decreased PaO2.
Management and Outcome
The patient was immediately administered naloxone (an opioid antagonist) to reverse the effects of the opioid. Assisted ventilation with a bag-valve-mask was initiated to support her breathing.
Following naloxone administration, the patient's respiratory rate and tidal volume gradually increased, leading to an improvement in her minute volume, PaCO2, and PaO2. She was monitored closely in the intensive care unit.
This case underscores the critical importance of recognizing and treating hypoventilation. Prompt intervention to improve MV can be life-saving.
Case Study 3: Patient with COPD
A 70-year-old male with a long history of COPD is admitted to the hospital with an acute exacerbation. He is complaining of increased shortness of breath and cough.
Initial assessment reveals:
- Respiratory rate (RR): 22 breaths/min
- Tidal volume (TV): 0.5 L (500 mL)
- Calculated minute volume (MV): 11 L/min
- Oxygen saturation (SpO2): 90% on 2L nasal cannula
- Arterial blood gas (ABG): pH 7.35, PaCO2 55 mmHg, PaO2 65 mmHg
Analysis
The patient's MV is slightly elevated, likely due to increased respiratory effort to compensate for impaired gas exchange in the setting of COPD. Although his PaO2 is reasonable with supplemental oxygen, his PaCO2 is elevated, indicating chronic CO2 retention, a common finding in COPD.
While the MV is elevated, the effectiveness of ventilation is compromised by the underlying lung disease. Air trapping and inefficient gas exchange contribute to the elevated PaCO2.
Management and Outcome
The patient was treated with bronchodilators, corticosteroids, and antibiotics. Oxygen therapy was adjusted to maintain adequate oxygen saturation without suppressing his respiratory drive.
Close monitoring of MV and ABGs was crucial to guide ventilator management. The goal was to optimize ventilation and oxygenation while avoiding over-ventilation or respiratory muscle fatigue.
This case highlights the complexities of managing patients with chronic respiratory disease. MV assessment, in conjunction with ABGs and clinical assessment, is essential for tailoring treatment to the individual patient's needs.
The Importance of Context
These case studies demonstrate that interpreting minute volume requires careful consideration of the patient's clinical context. MV is just one piece of the puzzle.
Factors such as underlying disease, arterial blood gas results, and clinical presentation must all be considered to accurately assess a patient's respiratory status and guide appropriate treatment.
By integrating MV assessments into a comprehensive clinical evaluation, healthcare professionals can optimize respiratory care and improve patient outcomes.
Video: Minute Volume: The Ultimate Guide You Need Right Now
Frequently Asked Questions About Minute Volume
Here are some frequently asked questions to help you further understand minute volume and its significance.
What exactly is minute volume?
Minute volume, also known as pulmonary ventilation, represents the total volume of air you breathe in (or out) per minute. It's calculated by multiplying your tidal volume (the amount of air inhaled in one breath) by your respiratory rate (the number of breaths you take per minute).
Why is minute volume important?
Minute volume is a key indicator of respiratory function. It reflects how effectively your lungs are delivering oxygen to your blood and removing carbon dioxide. Changes in minute volume can signal underlying respiratory issues or the body's response to exercise or stress.
What's considered a normal minute volume?
A normal resting minute volume typically ranges from 5 to 8 liters per minute. However, this can vary depending on factors like age, sex, body size, and activity level. During exercise, minute volume can increase significantly to meet the body's increased oxygen demands.
How can I improve my minute volume?
While you can't directly control your minute volume, improving your overall respiratory health through regular exercise, proper breathing techniques, and avoiding smoking can positively impact it. Addressing any underlying respiratory conditions is also crucial.