Load Resistance: A Beginner's Complete Guide
In electrical engineering, load resistance dictates the current flow through a circuit, influencing the performance of components like operational amplifiers (op-amps). The value of the load resistance impacts the power dissipated by elements connected to power supplies, and its proper calculation is crucial for designing efficient and stable circuits. Organizations such as the Institute of Electrical and Electronics Engineers (IEEE) provide standards and guidelines for understanding and implementing load resistance considerations in various applications. When troubleshooting circuit behavior using a multimeter, understanding load resistance helps in identifying faults and ensuring optimal performance.
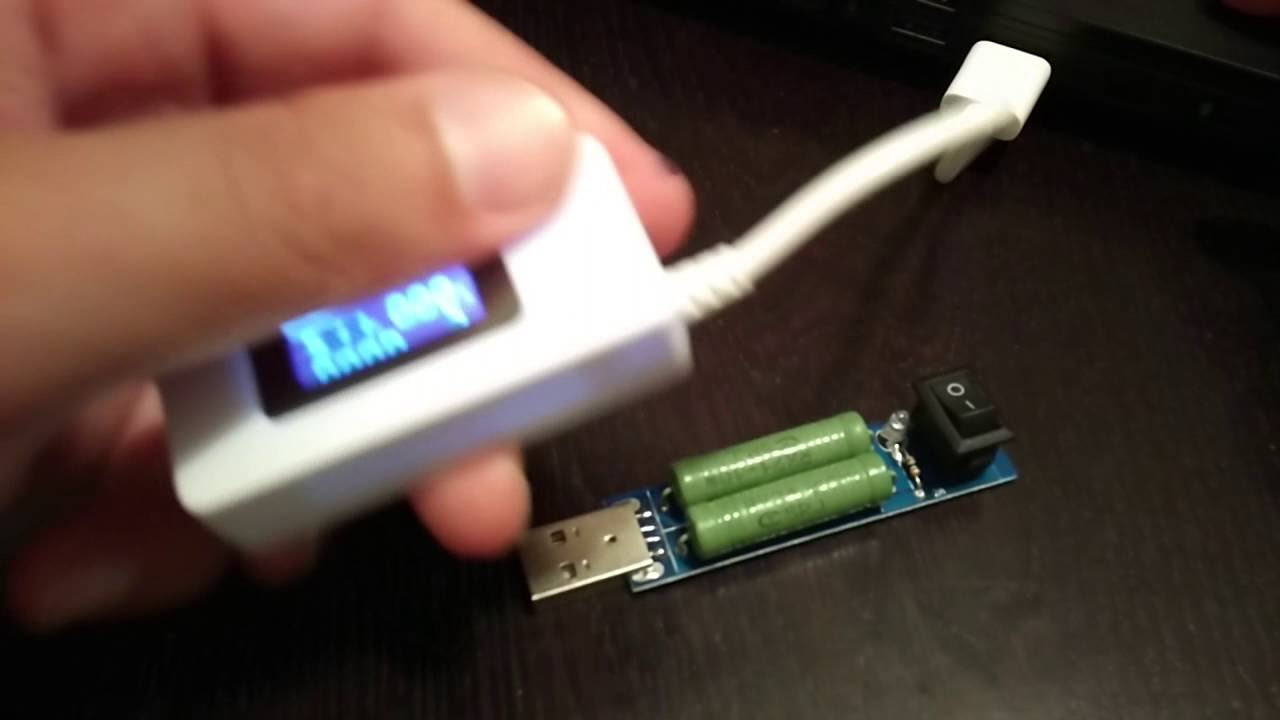
Image taken from the YouTube channel Gearbest stuff , from the video titled USB Voltage and Current Detector with USB Load Resistance Battery Capacity Tester .
Electrical resistance, a fundamental property of electrical circuits, is the opposition to the flow of electric current. It's the invisible force that governs how electricity behaves, shaping everything from the brightness of a lightbulb to the speed of a processor. Understanding resistance is not just academic; it's essential for anyone working with or designing electrical systems.
The Nature of Electrical Resistance
At its core, electrical resistance stems from the interactions between moving electrons and the atoms within a material. As electrons navigate through a conductor, they collide with atoms, impeding their flow. This impedance is what we define as resistance. The greater the resistance, the more difficult it is for current to pass through.
The Importance of Resistance
Understanding resistance is paramount for effective circuit design. By carefully selecting resistors, engineers can precisely control current flow and voltage levels, ensuring that each component operates within its specified limits. This control is vital for both performance and safety.
Troubleshooting electrical problems also heavily relies on understanding resistance. Measuring resistance values helps to pinpoint faulty components, identify shorts, and diagnose wiring issues. Without this knowledge, diagnosing issues within electrical systems becomes guesswork.
Resistance in the Real World
Resistance isn't some abstract concept confined to textbooks. It manifests in countless everyday applications. Heating elements in appliances like toasters and ovens utilize high resistance wires to generate heat. In electronics, resistors are vital for setting bias currents in transistors, dividing voltages, and limiting current to sensitive components like LEDs.
Even the human body exhibits electrical resistance. Measuring skin resistance is a technique used in lie detectors and some biofeedback devices. These diverse applications demonstrate how ubiquitous and essential resistance is.
What We'll Explore
This exploration will delve into the essential aspects of electrical resistance. We'll begin by establishing the foundational concepts like Ohm's Law and the relationships between voltage, current, and power. Next, we will investigate how resistance manifests in series and parallel circuits. This will cover the characteristics of different resistor types. Finally, we'll cover applications of resistors and how to measure resistance safely and accurately.
By the end of our investigation, you'll have a comprehensive understanding of electrical resistance and its crucial role in the world of electricity.
Foundational Concepts: The Building Blocks of Resistance
Electrical resistance, a fundamental property of electrical circuits, is the opposition to the flow of electric current. It's the invisible force that governs how electricity behaves, shaping everything from the brightness of a lightbulb to the speed of a processor. Understanding resistance is not just academic; it's essential for anyone working with or designing electrical systems. To truly grasp the intricacies of resistance, we must first delve into some foundational concepts.
Resistance (Electrical): The Obstacle to Current Flow
At its core, electrical resistance is the opposition a material offers to the flow of electric current. Imagine it as a narrow pipe restricting the flow of water. The greater the resistance, the harder it is for current to pass through.
Resistance is what transforms electrical energy into other forms, like heat in a toaster or light in a bulb.
Ohm's Law: The Cornerstone of Circuit Analysis
Perhaps the most fundamental relationship in electrical engineering is Ohm's Law, expressed as V = IR. This simple equation connects Voltage (V), Current (I), and Resistance (R). Let's break it down:
Voltage (V): The Electrical Potential Difference
Voltage, measured in Volts (V), represents the electrical potential difference between two points in a circuit. It's the "push" that drives the current. Think of it as the pressure in our water pipe analogy. Without voltage, there is no current flow.
Current (I): The Flow of Electrical Charge
Current, measured in Amperes (A), is the rate of flow of electrical charge. It's the quantity of charge passing a point in a circuit per unit of time. In our water analogy, it's the amount of water flowing through the pipe.
Resistance (R): The Opposition to Flow
Resistance, measured in Ohms (Ω), is the opposition to current flow. It dictates how much current will flow for a given voltage. Using our water analogy, it's the degree of constriction in the pipe.
Applying Ohm's Law: Examples
Let's illustrate Ohm's Law with a simple example: If a resistor with a resistance of 10 Ohms (Ω) has a voltage of 5 Volts (V) applied across it, the current flowing through it will be:
I = V / R = 5V / 10Ω = 0.5 Amperes (A).
Conversely, if we know the current and resistance, we can calculate the voltage. Understanding this interplay is crucial for designing and troubleshooting circuits.
Power (P): The Rate of Energy Consumption
Power, measured in Watts (W), is the rate at which electrical energy is transferred or consumed in a circuit. It tells us how quickly energy is being used. The relationship between Power (P), Voltage (V), and Current (I) is defined by the formula:
P = VI: The Fundamental Power Equation
This equation states that power is equal to the product of voltage and current. It's the most direct way to calculate power when both voltage and current are known.
P = I²R: Power Dissipation Based on Current
This equation shows that power is also equal to the square of the current multiplied by the resistance. This is especially useful for calculating the power dissipated by a resistor when the current flowing through it is known.
P = V²/R: Power Dissipation Based on Voltage
This equation shows that power is also equal to the square of the voltage divided by the resistance. This is useful for calculating the power dissipated by a resistor when the voltage across it is known.
Power Rating: Preventing Overload
Every resistor has a power rating, which is the maximum power it can safely dissipate without being damaged. Exceeding this rating can lead to overheating, failure, and even fire. In circuit design, it's crucial to select resistors with adequate power ratings to ensure reliability and safety.
The Ohm (Ω): Quantifying Resistance
The Ohm (Ω) is the standard unit of measurement for electrical resistance, named after Georg Ohm, the discoverer of Ohm's Law. It quantifies the amount of opposition to current flow. A higher Ohm value indicates greater resistance.
Circuit Configurations: Series and Parallel Connections
Having explored the fundamental concepts of resistance, voltage, current, and Ohm's Law, it's now time to delve into how resistors behave when connected in different configurations within a circuit. These configurations primarily fall into two categories: series and parallel. Understanding the characteristics of each configuration is crucial for analyzing and designing functional electrical circuits. In addition to series and parallel configurations, we will explore Kirchhoff’s Laws, which are foundational to circuit analysis, providing a systematic approach to understand electrical circuits.
Series Circuits: One Path for Current
In a series circuit, resistors are connected end-to-end, forming a single pathway for current to flow. This means that the current flowing through each resistor in the series is identical. This singular path dictates specific relationships between resistance, voltage, and current.
Calculating Total Resistance in Series
The total resistance in a series circuit (Rtotal) is simply the sum of all individual resistances. Mathematically, this is expressed as:
Rtotal = R1 + R2 + R3 + ... + Rn
This means that adding more resistors in series will always increase the total resistance of the circuit.
Current Behavior in Series Circuits
A defining characteristic of series circuits is that the current is the same at every point in the circuit. This is because there is only one path for the electrons to flow. Consequently, the current flowing through each resistor is identical to the total current supplied by the voltage source.
Voltage Distribution in Series Circuits
In a series circuit, the total voltage supplied by the source is divided across the individual resistors. The amount of voltage each resistor receives is directly proportional to its resistance value. This is described by the voltage divider rule. The voltage drop across any resistor Rx in the series is:
Vx = Vtotal
**(Rx / Rtotal)
Where Vtotal is the total voltage supplied by the source, Rx is the resistance of the resistor under consideration, and Rtotal is the total series resistance.
Parallel Circuits: Multiple Paths for Current
In contrast to series circuits, parallel circuits provide multiple pathways for current to flow. Here, resistors are connected side-by-side, allowing current to split and flow through each resistor independently.
Calculating Total Resistance in Parallel
Calculating the total resistance in a parallel circuit is a bit more involved than in a series circuit. The reciprocal of the total resistance is equal to the sum of the reciprocals of the individual resistances. This can be written as:
1/Rtotal = 1/R1 + 1/R2 + 1/R3 + ... + 1/Rn
To find Rtotal, you must take the reciprocal of the sum calculated above. A key takeaway is that adding more resistors in parallel will decrease the total resistance of the circuit.
Voltage Behavior in Parallel Circuits
In a parallel circuit, the voltage across each resistor is the same. This is because all resistors are directly connected to the voltage source. Therefore, each resistor experiences the full voltage supplied by the source.
Current Distribution in Parallel Circuits
The total current flowing into a parallel circuit is divided among the individual resistors. The amount of current flowing through each resistor is inversely proportional to its resistance value. Higher resistance means lower current, and vice versa. According to the current divider rule, the current through resistor Rx is:
Ix = Itotal** (Rtotal / Rx)
Where Itotal is the total current entering the parallel branch, Rtotal is the equivalent resistance of the parallel combination, and Rx is the resistance of the resistor you're calculating the current for.
Kirchhoff's Laws: Powerful Tools for Circuit Analysis
Kirchhoff's Laws provide a systematic method for analyzing more complex circuits, particularly those involving both series and parallel combinations of resistors. There are two fundamental laws: Kirchhoff's Current Law (KCL) and Kirchhoff's Voltage Law (KVL).
Kirchhoff's Current Law (KCL)
Kirchhoff's Current Law, also known as the junction rule, states that the total current entering a junction (or node) in a circuit is equal to the total current leaving the junction. In simpler terms, the current flowing into a point must equal the current flowing out of that point.
Mathematically, this can be expressed as:
∑Iin = ∑Iout
KCL is based on the principle of conservation of charge.
Example: Imagine a junction where 2A and 3A of current enter, then the total current exiting the junction must equal 5A.
Kirchhoff's Voltage Law (KVL)
Kirchhoff's Voltage Law, also known as the loop rule, states that the sum of the voltage drops around any closed loop in a circuit is equal to zero. This implies that the total voltage supplied by the source must equal the sum of the voltage drops across all components in the loop.
Mathematically, this can be expressed as:
∑V = 0
KVL is based on the principle of conservation of energy.
Example: In a closed loop with a 12V source and two resistors, the sum of the voltage drops across the two resistors must equal 12V.
Kirchhoff's Laws, alongside Ohm's Law, offer the fundamental tools required for analyzing and understanding the behavior of electrical circuits, regardless of their complexity. By applying these principles, one can effectively determine voltage, current, and resistance values throughout a circuit, enabling efficient design and troubleshooting.
Resistor Characteristics: Types, Properties, and Markings
Having explored the fundamental concepts of resistance, voltage, current, and Ohm's Law, it's now time to delve into the characteristics of resistors themselves. Resistors are not all created equal; they come in various types, possess unique properties, and are marked in specific ways to indicate their values and tolerances. Understanding these aspects is crucial for selecting the right resistor for a given application and ensuring circuit performance.
Resistors: The Foundation of Resistance
Resistors are the fundamental components in electronic circuits designed to provide a specific resistance to the flow of electrical current. They are passive components, meaning they do not generate energy but rather dissipate it in the form of heat. The choice of resistor significantly impacts circuit behavior, stability, and overall functionality.
Fixed Resistors: The Constant Value
Fixed resistors are the most common type of resistor, offering a single, non-adjustable resistance value. These resistors are manufactured with a specific resistance that remains relatively constant under normal operating conditions. They are widely used in various applications, from simple current limiting to more complex signal conditioning circuits.
Variable Resistors (Potentiometers/Rheostats): Adjustable Resistance
Variable resistors, unlike their fixed counterparts, offer the ability to adjust their resistance values. These components are commonly known as potentiometers and rheostats.
Potentiometers vs. Rheostats: What's the Difference?
While often used interchangeably, potentiometers and rheostats differ in their connection and application. A potentiometer is a three-terminal device used as a voltage divider, allowing for the selection of a voltage level between the two extremes. A rheostat, on the other hand, is a two-terminal device used to control current flow by varying the resistance in a circuit.
Resistor Color Code: Decoding the Bands
The resistor color code is a standardized coding system that uses colored bands to indicate a resistor's resistance value and tolerance. This system allows for quick and easy identification of resistor values without the need for direct measurement.
Understanding the Color Code Chart
The color code chart assigns a numerical value to each color. Typically, resistors have four bands: the first two bands represent the first two digits of the resistance value, the third band represents the multiplier (power of ten), and the fourth band represents the tolerance. Some resistors have a fifth band indicating reliability or temperature coefficient.
Decoding Examples: Putting it into Practice
For example, a resistor with bands of brown, black, red, and gold would have a resistance of 1000 ohms (10 x 10^2) with a 5% tolerance. Learning to quickly decipher the color code is an essential skill for any electronics enthusiast or professional.
Resistor Tolerance: Accounting for Deviation
Resistor tolerance specifies the permissible deviation, expressed as a percentage, of a resistor's actual resistance from its stated value. A 5% tolerance resistor, for instance, may have a resistance that is 5% higher or lower than the marked value.
In circuit design, understanding tolerance is crucial for predicting circuit performance and ensuring that components operate within their intended ranges.
Power Rating (Wattage): Preventing Overload
The power rating of a resistor represents the maximum power that it can safely dissipate without being damaged. Exceeding the power rating can lead to overheating, failure, and even fire. It is essential to select resistors with an appropriate power rating for the intended application.
Electrical Conductivity and Resistivity: Intrinsic Properties
Electrical conductivity describes a material's ability to conduct electricity, while electrical resistivity describes its opposition to current flow. Resistors are made from materials with specific resistivity to achieve desired resistance values. Conductivity and resistivity are intrinsic properties influenced by a material's atomic structure and temperature.
Thermistors: Temperature-Sensitive Resistors
Thermistors are resistors whose resistance changes significantly with temperature. They are used in temperature sensing and control applications. Two main types of thermistors exist: Negative Temperature Coefficient (NTC) thermistors, whose resistance decreases with increasing temperature, and Positive Temperature Coefficient (PTC) thermistors, whose resistance increases with increasing temperature.
Photoresistors (Light Dependent Resistors/LDRs): Light-Sensitive Resistors
Photoresistors, also known as Light Dependent Resistors (LDRs), are resistors whose resistance changes with the intensity of light. They are used in light-sensitive circuits. Their resistance decreases as light intensity increases, allowing them to be used as light sensors in various applications, such as light meters and automatic lighting controls.
Resistor Applications: Putting Resistance to Work
Having explored the fundamental concepts of resistance, voltage, current, and Ohm's Law, it's now time to delve into the characteristics of resistors themselves. Resistors are not all created equal; they come in various types, possess unique properties, and are marked in specific ways to indicate their electrical resistance values. This section will move beyond theory and examine how resistors are actively employed in diverse electronic applications, demonstrating their practical significance in circuit design.
We will explore specific examples such as LED circuits, voltage dividers, and the ubiquitous pull-up and pull-down resistor configurations. By examining these applications, the crucial role of resistors in shaping circuit behavior and ensuring component protection will become abundantly clear.
LED Circuits: Current Control
One of the most common and essential applications of resistors is in LED (Light Emitting Diode) circuits. LEDs are current-sensitive devices, meaning that exceeding their maximum current rating can lead to immediate failure. Resistors act as current-limiting devices in series with the LED.
Without a properly sized resistor, an LED would draw excessive current, resulting in overheating and potential burnout.
Calculating the appropriate resistor value involves considering the LED's forward voltage (Vf), the desired forward current (If), and the supply voltage (Vs). The formula used is derived from Ohm's Law:
R = (Vs - Vf) / If
By carefully selecting the resistor value, designers can ensure that the LED operates within its specified parameters, maximizing its lifespan and efficiency. This simple application showcases the critical role of resistance in protecting sensitive components.
Voltage Dividers: Precise Voltage Levels
Voltage dividers are resistor networks designed to generate specific voltage levels from a higher input voltage. This is achieved by connecting two or more resistors in series, with the output voltage taken across one of the resistors.
The voltage divider formula allows for the calculation of the output voltage (Vout) based on the input voltage (Vin) and the values of the resistors (R1 and R2):
Vout = Vin * (R2 / (R1 + R2))
Voltage dividers are extensively used in various applications, including:
- Sensor interfacing: Scaling down sensor output voltages to match the input range of microcontrollers or analog-to-digital converters (ADCs).
- Bias circuits: Establishing stable operating points for transistors and other active components.
- Reference voltages: Providing accurate voltage references for comparators and other circuits.
The ability to create precise voltage levels with simple resistor networks makes voltage dividers an indispensable tool in electronic design.
Pull-up and Pull-down Resistors: Defining Logic States
Pull-up and pull-down resistors are crucial for defining the logic states of digital input pins, particularly in microcontroller-based systems. These resistors ensure that a digital input pin has a defined voltage level (either high or low) when no external signal is applied.
A pull-up resistor is connected between the input pin and the positive supply voltage (VCC). When the input is not actively driven low by an external signal, the resistor pulls the voltage up to VCC, representing a logic high state.
Conversely, a pull-down resistor is connected between the input pin and ground. When the input is not actively driven high, the resistor pulls the voltage down to ground, representing a logic low state.
These resistor configurations are essential for:
- Switch inputs: Ensuring a clean and reliable signal from mechanical switches or buttons.
- Open-drain outputs: Providing a high level when the output is not actively driven low.
- Preventing floating inputs: Avoiding undefined or erratic behavior caused by unconnected input pins.
The strategic use of pull-up and pull-down resistors ensures proper signal integrity and reliable operation in digital circuits.
Current Limiting: Component Protection
Beyond LED circuits, current limiting resistors are employed throughout electronics to protect sensitive components from overcurrent conditions. This is especially important for ICs (Integrated Circuits), transistors, and other devices with specific current limitations.
By strategically placing a resistor in series with a component, the maximum current flowing through it can be controlled and limited. This prevents damage due to excessive current draw, extending the lifespan and reliability of the circuit.
Broad Applications in Electronic Circuits
In conclusion, the applications of resistors extend far beyond these specific examples. Resistors serve as fundamental building blocks in countless electronic circuits, performing essential functions such as:
- Load resistors: Providing a defined impedance for signal termination.
- Feedback resistors: Controlling the gain and stability of amplifiers.
- Filtering resistors: Shaping the frequency response of filters.
The versatility and ubiquity of resistors underscore their importance in the world of electronics. Understanding their properties and applications is crucial for anyone involved in circuit design, troubleshooting, or repair. From simple LED circuits to complex control systems, resistors play a vital role in shaping the behavior of electronic devices.
Measurement and Testing: Verifying Resistance Values
Resistor Applications: Putting Resistance to Work Having explored the fundamental concepts of resistance, voltage, current, and Ohm's Law, it's now time to delve into the characteristics of resistors themselves. Resistors are not all created equal; they come in various types, possess unique properties, and are marked in specific ways to indicate their resistance value. This section will delve into the essential tools and techniques required to accurately measure resistance, emphasizing the use of multimeters and ohmmeters.
The Essential Multimeter
The multimeter stands as a cornerstone in the toolkit of any electronics enthusiast, technician, or engineer. Its versatility lies in its ability to measure voltage, current, and resistance, making it an indispensable instrument for circuit diagnostics and component testing.
Measuring Resistance with a Multimeter
To accurately measure resistance with a multimeter, follow these essential steps:
-
Power Down: Always disconnect the circuit from its power source. This is crucial for your safety and to prevent damage to the multimeter.
-
Isolation: Ensure the resistor being measured is isolated from the rest of the circuit. Parallel paths can significantly skew the reading. Desolder one leg of the resistor if necessary to isolate it.
-
Selection: Select the resistance (Ω) measurement mode on the multimeter.
-
Range Selection: Choose an appropriate resistance range. If you're unsure of the resistor's value, start with the highest range and gradually decrease it until you get a stable reading. Most auto-ranging multimeters handle this automatically.
-
Connection: Connect the multimeter probes to the resistor's leads. Ensure a firm and clean connection for an accurate reading.
-
Reading: Observe the resistance value displayed on the multimeter's screen.
Safety First: Multimeter Precautions
Working with electrical circuits requires a strong awareness of safety protocols. Here are key safety precautions when using a multimeter:
-
Never measure resistance in a live circuit. Ensure the circuit is de-energized to avoid damaging the meter and risking personal injury.
-
Inspect the multimeter probes and leads for any damage before use. Damaged leads can provide inaccurate readings or pose a safety hazard.
-
Be aware of the multimeter's voltage and current ratings. Exceeding these ratings can damage the meter or create a safety risk.
-
When in doubt, consult the multimeter's user manual for specific instructions and safety guidelines.
The Dedicated Ohmmeter
While multimeters offer versatile measurement capabilities, the ohmmeter is specifically designed for measuring resistance. Ohmmeters are often found in specialized applications or in older equipment, though the multimeter has largely replaced them due to its wider functionality.
Multimeter vs. Ohmmeter: A Comparison
The key difference lies in versatility. A multimeter is a multi-function tool, whilst the ohmmeter is designed for measuring resistance. Both measure resistance by passing a small current through the resistor and measuring the voltage drop; however, the dedicated ohmmeter may offer higher accuracy in specific scenarios, particularly when measuring very low or very high resistances.
In summary, both multimeters and ohmmeters are essential for verifying resistance values in circuits. However, modern multimeters provide a convenient and safe way of reading the resistance of any circuit.
Safety Considerations: Handling Electrical Components Responsibly
Measurement and Testing: Verifying Resistance Values Resistor Applications: Putting Resistance to Work
Having explored the fundamental concepts of resistance, voltage, current, and Ohm's Law, it's now time to delve into the characteristics of resistors themselves. Resistors are not all created equal; they come in various types, possess unique prope…
Working with electrical circuits and components can be incredibly rewarding, unlocking the potential to create innovative devices and systems. However, it's paramount to acknowledge the inherent risks associated with electricity. This section underscores the critical importance of safety precautions to prevent electrical hazards. Understanding and adhering to these guidelines is not merely a suggestion—it's an absolute necessity for anyone engaging with electrical work.
The Primacy of Electrical Safety
Electrical safety encompasses a range of precautions and procedures designed to minimize the risk of electrical shock, burns, and other injuries. Electricity, while providing numerous benefits, can be extremely dangerous if not handled correctly. A lapse in judgment or a failure to follow safety protocols can have severe, even fatal, consequences. Complacency is the enemy of safety.
Essential Safety Protocols
Several fundamental practices should be ingrained in the routine of any individual working with electrical circuits. These are the cornerstones of a safe working environment.
Disconnecting Power: The First Line of Defense
The most crucial safety rule is to always disconnect power before commencing any work on a circuit. This simple step eliminates the potential for electrical shock. Ensure the circuit is de-energized at the source—whether it's unplugging a device, flipping a circuit breaker, or removing a fuse.
It's also advisable to verify the absence of voltage using a reliable multimeter before touching any wires or components. Trust, but verify.
The Importance of Insulated Tools
Using insulated tools is another vital safety measure. These tools are specifically designed with non-conductive handles to prevent electricity from flowing through your body in case of accidental contact with a live wire.
Inspect your tools regularly for any signs of damage to the insulation, such as cracks or tears. Compromised insulation renders the tool unsafe.
Avoiding Wet Environments
Water is an excellent conductor of electricity, significantly increasing the risk of electrical shock. Therefore, never work on electrical circuits in wet or damp environments.
Ensure that your hands are dry, and avoid placing beverages or other liquids near your workspace. A seemingly minor spill can create a hazardous situation.
When to Seek Professional Help
There are situations where attempting to troubleshoot or repair electrical problems yourself is simply not advisable. If you lack the necessary expertise, tools, or confidence, seek assistance from a qualified electrician.
Electrical work should never be undertaken lightly. Knowing your limitations and seeking professional help when needed is a sign of responsibility and a crucial aspect of safety.
Beyond the Basics: Advanced Safety Practices
While the aforementioned precautions form the bedrock of electrical safety, more advanced practices can further mitigate risk.
Lockout/Tagout Procedures
In industrial settings, lockout/tagout procedures are employed to ensure that equipment is completely de-energized and cannot be accidentally re-energized during maintenance or repair. These procedures involve physically locking out power sources and attaching warning tags.
Personal Protective Equipment (PPE)
Depending on the nature of the electrical work, appropriate PPE may include safety glasses, gloves, and arc-rated clothing. These items provide an extra layer of protection against potential hazards.
Regular Safety Training
Staying up-to-date with the latest safety standards and best practices is essential. Regular safety training sessions can help reinforce important concepts and ensure that everyone is aware of the potential risks.
A Culture of Safety
Ultimately, electrical safety is not just about following a set of rules; it's about cultivating a culture of safety. This means making safety a priority in every aspect of your work, from planning and preparation to execution and cleanup. It involves being mindful of your surroundings, looking out for potential hazards, and encouraging others to do the same. A proactive approach to safety is the most effective way to prevent accidents and injuries.
Video: Load Resistance: A Beginner's Complete Guide
FAQs: Load Resistance
What does "load resistance" really mean in a simple circuit?
Load resistance is the total resistance presented by the components in a circuit that are using the electrical energy. Think of it as the obstacle the current must overcome to power things like LEDs, motors, or speakers. A higher load resistance means it's harder for the current to flow.
How does load resistance affect the voltage and current in a circuit?
The load resistance determines how much current flows for a given voltage. According to Ohm's Law (V=IR), if you increase the load resistance, the current decreases, assuming the voltage remains constant. Conversely, decreasing load resistance increases current flow.
Why is matching load resistance important in some applications?
Matching load resistance, particularly in audio and RF circuits, ensures maximum power transfer from the source to the load. If the load resistance is significantly different from the source impedance, much of the power will be reflected back towards the source, resulting in inefficient energy transfer and potential damage. The ideal load resistance is equal to the source impedance in such situations.
Can the load resistance be too high or too low?
Yes, absolutely. If the load resistance is too high, very little current will flow, and the load won't function properly. If the load resistance is too low, excessive current will flow, potentially overloading the power supply or damaging circuit components. Choosing an appropriate load resistance is crucial for circuit operation.
So, that's the lowdown on load resistance! Hopefully, you now have a solid grasp on the fundamentals and can confidently apply this knowledge to your circuits and projects. Don't be afraid to experiment and see how different load resistance values affect performance. Happy tinkering!