Mass of Solute Meaning: Chemistry Guide
In chemistry, understanding the mass of solute meaning is fundamental for accurate calculations and predictions related to solutions. Molarity, a common unit of concentration in laboratories across the globe, depends directly on the mass of solute present in a solution. Titration experiments, often conducted using equipment such as a burette, require precise knowledge of the solute's mass to determine unknown concentrations of other substances. The International Union of Pure and Applied Chemistry (IUPAC) establishes standardized definitions and procedures for these measurements, ensuring consistency and reliability in scientific research.
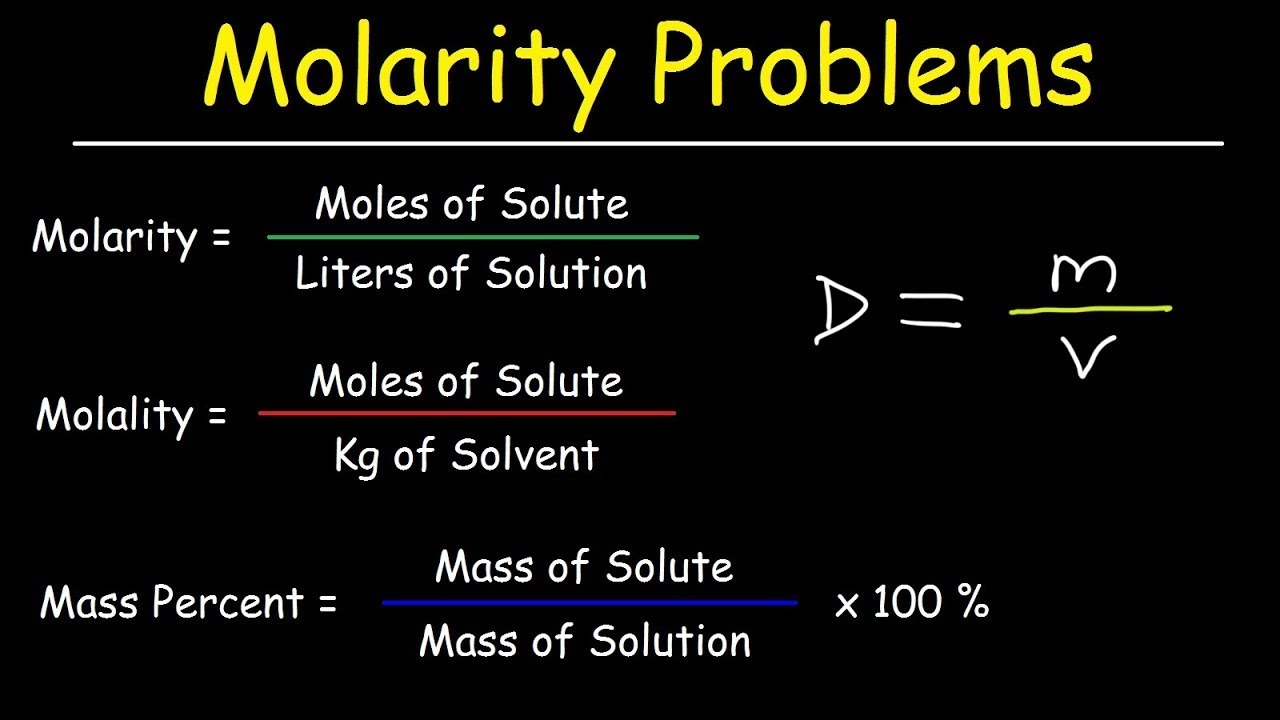
Image taken from the YouTube channel The Organic Chemistry Tutor , from the video titled Molarity, Molality, Volume & Mass Percent, Mole Fraction & Density - Solution Concentration Problems .
Solutions are fundamental to chemistry and permeate many facets of our lives, from the air we breathe to the medications we take. Understanding what constitutes a solution and how its components interact is crucial for grasping more advanced chemical concepts. This section lays the groundwork by defining solutions, explaining the roles of solutes and solvents, and illustrating the broad applicability of solution chemistry across various scientific and industrial domains.
Defining Solutions: Homogeneity is Key
At its core, a solution is a homogeneous mixture of two or more substances. This homogeneity implies that the mixture's composition is uniform throughout.
In other words, a sample taken from one part of the solution will have the same ratio of components as a sample taken from any other part.
This uniform distribution is what distinguishes solutions from heterogeneous mixtures, where the composition varies from point to point. Examples of homogeneous mixtures include saltwater and sugar dissolved in water.
The Players: Solute and Solvent
Two primary components define a solution: the solute and the solvent. The solute is the substance being dissolved. It can be a solid, liquid, or gas. Sugar dissolving in water is an example of a solid solute.
The solvent is the substance doing the dissolving, typically present in a larger amount than the solute. Water is often called the "universal solvent" because of its ability to dissolve a wide range of substances.
The interaction between the solute and solvent molecules is critical to the formation of a solution. Solvents interact with solutes to pull them apart and disperse them.
The Far-Reaching Impact of Solutions
Solutions are not confined to chemistry labs; they are ubiquitous across a multitude of disciplines.
In biology, bodily fluids like blood plasma are complex solutions that transport nutrients and waste products.
Medicine relies heavily on solutions for drug delivery, intravenous fluids, and diagnostic reagents.
In the industrial sector, solutions are used in various processes, including manufacturing, chemical synthesis, and materials processing.
Even everyday life is filled with examples of solutions, such as the air we breathe (a solution of gases) and the beverages we consume. A deep understanding of solutions is vital in science, technology, and daily life.
Unpacking the Components: Solute, Solvent, and Solution Types
Solutions are more than just a simple mix of substances; they are carefully orchestrated interactions between individual components. Understanding the roles of the solute and solvent is paramount to comprehending solution behavior. Furthermore, characterizing solutions based on the amount of solute they contain reveals key properties relevant to chemical processes and applications.
Defining the Solute: The Dissolved Component
The solute is the substance that is dispersed within the solvent to form a solution. It's the component that undergoes dissolution.
The amount of solute present is a critical factor in determining the solution's properties and behavior. Typically, the quantity of solute is measured in units of mass, such as grams (g) or kilograms (kg), especially when dealing with solid solutes.
However, for chemical reactions and stoichiometric calculations, expressing the amount of solute in moles (mol) is often more convenient. The molar mass of the solute can be used to convert between mass and moles.
The Solvent's Crucial Role
The solvent is the medium in which the solute dissolves. It's the component present in greater quantity (usually, but not always) and is responsible for dispersing the solute molecules or ions.
The solvent's properties, such as its polarity and intermolecular forces, play a critical role in its ability to dissolve a particular solute. For instance, polar solvents like water are effective at dissolving polar solutes, while nonpolar solvents like hexane are better suited for dissolving nonpolar solutes. This is because "like dissolves like."
The solvent interacts with the solute at the molecular level, breaking apart the solute's structure and surrounding the individual solute particles. This process, known as solvation, stabilizes the solute in the solution.
Types of Solutions Based on Solute Concentration
Solutions can be further classified based on the amount of solute dissolved relative to the solvent's capacity at a specific temperature. This gives rise to three primary categories: saturated, unsaturated, and supersaturated solutions.
Saturated Solutions: The Limit of Dissolution
A saturated solution contains the maximum amount of solute that can dissolve in a given amount of solvent at a specific temperature. At this point, the rate of dissolution is equal to the rate of precipitation, establishing a dynamic equilibrium.
Adding more solute to a saturated solution will not result in further dissolution; instead, the excess solute will remain undissolved as a solid precipitate.
Unsaturated Solutions: Room for More
An unsaturated solution contains less than the maximum amount of solute that could potentially dissolve in the solvent at the given temperature. This means that more solute can be added and dissolved without exceeding the solvent's capacity.
Supersaturated Solutions: Beyond the Limit
A supersaturated solution contains more than the maximum amount of solute that would normally dissolve in the solvent at that temperature. This is an unstable state that can be achieved by carefully cooling a saturated solution or by other specialized techniques.
Supersaturated solutions are metastable, meaning they can be easily triggered to precipitate the excess solute. A small disturbance, such as adding a seed crystal or scratching the container, can initiate rapid crystallization, returning the solution to a saturated state.
Quantifying Concentration: Measuring the Strength of a Solution
Concentration is the linchpin that connects the amount of solute to the overall properties of a solution. It is a quantitative expression of the amount of solute present in a defined amount of solution.
Expressing concentration accurately and precisely is vital for ensuring the reliability and reproducibility of experimental results, particularly in chemistry and related scientific fields.
Inaccurate measurements can lead to flawed conclusions and failed applications, particularly in sensitive fields such as medicine, pharmaceuticals, and manufacturing, where consistency and precision are paramount.
Why Accurate Concentration Matters
The ability to accurately quantify concentration is more than just an academic exercise; it's a cornerstone of scientific integrity. It ensures that experiments can be replicated across different laboratories and that the effects observed are truly due to the variables being studied, rather than inconsistencies in solution preparation.
Consider the implications in drug development, where even slight variations in concentration can drastically alter a drug's efficacy or toxicity. Or in environmental monitoring, where precisely measuring pollutant levels is critical for protecting public health and ecosystems.
Therefore, understanding and applying the correct methodologies for determining and expressing concentration are fundamental to achieving meaningful and reliable results in both research and applied settings.
Common Units of Concentration
Various units of concentration exist, each with its own strengths and weaknesses. The choice of which unit to use often depends on the specific application and the nature of the substances involved. Let's examine three of the most common:
Molarity (M): Moles per Liter
Molarity (M) is defined as the number of moles of solute per liter of solution (mol/L). This is perhaps the most frequently used unit of concentration in chemistry due to its direct relationship to stoichiometry.
For instance, if you dissolve 1 mole of sodium chloride (NaCl) in enough water to make 1 liter of solution, you have a 1 M solution of NaCl.
Molarity is convenient for reactions performed in solution because it directly relates the volume of a solution to the number of moles of reactant present. This makes calculating the amounts of reactants needed for a reaction straightforward.
Example Calculation: To prepare 0.5 L of a 2.0 M solution of glucose (C6H12O6), you would need to dissolve (2.0 mol/L) (0.5 L) = 1.0 mole of glucose. Convert moles to grams using the molar mass of glucose (180.16 g/mol): 1.0 mol 180.16 g/mol = 180.16 g of glucose.
Molality (m): Moles per Kilogram
Molality (m) is defined as the number of moles of solute per kilogram of solvent (mol/kg). This unit is particularly useful when dealing with properties of solutions that are temperature-dependent, such as boiling point elevation and freezing point depression.
Unlike molarity, molality is independent of temperature because it is based on mass, which does not change with temperature.
This contrasts with molarity, which is based on volume and can change with temperature due to the expansion or contraction of liquids.
For this reason, molality is often preferred over molarity in experiments where temperature variations are significant.
Example Use Case: When studying colligative properties like freezing point depression, where the change in freezing point is directly proportional to the molality of the solution.
Mass Percent (% w/w): Grams per 100 Grams
Mass percent (% w/w), also known as weight percent, expresses the concentration as the mass of solute divided by the total mass of the solution, multiplied by 100%.
Mass percent is useful when dealing with solid mixtures or when the molar mass of the solute is unknown or difficult to determine.
It is also a convenient way to express concentrations in industrial settings where solutions are often prepared by weight rather than by volume.
The masses of the solute and solution must be determined accurately, typically using an analytical balance. Ensure the balance is calibrated correctly and that the sample is free of contaminants.
Remember to account for the mass of the container when weighing the solute and the final solution.
Formula: % w/w = (Mass of solute / Mass of solution)
**100
**Example:If you dissolve 10 grams of NaCl in 90 grams of water, the mass percent of NaCl in the solution is (10 g / (10 g + 90 g)) 100 = 10%.
Tools of the Trade: Preparing Solutions with Precision
The creation of solutions with accurate and precise concentrations hinges not only on understanding the underlying chemistry but also on the skillful use of laboratory equipment. This section elucidates the function and proper operation of key tools essential for solution preparation.
From the analytical balance to volumetric flasks, each instrument plays a critical role in achieving the desired concentration with minimal error. Mastery of these techniques is crucial for any chemist or laboratory professional.
Analytical Balances: The Foundation of Accurate Mass Measurement
The analytical balance is arguably the most fundamental tool for solution preparation, providing the means to measure the mass of the solute with high accuracy. These balances are designed to minimize environmental influences and provide readings with a high degree of precision, often to the nearest 0.0001 gram.
Calibration and Maintenance
To ensure the accuracy of mass measurements, analytical balances must be calibrated regularly using certified calibration weights. Calibration corrects for any drift in the balance's sensor and ensures that the readings are traceable to a known standard.
Regular maintenance, including cleaning and leveling the balance, is also crucial for maintaining its performance. Dust, vibrations, and an unlevel surface can all introduce errors into mass measurements.
Factors Affecting Measurements
Several factors can influence the accuracy of measurements made with an analytical balance. These include:
- Air currents: Minimize air currents by closing the balance's doors during measurements.
- Temperature: Allow samples to equilibrate to room temperature before weighing, as temperature differences can cause air buoyancy errors.
- Static electricity: Static electricity can cause powders to cling to surfaces or repel each other, leading to inaccurate measurements. Use an antistatic device or carefully discharge the sample before weighing.
Volumetric Flasks: Achieving Precise Concentrations
Volumetric flasks are designed to contain a specific volume of liquid at a given temperature, typically 20°C. These flasks are essential for preparing solutions with accurate concentrations, as they allow you to dilute a known mass of solute to a precise final volume.
Proper Techniques for Filling and Mixing
When preparing a solution in a volumetric flask, first dissolve the solute in a small amount of solvent in a separate beaker.
Then, carefully transfer the solution to the volumetric flask, rinsing the beaker several times to ensure that all of the solute is transferred. Add solvent to the flask until the liquid level is close to the calibration mark.
Finally, use a dropper to add solvent until the bottom of the meniscus is exactly aligned with the calibration mark. Invert the flask several times to ensure that the solution is thoroughly mixed.
Avoiding Parallax Error
Parallax error occurs when the liquid level is viewed from an angle, causing it to appear higher or lower than it actually is. To avoid parallax error, always view the meniscus at eye level when filling a volumetric flask.
Volumetric and Graduated Pipettes: Accurate Liquid Transfer
Pipettes are used to accurately transfer specific volumes of liquid. Volumetric pipettes are designed to deliver a single, fixed volume, while graduated pipettes have markings along their length, allowing for the delivery of variable volumes.
Types of Pipettes and Their Applications
Volumetric pipettes are the most accurate type of pipette and are used when a precise volume of liquid needs to be transferred. Graduated pipettes are less accurate but more versatile, as they can be used to deliver a range of volumes. Micropipettes are specialized pipettes designed to transfer very small volumes, typically in the microliter range.
The choice of pipette depends on the desired accuracy and the volume of liquid to be transferred. For preparing solutions, volumetric pipettes are generally preferred for their high accuracy.
Burettes: Precise Dispensing for Titrations
Burettes are long, graduated glass tubes with a stopcock at the bottom, used to dispense precise volumes of liquid, particularly in titrations. The burette allows for the controlled addition of a titrant to a solution until the endpoint of the reaction is reached.
Reading and Using Burettes Correctly
To use a burette correctly, first rinse it with the titrant to remove any contaminants. Then, fill the burette with the titrant, making sure to remove any air bubbles from the tip.
Record the initial volume of the titrant in the burette, reading the bottom of the meniscus at eye level. During the titration, slowly add the titrant to the solution, swirling the solution continuously.
As the endpoint is approached, add the titrant dropwise until the indicator changes color or the desired endpoint is reached. Record the final volume of the titrant in the burette and calculate the volume of titrant added by subtracting the initial volume from the final volume.
Solution Properties and Behavior: Solubility and Titration
Understanding the characteristics that govern how substances dissolve and react in solution is paramount in chemistry. Two key concepts in this domain are solubility and titration, each providing unique insights into solution behavior.
Solubility dictates the extent to which a solute can dissolve in a solvent, while titration offers a quantitative approach to determine the concentration of a solution.
Solubility: The Extent of Dissolution
Solubility is defined as the maximum amount of a solute that can dissolve in a specific amount of solvent at a given temperature and pressure to form a saturated solution. It is a crucial property that dictates the behavior of solutions in diverse applications, ranging from pharmaceutical formulations to environmental processes.
Solubility is typically expressed in units of grams of solute per liter (g/L) or moles of solute per liter (mol/L) of solvent.
Factors Affecting Solubility
Several factors influence the solubility of a solute in a solvent. The most important of these are temperature, pressure, and the nature of the solute and solvent themselves.
Temperature
For most solid solutes, solubility increases with increasing temperature. This is because dissolving a solid often requires energy to break the bonds holding the solid lattice together. This energy is supplied by heat.
However, the effect of temperature on the solubility of gases is opposite. The solubility of gases in liquids typically decreases with increasing temperature because the gas molecules have more kinetic energy and are more likely to escape from the solution.
Pressure
Pressure has a significant effect on the solubility of gases, but it has little to no effect on the solubility of solids or liquids.
Henry's Law states that the solubility of a gas in a liquid is directly proportional to the partial pressure of the gas above the liquid.
This means that increasing the pressure of a gas above a liquid will increase the amount of gas that dissolves in the liquid.
Nature of Solute and Solvent
The "like dissolves like" rule is a useful guideline for predicting solubility. Polar solutes tend to dissolve in polar solvents, while nonpolar solutes tend to dissolve in nonpolar solvents.
This is because the intermolecular forces between the solute and solvent molecules must be similar in order for the solute to dissolve.
For example, water (a polar solvent) readily dissolves ionic compounds such as sodium chloride (NaCl), which are also polar. On the other hand, oil (a nonpolar solvent) readily dissolves nonpolar substances like fats.
Titration: Quantifying Solution Concentration
Titration is a quantitative analytical technique used to determine the concentration of an unknown solution (the analyte) by reacting it with a solution of known concentration (the titrant). This technique is widely used in chemistry, biology, and medicine to analyze the composition of solutions.
The process involves the gradual addition of the titrant to the analyte until the reaction between them is complete, a point known as the equivalence point.
Performing a Titration
A typical titration setup involves a burette containing the titrant, a flask containing the analyte, and an indicator to signal the endpoint of the reaction.
The titrant is slowly added to the analyte while continuously stirring the solution. The indicator changes color near the equivalence point, signaling the endpoint of the titration.
The volume of titrant required to reach the endpoint is carefully measured, and this value is then used to calculate the concentration of the analyte.
Several types of titrations exist, including acid-base titrations, redox titrations, and complexometric titrations, each based on a different type of chemical reaction.
Solution Calculations: Dilution Made Easy
Dilution is a fundamental technique in chemistry and biology, involving the reduction of a solution's concentration by adding more solvent. This process is essential for preparing solutions of desired concentrations from stock solutions, ensuring accurate measurements and consistent experimental results.
A clear understanding of dilution principles and calculations is crucial for anyone working in a laboratory setting.
Understanding Dilution: Lowering Concentration
At its core, dilution is the process of decreasing the concentration of a solute in a solution.
This is achieved by adding more solvent to the existing solution, increasing the total volume while keeping the amount of solute constant.
The result is a solution with a lower concentration than the original stock solution.
The Dilution Equation: M1V1 = M2V2
The dilution equation, M1V1 = M2V2, is the cornerstone for calculating the volumes and concentrations involved in dilution processes.
Where:
- M1 = Initial concentration (molarity)
- V1 = Initial volume
- M2 = Final concentration (molarity)
- V2 = Final volume
This equation is based on the principle that the number of moles of solute remains constant during dilution.
By knowing three of these values, one can easily calculate the fourth.
Example Application: Calculating Dilution Volume
Suppose you have a 1.0 M stock solution of NaCl and you need to prepare 100 mL of a 0.1 M solution. How would you do that?
Using M1V1 = M2V2:
- M1 = 1.0 M
- V1 = ?
- M2 = 0.1 M
- V2 = 100 mL
Rearranging the equation to solve for V1: V1 = (M2V2) / M1
V1 = (0.1 M
**100 mL) / 1.0 M = 10 mL
Therefore, to prepare the solution, you would take 10 mL of the 1.0 M stock solution and dilute it with enough solvent (usually water) to reach a final volume of 100 mL.
Serial Dilutions: Step-Wise Concentration Reduction
**Serial dilutions
**involve performing a series of dilutions to achieve a highly diluted solution from a concentrated stock solution.
Instead of diluting the stock solution in one large step, multiple smaller dilutions are performed sequentially.
Advantages of Serial Dilutions
Serial dilutions offer several advantages:
- **Accuracy:
**Minimizes errors associated with measuring small volumes or making very large dilutions in a single step.
- **Precision:
**Provides better control over the final concentration, especially when preparing extremely dilute solutions.
- **Range:
**Extends the range of measurable concentrations, allowing for the quantification of substances present in very low amounts.
How to Perform Serial Dilutions
A serial dilution is performed as follows:
- Prepare a series of dilution tubes or vessels.
- Transfer a specific volume of the original stock solution to the first tube, and dilute it with a specific amount of solvent, according to the dilution factor (e.g., 1:10 dilution).
- Mix the solution in the first tube well.
- Transfer the**samevolume from the first tube to thenext* tube, repeating the same dilution factor.
- Repeat steps 3 and 4 for subsequent tubes, until the desired range of dilutions is achieved.
Video: Mass of Solute Meaning: Chemistry Guide
Frequently Asked Questions
What is the mass of solute?
The mass of solute is the quantity of solute, measured in units like grams or kilograms, that is dissolved in a solvent to form a solution. Understanding the mass of solute meaning is essential for calculating concentration.
How does the mass of solute differ from concentration?
The mass of solute meaning refers to the amount of the solute itself. Concentration, on the other hand, describes how much solute is present relative to the amount of solvent or solution. Concentration units include molarity and percentage.
Why is knowing the mass of solute important?
Knowing the mass of solute is important because it lets us precisely determine the concentration of a solution, allowing for accurate chemical reactions and analysis. It’s fundamental to the mass of solute meaning and its applications.
How do you calculate the mass of solute in a solution?
The mass of solute can be determined experimentally by evaporating the solvent and weighing the remaining solute. Alternatively, if the concentration and volume of the solution are known, the mass of solute can be calculated using the appropriate concentration formula. The mass of solute meaning is directly linked to these calculations.
So, there you have it! Hopefully, this clears up any confusion about the mass of solute meaning and how to calculate it. Keep experimenting, and don't be afraid to revisit this guide if you need a quick refresher. Happy chemistry-ing!