Lever Arm Secrets: Unleash Power & Efficiency Now!
Understanding mechanical advantage is crucial for harnessing the full potential of a lever arm. This principle directly impacts efficiency, especially in systems involving torque and force application. Engineers at institutions like MIT extensively study how optimizing the lever arm can yield significant improvements in various applications, from simple machines to complex robotic systems. By strategically positioning the fulcrum, designers can maximize the output force relative to the input force, unlocking the secrets of a well-designed lever arm.
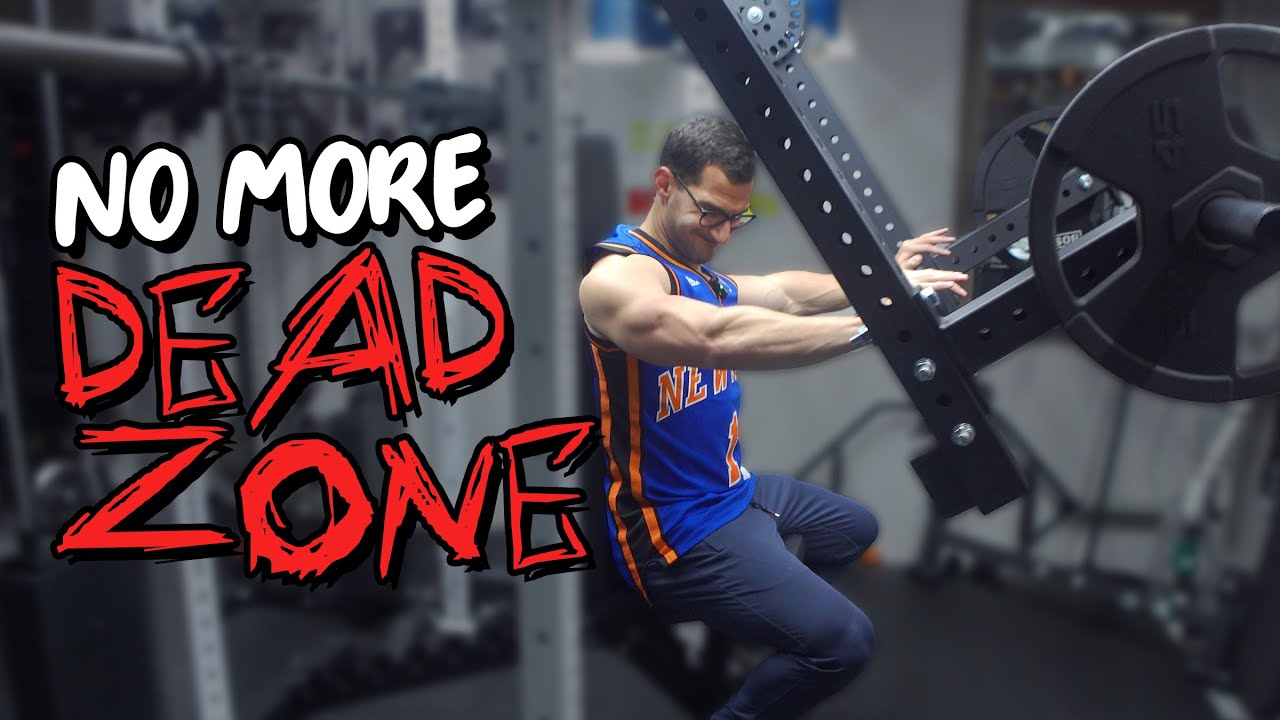
Image taken from the YouTube channel Matt Rosenman , from the video titled I Solved The Biggest Problem With Lever Arms - No More Dead Zone! .
Lever arms are all around us, often unnoticed, yet constantly working to amplify our efforts. From the simple act of opening a door to the complex machinery of construction sites, the principles of leverage are fundamental to how we interact with and manipulate the physical world. They are present in the most basic actions we perform to the most groundbreaking advancements we make.
This introduction serves as a launchpad, propelling us into a realm where the secrets of lever arms are unveiled. Understanding these secrets is the key to unlocking immense potential for increased power and efficiency in countless applications.
The Ubiquitous Lever Arm
Think about the last time you used a pair of scissors, pried open a can of paint, or even took a step. Each of these actions, seemingly simple, relies on the mechanics of a lever arm.
A lever arm, at its core, is a simple machine that magnifies force. It allows us to accomplish tasks that would otherwise be impossible, or at least require significantly more effort. Its presence is so pervasive that we often take it for granted, failing to recognize the ingenuity behind its operation.
The Promise of Amplified Power and Efficiency
The true power of a lever arm lies in its ability to provide mechanical advantage. This advantage allows us to exert a relatively small force over a longer distance to move a much larger load a shorter distance. The implications of this are profound.
Imagine lifting a heavy boulder. Without a lever, the task would be daunting, if not impossible. But with a well-placed lever and fulcrum, even a single person can move substantial weight. This concept extends far beyond simple tasks; it is at the heart of complex engineering designs, allowing for the construction of massive structures and the operation of intricate machinery.
Unveiling the Secrets: A Roadmap
This article will dissect the inner workings of lever arms, revealing the secrets that govern their behavior. We will explore the fundamental components of a lever arm system, including the lever itself, the fulcrum, and the forces involved.
We will delve into the concept of mechanical advantage and how it is determined by the arrangement of these components. From there, we will categorize the three classes of lever arms and examine their unique characteristics and applications.
Finally, we will explore practical tips for optimizing lever arm usage and maximizing efficiency in a variety of real-world scenarios. Prepare to gain a new appreciation for this fundamental principle of physics and its potential to transform the way we work and interact with the world around us.
Of course, here is the expansion you requested:
The promise of amplified power and efficiency, however, hinges on a firm grasp of the fundamental components that constitute a lever arm system. It's akin to understanding the alphabet before attempting to write a novel; without this foundational knowledge, the nuances and intricacies of lever arm mechanics remain elusive.
Deciphering the Fundamentals: Key Components of a Lever Arm
At its heart, a lever arm system is a deceptively simple arrangement. It comprises four essential elements: the lever arm itself, the fulcrum, the effort force, and the load.
Understanding each of these components and their interrelationships is paramount to unlocking the secrets of leverage. Let's delve into each aspect.
Defining Key Terms
To speak the language of lever arms fluently, we must first define our terms.
-
Lever Arm: This is the rigid object – a bar, a beam, or even a bone in your body – that transmits and amplifies force. Its primary function is to provide a structure upon which the effort and load can interact around a pivot point.
-
Fulcrum: The fulcrum is the pivot point around which the lever arm rotates. Its placement is critical, as it directly influences the mechanical advantage gained.
-
Effort: The effort (also known as the force) is the energy applied to the lever arm, initiating the movement that overcomes the load. The goal is to achieve more with less.
-
Load: The load represents the resistance or weight that we are trying to overcome. It can be anything from a stubborn bolt to a heavy object being lifted.
The Relationship Between Force, Distance, and Torque
The magic of a lever arm lies in its ability to translate a force applied over a distance into torque. Torque, in essence, is the rotational force that causes the lever arm to pivot around the fulcrum.
The length of the lever arm is a crucial factor in torque generation. A longer lever arm allows a smaller force to be applied over a greater distance from the fulcrum, resulting in a larger torque.
Imagine using a long wrench versus a short one to loosen a tight nut. The longer wrench provides a greater lever arm, allowing you to generate significantly more torque with the same amount of effort.
This relationship between force, distance, and torque is the foundation upon which the principle of mechanical advantage is built. In short, by increasing the length of the lever arm, we can effectively multiply the force we apply.
Deciphering the components of a lever arm system equips us with the vocabulary to understand how these systems function. But simply knowing the what is insufficient; we must understand the how. The magic of lever arms truly manifests in their ability to amplify force – a concept known as mechanical advantage.
The Secret Weapon: Mastering Mechanical Advantage
At the heart of a lever arm's utility lies the principle of mechanical advantage.
This is where simple tools transform into force multipliers, allowing us to accomplish feats seemingly beyond our natural capabilities. Understanding and harnessing mechanical advantage is the key to unlocking the true potential of lever arm systems.
Defining Mechanical Advantage
Mechanical advantage (MA) is, at its core, a ratio.
Specifically, it’s the ratio of the output force (the force exerted on the load) to the input force (the effort we apply).
MA is often expressed as a numerical value:
- MA > 1: Indicates force amplification, meaning the output force is greater than the input force.
- MA = 1: Indicates no force amplification; the output force equals the input force.
- MA < 1: Indicates force reduction, meaning the output force is less than the input force (often traded for increased speed or distance).
How Lever Arms Provide Mechanical Advantage
Lever arms achieve mechanical advantage by strategically manipulating the relationship between force and distance.
This manipulation revolves around the fulcrum, the pivot point of the lever.
The positioning of the fulcrum relative to the effort and the load dictates the magnitude of the mechanical advantage.
Effort Multiplication: The Power of Leverage
Imagine using a crowbar to lift a heavy rock.
The crowbar acts as the lever arm, the point where it rests on the ground near the rock is the fulcrum, the rock is the load, and your downward push on the other end of the crowbar is the effort.
By positioning the fulcrum close to the rock (the load), you create a longer effort arm (the distance from the fulcrum to where you apply force) and a shorter load arm (the distance from the fulcrum to the rock).
This configuration allows you to generate a significantly larger upward force on the rock than the force you exert on the crowbar.
This is effort multiplication in action.
The Lever Arm Length's Impact
The length of the lever arm plays a crucial role in determining the mechanical advantage.
A longer effort arm, relative to the load arm, translates to a greater mechanical advantage, allowing you to move heavier objects with less effort.
Think of opening a door.
Pushing near the hinges (short effort arm) requires significantly more force than pushing near the handle (longer effort arm).
This is because the longer effort arm provides a greater mechanical advantage.
Deciphering the components of a lever arm system equips us with the vocabulary to understand how these systems function. But simply knowing the what is insufficient; we must understand the how. The magic of lever arms truly manifests in their ability to amplify force – a concept known as mechanical advantage.
Now, let's move beyond the foundational principles. The practical application of lever arms becomes even more apparent when we explore the different classes into which they are categorized. Each class offers unique characteristics and serves specific purposes, contributing to a broad spectrum of applications we encounter daily.
Lever Arms Unveiled: Exploring the Three Classes and Their Applications
Lever arms, while sharing the same fundamental principles, are not created equal. They are categorized into three distinct classes based on the relative positioning of the fulcrum, load, and effort. Understanding these classifications unlocks a deeper comprehension of how each lever arm functions and where it is best applied.
Each class has unique characteristics, advantages, and disadvantages, making them suitable for diverse applications.
First-Class Levers: Balancing Act
In a first-class lever, the fulcrum is positioned between the effort and the load. This arrangement allows for a balanced exchange between force and distance.
Depending on the placement of the fulcrum, a first-class lever can either amplify force or increase the distance over which the load moves.
Seesaw: A Classic Example
A seesaw perfectly illustrates a first-class lever. The fulcrum is at the center, with the weight of one person acting as the load and the push of the other person acting as the effort.
Moving the fulcrum closer to one person allows them to lift a heavier person on the other side.
Crowbar: Prying Power
A crowbar is another common example. The fulcrum is a fixed point near the object being moved (the load), and the effort is applied at the opposite end.
This configuration allows a relatively small force to generate a large force to lift or pry open heavy objects.
Second-Class Levers: Force Amplification
A second-class lever is defined by having the load positioned between the fulcrum and the effort. This configuration inherently favors force amplification. The effort arm (the distance between the effort and the fulcrum) is always longer than the load arm (the distance between the load and the fulcrum).
Consequently, second-class levers always provide a mechanical advantage greater than one, making them ideal for moving heavy loads with relatively little effort.
Wheelbarrow: Effortless Transport
A wheelbarrow exemplifies the second-class lever perfectly. The wheel acts as the fulcrum, the load is placed in the barrow's container, and the effort is applied by lifting the handles.
This design allows one person to easily transport heavy loads across a construction site or garden.
Third-Class Levers: Emphasizing Speed and Range
Third-class levers differ significantly; here, the effort is positioned between the fulcrum and the load. This arrangement always results in a mechanical advantage of less than one.
While they don't amplify force, they excel at increasing the speed and range of motion. These levers are crucial where rapid movement is more important than brute strength.
Human Limbs: Agility and Dexterity
The human body extensively utilizes third-class levers. For instance, the biceps muscle flexing the forearm. The elbow joint acts as the fulcrum, the biceps muscle applying effort to the forearm (between the elbow and hand), and the load is the weight in the hand or the resistance being overcome.
This configuration allows for rapid and controlled movements, essential for everyday tasks and athletic activities.
Effort, Load, and Fulcrum: A Class-by-Class Comparison
To solidify your understanding, let's recap how the effort, load, and fulcrum interact within each lever class:
-
First-Class: The fulcrum sits in the middle, allowing for balanced force or distance amplification.
-
Second-Class: The load is in the middle, prioritizing force amplification for heavy lifting.
-
Third-Class: The effort is in the middle, emphasizing speed and range of motion.
By recognizing these arrangements, you can quickly identify the class of lever and predict its behavior in various applications. This fundamental understanding unlocks the ability to analyze and optimize lever systems for greater efficiency and effectiveness.
Lever arms are not just tools confined to the external world of machines and construction; they are integral to the very mechanics of our bodies. Our musculoskeletal system, with its intricate network of bones, muscles, and joints, functions as a sophisticated system of levers, enabling a wide range of movements, from the delicate precision of a pianist's fingers to the explosive power of a sprinter's stride. Understanding these biomechanical lever systems is crucial not only for comprehending human movement but also for optimizing athletic performance and preventing injuries.
The Body's Ingenious Design: Lever Arms in Human Biomechanics
The human body represents a remarkable feat of engineering, where lever arm principles are elegantly interwoven into its anatomical structure. Muscles provide the effort force, bones act as the lever arms, and joints serve as the fulcrums, creating a dynamic system that allows us to move, lift, and interact with our environment. Recognizing how these components interact is key to appreciating the body’s biomechanical efficiency and potential vulnerabilities.
Muscles and Bones: Nature's Lever Arms
Muscles, attached to bones via tendons, generate the force needed to initiate movement. When a muscle contracts, it pulls on the bone, causing it to rotate around the joint (the fulcrum). The effectiveness of this force depends on several factors, including the length of the lever arm (the bone), the point of muscle attachment, and the angle of pull.
For instance, consider the biceps muscle and its role in elbow flexion. The biceps muscle, attached to the radius bone (the lever arm), exerts force to lift the forearm. The elbow joint acts as the fulcrum. This configuration exemplifies a third-class lever, where the effort is between the fulcrum and the load (the weight in the hand).
Another example is the calf muscle (gastrocnemius) and its role in plantar flexion of the foot. The calf muscle attaches to the heel bone (calcaneus), which acts as the lever arm. The ankle joint serves as the fulcrum. When the calf muscle contracts, it lifts the heel, allowing us to stand on our toes or push off during walking and running.
Biomechanics and Athletic Performance
A deeper understanding of lever arm mechanics can significantly enhance athletic performance. By optimizing movement techniques to maximize mechanical advantage, athletes can generate more force with less effort, improve efficiency, and reduce the risk of injury.
For example, in baseball, a batter's swing involves a complex sequence of lever actions. The legs, torso, and arms work together to generate power, with each segment contributing to the overall force applied to the bat. Understanding the optimal angles and timing of these movements can help batters increase their bat speed and hit the ball with greater force.
In weightlifting, the principles of lever arms are even more apparent. Lifters strategically position their bodies to maximize mechanical advantage when lifting heavy weights. By keeping the weight close to the body and maintaining a stable base, they can reduce the torque required to lift the load, minimizing strain on the muscles and joints.
Injury Prevention Strategies
Conversely, a lack of understanding of lever arm principles can lead to improper movement patterns and an increased risk of injury. Overexertion, poor posture, and incorrect lifting techniques can place excessive stress on the musculoskeletal system, leading to muscle strains, joint pain, and other injuries.
For example, lifting a heavy object with a rounded back increases the lever arm length between the load and the fulcrum (the spine), placing significant stress on the lower back muscles. By bending the knees and keeping the back straight, one can reduce the lever arm length and minimize the risk of injury.
Similarly, maintaining proper posture while sitting or standing helps to align the body's lever systems, reducing strain on the muscles and joints. Ergonomic assessments and training programs can help individuals identify and correct improper movement patterns, preventing injuries and promoting overall musculoskeletal health.
Understanding how lever arms function within the human body provides valuable insights into movement, performance, and injury prevention. By applying these principles, we can optimize our physical activities, enhance athletic performance, and safeguard our musculoskeletal health for years to come.
The human body's biomechanical artistry underscores the fundamental importance of lever arms. But how can we deliberately harness these principles to amplify our efforts and boost efficiency in practical settings?
Maximize Your Leverage: Practical Tips for Power and Efficiency
Optimizing lever arm usage is about more than just understanding the theory; it's about applying that understanding to real-world scenarios to maximize force and minimize effort. This involves making smart choices about lever arm length, fulcrum placement, and minimizing energy losses due to friction. When done effectively, it can lead to dramatic improvements in productivity and performance across various fields.
Optimizing Lever Arm Usage: Key Considerations
To get the most out of a lever arm system, consider these practical tips that focus on maximizing mechanical advantage and minimizing inefficiencies.
Choosing the Right Lever Arm Length
The length of a lever arm directly influences the amount of force you need to apply to move a load. A longer lever arm provides greater mechanical advantage, meaning you can move a heavier load with less effort. However, it also requires a greater range of motion.
Consider the trade-offs involved:
- Longer Lever Arms: Ideal for tasks requiring significant force amplification with a wide range of motion.
- Shorter Lever Arms: Better suited for applications where space is limited or faster, smaller movements are needed, even if it means applying more force.
Strategic Fulcrum Placement
The position of the fulcrum is critical for maximizing mechanical advantage. The closer the fulcrum is to the load, the less effort is required to move it (in second-class levers). Conversely, in first-class levers, the positioning of the fulcrum between the load and effort determines both the magnitude and direction of the force.
Experimentation and careful calculation are often necessary to find the optimal fulcrum position for a given task.
Minimizing Friction and Other Losses
Friction is the enemy of efficiency in any lever system. Friction between the lever arm and the fulcrum, or between the load and the surface it's being moved across, can significantly reduce the mechanical advantage.
To minimize friction:
- Use lubricants to reduce friction between moving parts.
- Employ rolling elements (like wheels or bearings) to reduce sliding friction.
- Ensure surfaces are smooth and clean.
- Choose materials with low coefficients of friction.
Addressing these sources of energy loss will help to ensure that more of your effort is translated into useful work.
Real-World Examples of Enhanced Efficiency
The principles of lever arm optimization are applied across a vast range of industries and activities. Here are just a few examples:
-
Construction: Cranes utilize long booms (lever arms) and strategically placed fulcrums to lift incredibly heavy materials with relative ease. The use of pulleys (another form of lever system) further multiplies the force, enabling the construction of massive structures.
-
Manufacturing: Assembly lines often incorporate lever-based tools for tasks requiring precise force application. These tools are designed to minimize operator fatigue and increase production speed.
-
Sports: In sports like baseball or golf, the athlete's limbs act as levers. Optimizing technique to maximize the length and efficiency of these lever systems is crucial for generating power and achieving peak performance. Consider the difference in power between a short, compact swing and a full, extended swing.
-
Everyday Life: Simple tools like bottle openers, nutcrackers, and even door handles are all examples of lever arms in action. Understanding how these tools work allows you to use them more efficiently and effectively.
By understanding and applying these practical tips, you can harness the power of lever arms to amplify your efforts, reduce strain, and achieve greater efficiency in a wide variety of tasks. The key is to carefully consider the specific requirements of the job at hand and to optimize the lever system accordingly.
Video: Lever Arm Secrets: Unleash Power & Efficiency Now!
Lever Arm Secrets: Your Burning Questions Answered
Understanding lever arms is key to maximizing power and efficiency. Here are some common questions to help clarify the concepts.
What exactly is a lever arm, in simple terms?
Think of a lever arm as the distance from the pivot point (or fulcrum) to the point where you apply force. The longer the lever arm, the more leverage you have, allowing you to exert more force with less effort.
How does a longer lever arm increase efficiency?
A longer lever arm provides a mechanical advantage. It allows you to move or lift heavier objects, or generate more rotational force, with the same amount of energy input. This translates directly into increased efficiency.
Can a lever arm be too long? What are the drawbacks?
While a longer lever arm increases force, it also requires a greater distance of movement. A very long lever arm might be impractical in certain situations due to space constraints or the amount of travel needed to achieve the desired effect.
How does the lever arm principle apply to everyday life?
The lever arm principle is at work everywhere! Think of using a wrench to tighten a bolt, opening a door with a doorknob, or even paddling a canoe. Each of these involves using a lever arm to amplify your force and make the task easier.