Laser Wavelength Chart: Your Safe Use Guide
Laser safety standards, as meticulously outlined by organizations like the American National Standards Institute (ANSI), are inextricably linked to the specific emissions detailed in a laser wavelength chart. The wavelength of a laser beam, a critical attribute, dictates its interaction with various materials, including human tissue. Understanding the information presented in a laser wavelength chart is, therefore, paramount for professionals in fields such as medicine and manufacturing, where lasers of varying wavelengths are routinely employed. A comprehensive laser wavelength chart serves as an essential reference tool, enabling the selection of appropriate safety measures and personal protective equipment (PPE) necessary to mitigate the risks associated with exposure to specific laser radiation.
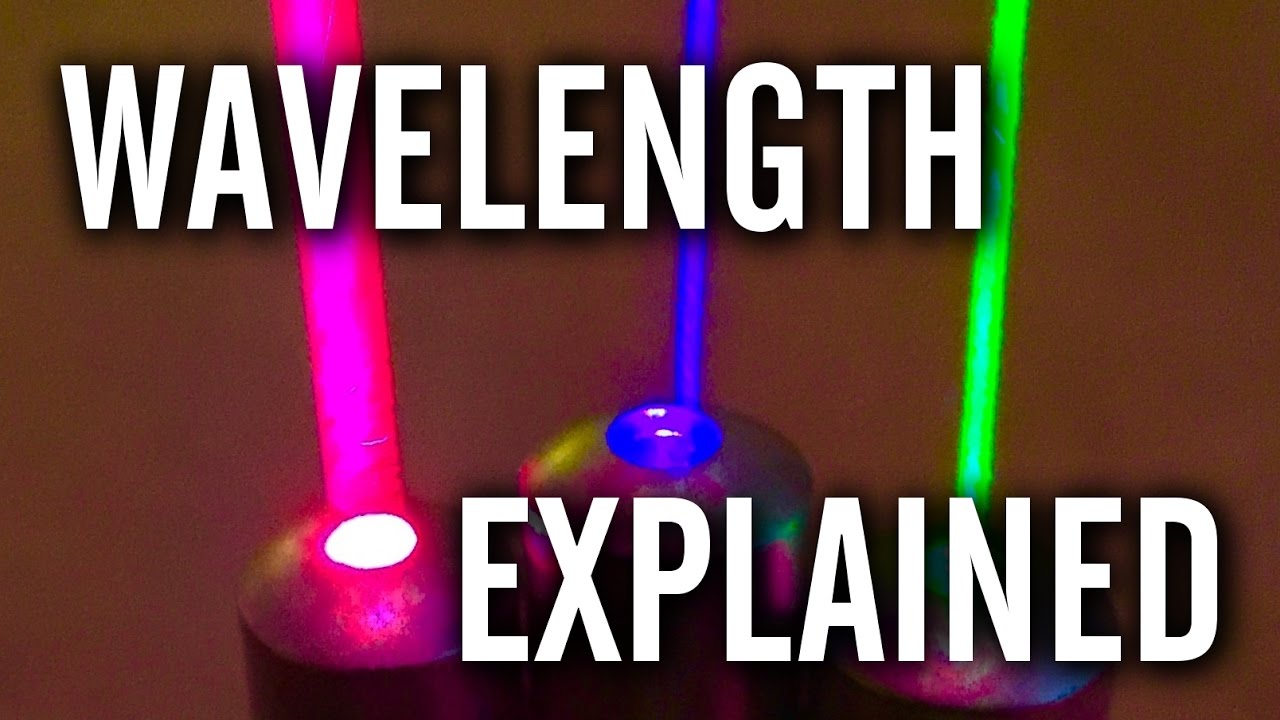
Image taken from the YouTube channel XM360 , from the video titled Lasers - Wavelength (nm) Explained .
Unveiling the World of Laser Radiation
Laser radiation, an indispensable element of modern science and technology, stands apart from conventional light sources due to its unique properties and wide-ranging applications.
This introduction serves as a foundational exploration of laser radiation, providing a glimpse into its fundamental characteristics and the critical role it plays across various disciplines. It also emphasizes the essential aspect of laser safety.
Defining Laser Radiation and its Key Characteristics
At its core, laser radiation is a form of electromagnetic radiation produced through a process called light amplification by stimulated emission of radiation. This acronym, LASER, aptly describes the mechanism behind its generation.
Unlike ordinary light, laser radiation possesses several distinctive features:
-
Monochromaticity: It consists of a narrow range of wavelengths, resulting in a highly pure color.
-
Coherence: The photons within the laser beam are in phase, leading to constructive interference and a highly focused beam.
-
Collimation: Laser beams exhibit minimal divergence, enabling them to travel long distances without significant spreading.
A Broad Spectrum of Applications
Lasers have transcended their initial perception as laboratory curiosities and have become integral components in a vast array of applications. From the precision of surgical procedures to the accuracy of industrial manufacturing, lasers have revolutionized numerous fields:
-
Medicine: Lasers are used in eye surgery (LASIK), cosmetic procedures, and cancer treatment.
-
Industry: They are employed for cutting, welding, marking, and engraving materials.
-
Telecommunications: Lasers are essential for transmitting data through fiber optic cables.
-
Scientific Research: Lasers are indispensable tools for spectroscopy, microscopy, and advanced materials research.
-
Consumer Electronics: Lasers power barcode scanners, DVD players, and laser printers.
The Paramount Importance of Laser Safety
While the benefits of laser technology are undeniable, it is crucial to acknowledge the potential hazards associated with laser radiation. High-powered lasers can cause severe eye and skin damage.
Therefore, adherence to laser safety protocols is of utmost importance to prevent accidents and protect personnel working with or around lasers. This includes the use of appropriate laser safety eyewear, implementation of engineering controls, and comprehensive training programs.
Blog Post Structure: A Roadmap
This blog post is structured to provide a comprehensive understanding of laser radiation, progressing from fundamental principles to practical applications and safety considerations. Subsequent sections will delve into:
-
The fundamental properties of laser radiation (wavelength, frequency, and photon energy).
-
The unique characteristics of laser beams (coherence, collimation, and power).
-
An overview of different types of lasers (HeNe, Argon-ion, CO2, etc.).
-
A detailed examination of laser applications across various industries.
-
A comprehensive guide to laser safety protocols and best practices.
-
Laser Diagnostics and Measurement strategies and technology.
Finally, the conclusion will summarize the key takeaways and offer a glimpse into the future of laser technology.
Fundamentals of Laser Radiation: The Building Blocks
Understanding the fundamental properties of laser radiation is essential for appreciating its diverse applications and interactions. Laser light, unlike ordinary light, exhibits unique characteristics rooted in its coherent and monochromatic nature. These properties are governed by the underlying physics of wavelength, frequency, and photon energy, all intricately linked within the electromagnetic spectrum.
Wavelength: The Color of Light
Wavelength, denoted by the Greek letter lambda (λ), defines the distance between two successive crests or troughs of a wave. In the context of laser radiation, wavelength determines the color of the light.
It is typically measured in nanometers (nm) or micrometers (µm).
A laser emitting light with a wavelength of 632.8 nm appears red, while a laser emitting light at 532 nm appears green. Different laser types are designed to emit specific wavelengths based on their lasing medium and cavity configuration. Controlling wavelength is crucial for applications ranging from barcode scanning to medical treatments.
Frequency: The Rate of Oscillation
Frequency (ν) represents the number of wave cycles that pass a given point per unit time. It is inversely proportional to wavelength, meaning that as wavelength increases, frequency decreases, and vice versa. This relationship is mathematically expressed as:
c = λν,
where c is the speed of light in a vacuum (approximately 3 x 108 meters per second).
The frequency of laser radiation, like its wavelength, is a defining characteristic. It dictates how the laser interacts with matter.
Electromagnetic Spectrum: A Broader Perspective
The electromagnetic spectrum encompasses the entire range of electromagnetic radiation, from low-frequency radio waves to high-frequency gamma rays. Laser radiation occupies a specific portion of this spectrum, spanning from the ultraviolet (UV) to the infrared (IR) regions.
Ultraviolet (UV) Radiation
UV radiation has shorter wavelengths and higher frequencies than visible light. UV lasers are used in various applications, including:
- Photolithography.
- Sterilization.
- Medical treatments.
However, UV radiation can be harmful to living tissue, necessitating strict safety measures.
Visible Light
Visible light is the portion of the electromagnetic spectrum that the human eye can detect. Lasers emitting visible light are widely used in:
- Displays.
- Pointers.
- Optical instruments.
The perceived color of visible laser light depends directly on its wavelength.
Infrared (IR) Radiation
IR radiation has longer wavelengths and lower frequencies than visible light. IR lasers are used in:
- Telecommunications.
- Industrial heating.
- Night vision applications.
While not visible to the naked eye, IR lasers can still pose safety hazards, particularly to the eyes.
Photon Energy: The Quantum Nature of Light
Photon energy (E) represents the energy carried by a single photon of light. It is directly proportional to frequency and inversely proportional to wavelength, described by the equation:
E = hν = hc/λ,
where h is Planck's constant (approximately 6.626 x 10-34 Joule-seconds).
Higher frequency (shorter wavelength) laser radiation carries more energy per photon than lower frequency (longer wavelength) radiation. This is crucial for understanding laser-material interactions.
The energy of the laser photons must be high enough to induce a photochemical change in an analyte for an absorption process to occur.
For example, UV lasers, with their high photon energies, are capable of breaking chemical bonds, a phenomenon utilized in laser ablation and UV curing processes.
Laser Beam Properties: What Makes Lasers Special
Understanding the fundamental properties of laser radiation is essential for appreciating its diverse applications and interactions. Laser light, unlike ordinary light, exhibits unique characteristics rooted in its coherent and monochromatic nature. These properties are governed by the underlying physics of stimulated emission and resonant cavities, ultimately defining how a laser beam interacts with the world.
Coherence: The Essence of Order
Coherence, in the context of laser beams, refers to the degree of phase correlation among the photons within the beam. A highly coherent laser beam exhibits photons that are synchronized in both space and time.
This synchronization translates to the ability to focus the beam to a very small spot, concentrating its power. High coherence is paramount in applications like laser cutting, precision surgery, and holography.
Furthermore, the degree of coherence directly influences the interference properties of laser light. Highly coherent sources are capable of generating strong and well-defined interference patterns. This phenomenon is utilized in interferometry for precise measurements of distances, surface profiles, and refractive indices.
Collimation: Traveling the Distance
Collimation describes the degree to which a beam remains parallel as it propagates through space. An ideally collimated beam would maintain its initial diameter indefinitely. However, in reality, all beams exhibit some degree of divergence.
The impact of collimation on beam propagation is substantial. A well-collimated laser beam can travel long distances without significant spreading, making it suitable for applications like laser rangefinding, satellite communication, and barcode scanning.
The divergence of a laser beam is intrinsically linked to the wavelength of the light and the diameter of the beam at its source. Achieving a high degree of collimation typically requires careful optical design and beam shaping techniques.
Beam Divergence: Quantifying Spread
Beam divergence is the gradual increase in beam diameter as it propagates away from its source. It's typically measured in milliradians (mrad) or degrees.
The relationship between collimation and beam divergence is inherently inverse. A beam with low divergence is considered well-collimated, and vice versa.
Beam divergence is a crucial parameter to consider when selecting a laser for a specific application. In applications where a tight focus or long-range propagation is required, minimizing beam divergence is essential. Optical elements, such as lenses and mirrors, can be used to manipulate beam divergence and shape the beam profile.
Power and Energy: Quantifying Laser Output
Laser power refers to the rate at which energy is delivered by the laser beam. It's measured in Watts (W), where one Watt is equal to one Joule per second.
Laser energy, on the other hand, represents the total amount of energy delivered by a laser pulse. It's measured in Joules (J).
The choice between a high-power continuous wave (CW) laser and a high-energy pulsed laser depends on the specific application. CW lasers are suitable for continuous processing like cutting and welding, while pulsed lasers are utilized for applications requiring high peak intensities, such as laser ablation and nonlinear optics. The average power of a pulsed laser is equal to its energy per pulse times the number of pulses per second.
Interaction with Matter: Absorption, Transmission, and Reflection Spectra
When a laser beam interacts with matter, the outcome is governed by the absorption, transmission, and reflection characteristics of the material at the laser's wavelength. These characteristics are summarized in what is known as the Absorption, Transmission, and Reflection spectra.
The absorption spectrum of a material describes the fraction of incident light that is absorbed as a function of the wavelength. The amount of light that is absorbed depends on the chemical properties and molecular composition of the substance.
The transmission spectrum of a material describes the fraction of incident light that is transmitted as a function of the wavelength. Transmitted light passes straight through the material.
The reflection spectrum of a material describes the fraction of incident light that is reflected as a function of the wavelength. Reflected light bounces off of the material.
These spectra are critical for predicting how a particular laser will interact with a given material. For example, a laser used for laser-induced breakdown spectroscopy (LIBS) will emit radiation at a wavelength with a high absorption spectrum. Similarly, if you wanted to block a particular radiation frequency, you could use an optical filter that transmits all other frequencies except for the frequency that you want to block (i.e. low transmission spectrum). These spectra also find practical applications in laser safety.
Types of Lasers: A Diverse Family
Understanding the fundamental properties of laser radiation is essential for appreciating its diverse applications and interactions. Laser light, unlike ordinary light, exhibits unique characteristics rooted in its coherent and monochromatic nature. These properties are governed by the underlying physical principles and the specific lasing medium employed. This section explores the diverse family of lasers, categorized by their lasing medium, emission wavelength, and common applications, providing a comprehensive overview of the laser landscape.
Gas Lasers
Gas lasers were among the earliest types of lasers developed and continue to find use in a variety of applications. These lasers use a gas mixture as their active medium and are typically excited by an electrical discharge.
Helium-Neon (HeNe) Laser
The Helium-Neon (HeNe) laser is a classic example of a gas laser, recognized for its stable and coherent red light output at 632.8 nm. The lasing medium consists of a mixture of helium and neon gas. Helium atoms are excited by an electrical discharge and then transfer their energy to neon atoms, which subsequently emit laser light.
HeNe lasers are commonly used in alignment, barcode scanners, and educational demonstrations due to their relatively low cost and high beam quality.
Argon-Ion Laser
Argon-Ion lasers utilize ionized argon gas as the lasing medium, producing intense blue and green light emissions at 488 nm and 514.5 nm, respectively. These lasers require a high current electrical discharge to ionize the argon gas.
Argon-Ion lasers are employed in confocal microscopy, flow cytometry, and laser light shows due to their high output power in the visible spectrum.
Krypton-Ion Laser
Krypton-Ion lasers, similar to argon-ion lasers, use ionized krypton gas as the lasing medium. They are capable of producing multiple wavelengths of light, including red, yellow, and green, making them versatile sources for applications requiring multiple colors.
Krypton-Ion lasers find applications in laser displays, scientific research, and medical treatments where specific wavelengths are needed.
Carbon Dioxide (CO2) Laser
Carbon Dioxide (CO2) lasers are high-power gas lasers that emit far-infrared radiation at 10.6 µm. The lasing medium consists of a mixture of carbon dioxide, nitrogen, and helium.
CO2 lasers are widely used in industrial applications such as cutting, welding, and engraving of various materials due to their high power and efficiency.
Solid-State Lasers
Solid-state lasers utilize a solid material as the lasing medium, typically a crystal or glass doped with rare-earth ions. These lasers are known for their high power and efficiency.
Nd:YAG Laser
The Nd:YAG (Neodymium-doped Yttrium Aluminum Garnet) laser is a widely used solid-state laser that emits near-infrared radiation at 1064 nm. The lasing medium is a YAG crystal doped with neodymium ions.
Nd:YAG lasers are employed in material processing, medical procedures, and military applications due to their high power and good beam quality.
Nd:Glass Laser
Nd:Glass lasers are similar to Nd:YAG lasers but use a glass host material doped with neodymium ions. They also emit near-infrared radiation at around 1060 nm.
Nd:Glass lasers are often used in high-energy pulsed laser systems due to their ability to store large amounts of energy.
Titanium-Sapphire (Ti:Sapphire) Laser
Titanium-Sapphire (Ti:Sapphire) lasers utilize a sapphire crystal doped with titanium ions as the lasing medium. These lasers are known for their broad tunability in the near-infrared range, typically from 650 to 1100 nm.
Ti:Sapphire lasers are essential tools in scientific research, particularly in ultrafast spectroscopy and microscopy, where tunable and short-pulse lasers are required.
Excimer Lasers
Excimer lasers are a type of gas laser that utilizes noble gas halides as the lasing medium. These lasers emit ultraviolet (UV) light.
Excimer Laser (e.g., ArF at 193 nm)
Excimer lasers use a mixture of a noble gas (such as argon, krypton, or xenon) and a reactive gas (such as fluorine or chlorine) to form an excimer molecule. A common example is the ArF excimer laser, which emits UV light at 193 nm.
Excimer lasers are used in photolithography for semiconductor manufacturing, LASIK eye surgery, and scientific research due to their ability to precisely ablate materials.
Semiconductor Lasers
Semiconductor lasers, also known as laser diodes, are compact and efficient lasers that use a semiconductor material as the lasing medium.
Diode Laser (Laser Diode)
Diode lasers are available in various wavelengths, ranging from the visible to the infrared spectrum. They are widely used in telecommunications, barcode scanners, laser pointers, and optical storage devices. The specific wavelength depends on the semiconductor material used.
The versatility, compactness, and low cost of laser diodes make them ubiquitous in modern technology.
Fiber Lasers
Fiber lasers use an optical fiber doped with rare-earth elements as the lasing medium. These lasers offer high beam quality, high efficiency, and excellent thermal management.
Fiber Laser
Fiber lasers are typically pumped by diode lasers, and the resulting laser light is confined and amplified within the fiber. They are commonly available in the near-infrared region, but other wavelengths can be achieved through nonlinear optical processes.
Fiber lasers are used in material processing, telecommunications, and medical applications due to their high power and excellent beam quality.
Dye Lasers
Dye lasers utilize organic dyes dissolved in a liquid solvent as the lasing medium. These lasers are known for their broad tunability, allowing for the generation of light at various wavelengths.
Dye Laser
Dye lasers can be tuned across a wide range of wavelengths by changing the dye or adjusting the optical components within the laser cavity.
Dye lasers are used in spectroscopy, photochemistry, and medical applications where tunable laser light is required.
Quantum Cascade Lasers (QCLs)
Quantum Cascade Lasers (QCLs) are semiconductor lasers that emit mid- to far-infrared radiation. Unlike traditional semiconductor lasers, QCLs utilize intersubband transitions in quantum wells to generate laser light.
Quantum Cascade Laser (QCL)
QCLs offer unique capabilities for spectroscopy, chemical sensing, and industrial process control in the mid- to far-infrared region, where many molecules have strong absorption features.
The diverse family of lasers reflects the ongoing innovation in photonics and the expanding applications of laser technology across various fields.
Laser Applications: A World of Possibilities
Understanding the fundamental properties of laser radiation is essential for appreciating its diverse applications and interactions. Laser light, unlike ordinary light, exhibits unique characteristics rooted in its coherent and monochromatic nature. These properties are governed by the underlying physical principles that make lasers invaluable across numerous fields. This section examines the extensive applications of lasers, highlighting their versatility and profound impact on modern technology, medicine, industry, and scientific research.
Lasers in Medicine: Precision and Innovation
Medical applications of lasers have revolutionized various treatments and procedures, offering precision and minimizing invasiveness. Lasers are employed in a wide array of medical specialties, each leveraging the unique properties of laser light for specific therapeutic effects.
Surgical Applications
In surgery, lasers offer unparalleled precision. They can selectively ablate tissue while minimizing damage to surrounding areas. Lasers are commonly used in procedures such as:
- LASIK Eye Surgery: To reshape the cornea and correct vision.
- Tumor Removal: Precise ablation of cancerous tissue.
- Vascular Surgery: Sealing blood vessels to reduce bleeding.
Dermatology
Dermatologists utilize lasers for a range of cosmetic and therapeutic applications. These include:
- Laser Hair Removal: Selectively targeting melanin in hair follicles.
- Acne Treatment: Reducing inflammation and bacteria.
- Scar Reduction: Smoothing and resurfacing skin.
- Treatment of Vascular Lesions: Targeting and destroying blood vessels.
Ophthalmology
Lasers have transformed ophthalmology, offering precise and effective treatments for various eye conditions.
- Retinal Detachment Repair: Sealing retinal tears and reattaching the retina.
- Glaucoma Treatment: Reducing intraocular pressure.
- Cataract Surgery: Using femtosecond lasers to assist in precise lens removal.
Dentistry
In dentistry, lasers offer advantages such as reduced pain, less bleeding, and increased precision.
- Cavity Detection: Early detection of tooth decay.
- Gum Disease Treatment: Removing infected tissue.
- Teeth Whitening: Activating whitening agents for faster results.
Industrial Applications: Precision Manufacturing
Lasers play a pivotal role in numerous industrial processes, providing precision, efficiency, and versatility. Their ability to deliver focused energy makes them ideal for a wide range of manufacturing applications.
Cutting and Welding
Lasers are widely used for cutting and welding materials with high precision and minimal heat-affected zones.
- Laser Cutting: Cutting metals, plastics, and composites.
- Laser Welding: Joining materials with high strength and precision.
Marking and Engraving
Lasers can create permanent markings and engravings on a variety of materials.
- Laser Marking: Applying codes, serial numbers, and logos.
- Laser Engraving: Creating detailed designs on metal, wood, and glass.
Lasers in Science: Discovery and Analysis
Scientific applications of lasers have propelled research across various disciplines, enabling precise measurements and innovative experiments. Lasers are used in spectroscopy, microscopy, and numerous other research areas.
Spectroscopy
Spectroscopy utilizes lasers to analyze the interaction of light with matter, providing valuable information about the composition and structure of materials.
- Raman Spectroscopy: Identifying molecular structures.
- Absorption Spectroscopy: Measuring the absorption of light by a substance.
- Laser-Induced Breakdown Spectroscopy (LIBS): Analyzing elemental composition.
Microscopy
Lasers enhance the capabilities of microscopes, enabling high-resolution imaging and analysis of biological and material samples.
- Confocal Microscopy: Creating three-dimensional images of cells and tissues.
- Two-Photon Microscopy: Imaging deeper into tissues with reduced phototoxicity.
Laser Pointers: A Ubiquitous Tool
Laser pointers, although seemingly simple, are an accessible application of laser technology.
Varieties and Uses
Available in various colors (red, green, blue), they function primarily as pointing devices. However, higher-powered laser pointers can present safety hazards.
- Presentations: Highlighting key points in presentations.
- Astronomy: Pointing out stars and constellations.
- Educational Tools: Demonstrating scientific concepts.
The applications of lasers are vast and continue to expand as technology advances. From life-saving medical procedures to precision manufacturing and groundbreaking scientific research, lasers have become indispensable tools. Understanding their capabilities and safety considerations is crucial for harnessing their full potential.
Laser Safety: Protecting Yourself and Others
Understanding the fundamental properties of laser radiation is essential for appreciating its diverse applications and interactions. Laser light, unlike ordinary light, exhibits unique characteristics rooted in its coherent and monochromatic nature. These properties are governed by the underlying physical principles discussed earlier in this blog. However, with great power comes great responsibility, and the use of lasers necessitates a thorough understanding and implementation of stringent safety protocols. Ensuring the well-being of individuals working with or around lasers is paramount, requiring a multi-faceted approach encompassing administrative controls, engineering safeguards, and the consistent use of personal protective equipment. This section delves into the core elements of laser safety, providing an overview of the measures necessary to mitigate risks and prevent laser-related injuries.
The Central Role of the Laser Safety Officer (LSO)
The Laser Safety Officer (LSO) stands as the cornerstone of any effective laser safety program.
This individual shoulders the primary responsibility for ensuring a safe working environment.
The LSO's duties extend to:
- Conducting hazard analyses.
- Implementing control measures.
- Providing comprehensive laser safety training.
- Monitoring compliance with relevant safety standards and regulations.
The LSO also acts as a vital liaison, bridging the gap between management, laser users, and regulatory bodies.
The LSO has the authority to suspend, restrict, or terminate laser operations if it is deemed that hazards are not controlled.
Laser Safety Eyewear: The First Line of Defense
Laser safety eyewear is an indispensable component of laser safety.
Different lasers emit light at specific wavelengths, each possessing a unique energy level and potential for harm.
Standard safety glasses are often inadequate for protection.
Laser safety eyewear is specifically designed to attenuate or block particular wavelengths of light.
The selection of appropriate eyewear is based on the laser's wavelength, power, and potential exposure duration.
Using the wrong type of eyewear can provide a false sense of security and may result in severe eye damage.
Navigating the Landscape of Laser Safety Standards and Regulations
Multiple organizations play crucial roles in establishing and enforcing laser safety standards worldwide:
American National Standards Institute (ANSI)
In the United States, the American National Standards Institute (ANSI) publishes the ANSI Z136 series of laser safety standards.
These standards provide comprehensive guidance on the safe use of lasers in various settings.
ANSI standards are widely adopted and serve as a benchmark for laser safety practices.
International Electrotechnical Commission (IEC)
The International Electrotechnical Commission (IEC) develops international standards for laser safety.
These standards, such as IEC 60825, are recognized and implemented globally.
They provide a framework for ensuring consistency in laser safety practices across different countries.
Center for Devices and Radiological Health (CDRH) - US FDA
The Center for Devices and Radiological Health (CDRH), a division of the U.S. Food and Drug Administration (FDA), regulates laser products sold in the United States.
The CDRH enforces regulations pertaining to laser product performance, labeling, and safety features.
Manufacturers must comply with CDRH regulations to ensure that their laser products meet minimum safety requirements.
Understanding Laser Hazard Classification
Lasers are classified into different classes based on their potential hazards:
- Class 1 Lasers: Considered inherently safe under normal operating conditions.
- Class 2 Lasers: Emit visible light and are safe for momentary viewing due to the blink reflex.
- Class 3R Lasers: Potentially hazardous but with limited power.
- Class 3B Lasers: Can cause serious eye injury if directly viewed.
- Class 4 Lasers: The most hazardous class, capable of causing eye and skin damage and potentially igniting fires.
This classification system provides a framework for assessing the risks associated with different laser systems.
Quantifying Risk: Maximum Permissible Exposure (MPE) and Nominal Hazard Zone (NHZ)
Two critical concepts in laser safety are Maximum Permissible Exposure (MPE) and Nominal Hazard Zone (NHZ):
- Maximum Permissible Exposure (MPE): The maximum level of laser radiation to which a person can be exposed without adverse health effects. MPE values are wavelength-dependent and vary based on exposure duration.
- Nominal Hazard Zone (NHZ): The area around a laser where the level of laser radiation exceeds the MPE. The NHZ defines the space where specific safety controls are required.
Implementing Engineering Controls: Building Safety into the System
Engineering controls involve incorporating physical safeguards into the laser system or the surrounding environment.
Examples include:
- Interlocks that automatically shut off the laser when a protective barrier is removed.
- Enclosures that prevent access to the laser beam.
- Beam traps that absorb or deflect laser radiation.
- Remote Interlock Connectors that can connect an external switch to the laser (typically in emergency stop situations.)
Engineering controls provide a reliable and passive means of risk mitigation.
Administrative Controls: Establishing Safe Practices
Administrative controls encompass policies, procedures, and training programs designed to promote laser safety.
Key elements include:
- Standard operating procedures (SOPs) that outline safe work practices.
- Laser safety training for all personnel working with or around lasers.
- Warning signs and labels that clearly identify laser hazards.
- Controlling access to laser areas.
Effective administrative controls rely on consistent implementation and adherence.
Personal Protective Equipment (PPE): A Critical Layer of Defense
Personal Protective Equipment (PPE) serves as an additional layer of protection against laser hazards.
- Laser safety eyewear is the most critical form of PPE for preventing eye injuries.
- Gloves can protect the skin from exposure to high-power laser beams.
- Flame-resistant clothing may be necessary in situations where there is a risk of fire.
The selection and use of appropriate PPE are essential for minimizing the risk of injury.
The Importance of Regular Laser Safety Audits
Regular laser safety audits are essential for verifying the effectiveness of safety programs and identifying potential areas for improvement.
Audits should be conducted by qualified personnel and should assess all aspects of laser safety.
Findings from audits should be documented and used to implement corrective actions.
Laser Safety Training Programs: Empowering Personnel Through Knowledge
Comprehensive laser safety training programs are vital for educating personnel about laser hazards and safety measures.
Training should cover:
- Laser safety principles.
- Hazard identification.
- Control measures.
- Emergency procedures.
Effective training empowers individuals to work safely with lasers and to recognize and respond to potential hazards.
Laser Diagnostics and Measurement: Understanding Laser Output
Understanding the fundamental properties of laser radiation is essential for appreciating its diverse applications and interactions. Laser light, unlike ordinary light, exhibits unique characteristics rooted in its coherent and monochromatic nature. These properties are governed by the underlying physical principles and require specialized tools for accurate measurement and characterization. Laser diagnostics and measurement techniques play a crucial role in ensuring laser performance, safety, and the optimization of laser applications across various fields.
This section outlines the methods and instruments used to characterize and measure laser radiation.
Spectrometers: Analyzing the Wavelength Composition
Spectrometers are indispensable tools for analyzing the spectral composition of laser light. These instruments separate incoming light into its constituent wavelengths, allowing for precise determination of the laser's output spectrum.
Spectrometers operate based on principles of diffraction or refraction, using components such as prisms or diffraction gratings to disperse light according to wavelength. This dispersed light is then detected by an array of sensors, providing a detailed profile of the laser's spectral distribution.
The resulting spectrum reveals critical information about the laser's performance, including its central wavelength, spectral bandwidth, and the presence of any unwanted spectral components or impurities. This information is vital for ensuring that the laser operates within specified parameters and for troubleshooting any spectral anomalies that may arise.
Optical Power Meters: Measuring Laser Beam Power
Optical power meters are fundamental instruments for quantifying the power of a laser beam. They provide a direct measurement of the amount of energy delivered by the laser per unit of time, typically expressed in Watts (W) or milliwatts (mW).
These meters utilize various detection mechanisms, such as thermal detectors or photodiodes, to convert the incident laser light into an electrical signal proportional to the beam's power. The electrical signal is then processed and displayed as a numerical reading, providing a precise measurement of the laser's output power.
Accurate power measurement is crucial for monitoring laser performance over time, ensuring that the laser delivers the required power for its intended application, and verifying compliance with safety standards. Regular power measurements can also help detect any degradation or malfunction of the laser system.
Online Databases of Laser Wavelengths: A Quick Reference Guide
Online databases of laser wavelengths serve as valuable resources for quickly obtaining information about the spectral characteristics of various laser types. These databases compile comprehensive data on the wavelengths emitted by different lasing media, including common and less common laser transitions.
They offer a convenient way to access critical spectral information, aiding in the selection of appropriate lasers for specific applications, the design of optical systems compatible with particular laser wavelengths, and the identification of potential spectral interferences. These resources are regularly updated with new laser types and spectral data, ensuring their accuracy and relevance.
Optical Filters: Selecting and Blocking Specific Wavelengths
Optical filters are essential components in laser systems for selectively transmitting or blocking specific wavelengths of light. These filters are designed to manipulate the spectral content of laser beams, allowing for precise control over the wavelengths that are allowed to pass through.
They are fabricated using various materials and techniques, including thin-film coatings and colored glass, to achieve the desired spectral transmission characteristics.
Optical filters are used for a wide range of applications, including laser beam cleanup, spectral filtering, fluorescence excitation, and the suppression of unwanted background light. By carefully selecting the appropriate filters, it is possible to optimize the performance of laser systems and enhance the quality of laser-based measurements.
Video: Laser Wavelength Chart: Your Safe Use Guide
Frequently Asked Questions
Why is understanding laser wavelength important for safety?
Laser wavelength directly affects how deeply a laser penetrates tissues. Different wavelengths are absorbed differently by the eyes and skin. A laser wavelength chart helps you determine the potential hazards of a particular laser and the appropriate safety measures needed to protect yourself.
What information does a laser wavelength chart provide?
A laser wavelength chart typically shows the range of wavelengths emitted by different types of lasers and how those wavelengths interact with various materials, including human tissue. This information is crucial for selecting appropriate laser safety eyewear.
How do I use a laser wavelength chart to choose the correct laser safety glasses?
The laser wavelength chart will identify the specific wavelengths your laser emits. Look for safety glasses that are rated to block or attenuate those particular wavelengths. The chart will also offer information on what the optimal optical density (OD) would be for that specific laser application.
Where can I find a reliable laser wavelength chart?
Reputable sources for a laser wavelength chart include laser safety equipment manufacturers, industry-specific organizations (e.g., laser surgery associations), and scientific publications that deal with laser safety. Always confirm that the chart is up-to-date and relevant to the lasers you are working with.
So, there you have it! Hopefully, this breakdown of the laser wavelength chart helps you understand the science a little better and, more importantly, stay safe while working with lasers. Remember, knowledge is power, especially when dealing with light as potent as laser beams. Stay informed, be cautious, and keep that laser wavelength chart handy!