Heat and Temperature: The Shocking Difference You Didn't Know
Thermodynamics, the study of energy transfer, heavily relies on understanding heat and temperature. The kinetic energy of molecules, often measured using a thermometer, is closely associated with both concepts, though they are distinct. Even the National Institute of Standards and Technology (NIST) invests resources into precisely defining units to measure heat and temperature accurately. Understanding how these seemingly related ideas differ is foundational in many scientific and engineering disciplines, helping us grasp the fundamental nature of energy transfer.
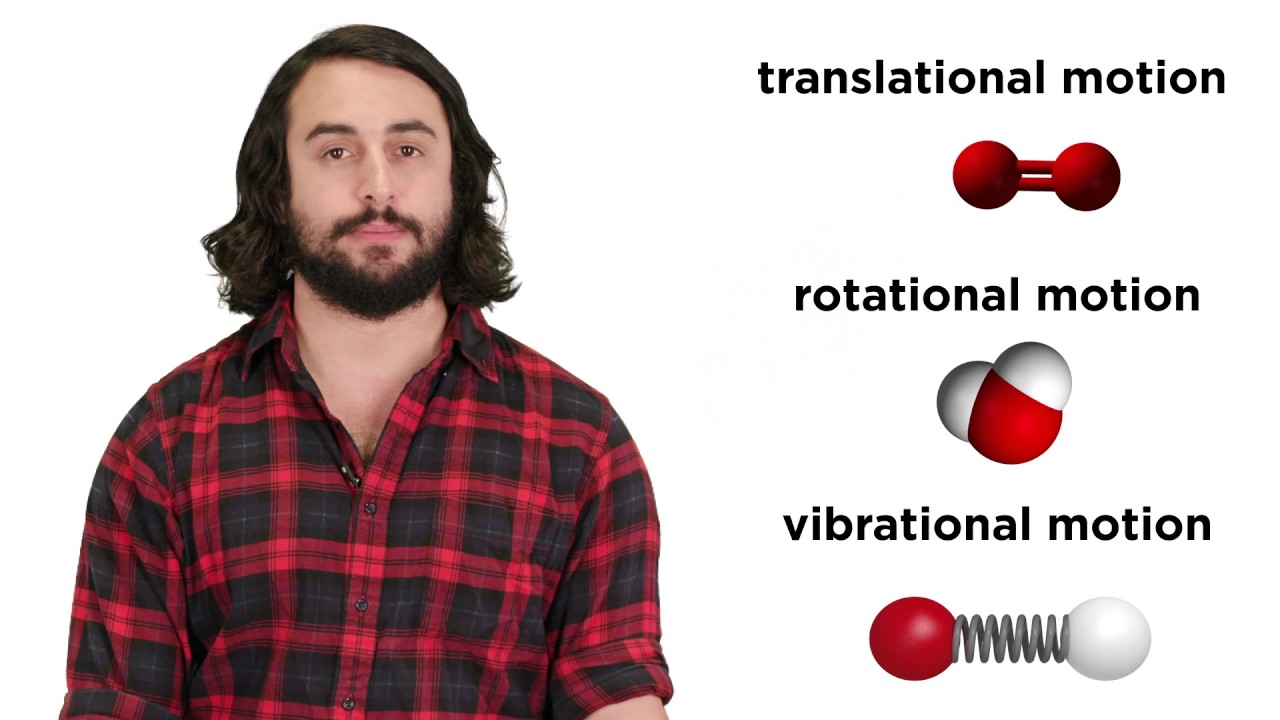
Image taken from the YouTube channel Professor Dave Explains , from the video titled Heat and Temperature .
Decoding the Mystery of Heat and Temperature
We've all been there: reaching for a pan on the stove and declaring, "That's hot!" Or shivering on a winter day, proclaiming, "It's freezing!" These everyday statements, while perfectly understandable, often blur the crucial distinction between heat and temperature. This casual conflation, though commonplace, obscures a deeper understanding of the physical world around us.
The Common Misconception
Think about a swimming pool in the summer. The water might feel cool even on a scorching day. Conversely, a tiny sparkler, although small, can feel intensely hot if it lands on your skin.
This difference arises not just from temperature alone, but also from the amount of energy transferred – which is heat. We intuitively associate "hot" with high temperature, but this is only part of the story.
Why Understanding the Difference Matters
Differentiating between heat and temperature isn't merely a matter of semantics. This understanding unlocks our ability to accurately describe, predict, and control a vast range of phenomena.
From designing efficient engines to understanding climate change, grasping the nuances of these concepts is paramount. It's essential for engineers, scientists, and anyone seeking a more profound understanding of how energy shapes our world.
Consider the design of a car engine. Engineers must manage both the temperature of the engine components to prevent overheating and the heat generated by combustion to maximize efficiency.
Ignoring the distinction would lead to catastrophic design flaws.
Thesis: Unraveling the Interplay
Heat and temperature are undeniably related. Temperature is an indicator of how fast the molecules inside something are moving. Yet, they are distinct concepts with significant implications for understanding the world around us.
Temperature describes the average energy of molecular motion. Heat, on the other hand, refers to the transfer of energy.
This distinction has far-reaching consequences, impacting everything from cooking to climate science.
By exploring the intricacies of heat and temperature, we can gain a powerful lens through which to view the universe and our place within it. Understanding these concepts opens doors to greater innovation and critical thinking.
Temperature: Gauging the Speed of Molecules
Having established the fundamental difference between heat and temperature, it's time to zoom in on temperature itself. What exactly is temperature? It's more than just a number on a thermometer; it's a window into the microscopic world.
At its core, temperature is a measure of the average kinetic energy of the molecules within a substance. The faster these molecules are zipping around, vibrating, and rotating, the higher the temperature.
Defining Temperature: The Average Kinetic Energy
Imagine a bustling city street. People are walking, running, some are standing still. Temperature is akin to calculating the average speed of all those people.
Some individuals are moving quickly, others slowly, but the average gives you a sense of the overall activity level on the street. Similarly, temperature reflects the average kinetic energy of the molecules in a system, not the energy of any single molecule.
This distinction is critical. A single, extremely fast-moving molecule doesn't define the temperature of the entire substance.
Thermal Energy vs. Temperature: A Crucial Contrast
While temperature reflects the average kinetic energy, thermal energy represents the total energy of all the molecules within a substance. Think of thermal energy as the sum total of all the individual energies.
A large swimming pool at a cool temperature can possess more thermal energy than a hot cup of coffee because the pool contains far more molecules.
This is why the pool can transfer more energy overall, even if the coffee has a higher temperature.
Temperature provides a snapshot of the average molecular motion, while thermal energy describes the total energy reservoir.
Absolute Zero: The Ultimate Standstill
Now, let's consider the theoretical extreme: Absolute Zero. This is the point at which all molecular motion ceases, at least according to classical physics.
It's the ultimate standstill, a state where molecules have minimal kinetic energy. On the Kelvin scale, absolute zero is defined as 0 K, equivalent to -273.15 °C or -459.67 °F.
Reaching absolute zero is practically impossible, as even at extremely low temperatures, quantum mechanical effects still cause some residual motion. However, it serves as a fundamental reference point in thermodynamics.
Temperature Scales: Fahrenheit, Celsius, and Kelvin
To quantify temperature, we use various scales. The most common are Fahrenheit, Celsius, and Kelvin, each with its own history and practical applications.
A Brief History and Uses
-
Fahrenheit: Developed by Daniel Gabriel Fahrenheit, it's primarily used in the United States. Water freezes at 32 °F and boils at 212 °F.
-
Celsius: Also known as centigrade, it's based on the properties of water, with 0 °C as the freezing point and 100 °C as the boiling point. It’s widely used in scientific contexts and most countries worldwide.
-
Kelvin: This is the absolute temperature scale, with its zero point at absolute zero. It's the preferred scale in scientific and engineering applications because it directly relates to molecular kinetic energy.
Converting Between Scales
Converting between these scales is essential for scientific accuracy and understanding. Here are the key formulas:
-
Celsius to Fahrenheit: °F = (°C × 9/5) + 32
-
Fahrenheit to Celsius: °C = (°F − 32) × 5/9
-
Celsius to Kelvin: K = °C + 273.15
-
Kelvin to Celsius: °C = K - 273.15
Understanding these temperature scales and their conversions allows us to accurately measure and compare temperatures across different contexts, highlighting temperature's important role in scientific investigations and everyday life.
Temperature, therefore, gives us an indication of the average molecular activity within a system. But it doesn't tell us how much energy is available for transfer. That's where the concept of heat comes into play. Heat and temperature are inextricably linked, but they represent different aspects of energy. Understanding heat requires shifting our focus from the state of a substance (temperature) to the process of energy transfer.
Heat: The Energy in Transit
Heat, unlike temperature, isn't a property possessed by an object. Instead, heat is the transfer of thermal energy.
It's energy in motion, flowing from one object or system to another due to a temperature difference.
Think of it like this: temperature is the level of water in a tank, while heat is the flow of water between tanks of different levels.
Without a temperature difference, there can be no heat transfer. Objects may contain thermal energy, but this energy only becomes "heat" when it's being exchanged.
Defining Heat: The Engine of Change
Formally, heat is defined as the transfer of thermal energy across the boundary of a system due to a temperature difference. This transfer always occurs from a region of higher temperature to a region of lower temperature, obeying the second law of thermodynamics.
This is a crucial point: heat is energy in transit, constantly seeking equilibrium.
The greater the temperature difference, the faster the rate of heat transfer. This drive towards equilibrium is what powers countless natural and technological processes.
Specific Heat Capacity: A Material's Resistance to Temperature Change
Not all materials respond to heat transfer in the same way. Some materials heat up quickly with only a small amount of energy input, while others require much more energy to achieve the same temperature change.
This property is known as specific heat capacity.
Specific heat capacity is defined as the amount of heat required to raise the temperature of one gram of a substance by one degree Celsius (or one Kelvin).
Water, for example, has a very high specific heat capacity. This means it takes a significant amount of energy to raise its temperature. This is why oceans and large bodies of water moderate temperatures, preventing drastic swings between day and night.
Metals, on the other hand, generally have low specific heat capacities, heating up and cooling down quickly.
Understanding specific heat capacity is crucial in many applications, from designing efficient cooling systems to choosing the right materials for cookware.
Heat and Thermodynamics: Governing the Flow of Energy
The relationship between heat and thermodynamics is fundamental. Thermodynamics is the science that deals with the relationships between heat, work, and energy.
The first law of thermodynamics states that energy is conserved. In other words, energy cannot be created or destroyed, only transferred or converted from one form to another.
Heat plays a central role in this law, as it represents one of the primary mechanisms for energy transfer.
The second law of thermodynamics introduces the concept of entropy, which is a measure of disorder in a system. This law dictates that the total entropy of an isolated system can only increase over time.
Heat transfer invariably increases entropy, as energy disperses and becomes less available for doing work. Therefore, heat is not simply an isolated phenomenon. It is governed by—and illustrates—the fundamental laws of thermodynamics.
The Mechanisms of Heat Transfer: Conduction, Convection, and Radiation
Heat can be transferred in three primary ways: conduction, convection, and radiation. Each mechanism relies on different physical principles and is dominant in different situations.
Conduction: The Touch of Heat
Conduction is the transfer of heat through direct contact between objects or substances. It occurs when faster-moving molecules in a warmer object collide with slower-moving molecules in a cooler object, transferring some of their kinetic energy.
This process continues until thermal equilibrium is reached, and both objects are at the same temperature. Conduction is most effective in solids, where molecules are closely packed together. Metals are particularly good conductors of heat due to the presence of free electrons, which can easily transport energy.
Convection: Riding the Currents
Convection is the transfer of heat through the movement of fluids (liquids or gases).
When a fluid is heated, it expands and becomes less dense, causing it to rise. Cooler, denser fluid then sinks to take its place, creating circulating currents.
These currents carry thermal energy from one location to another. Convection is responsible for weather patterns, ocean currents, and the operation of many heating and cooling systems.
Radiation: The Silent Traveler
Radiation is the transfer of heat through electromagnetic waves. Unlike conduction and convection, radiation does not require a medium to travel through; it can occur even in a vacuum.
All objects emit electromagnetic radiation, with the amount and type of radiation depending on their temperature. When this radiation strikes another object, some of it is absorbed, transferring energy and increasing the object's temperature.
The sun's energy reaches Earth through radiation, and this is how a fire warms you even when you are not touching the flames or standing in the rising hot air.
Heat vs. Temperature: Key Distinctions Unveiled
Having explored temperature as a measure of molecular motion and heat as the energy transfer arising from temperature differences, it's time to directly compare these two fundamental concepts. Untangling their distinctions is essential for a clear understanding of thermal phenomena. While inextricably linked, heat and temperature represent fundamentally different aspects of energy.
Temperature: A Measure of Molecular Agitation
Temperature, at its core, is a measure of the average kinetic energy of the molecules within a substance. Think of it as an indicator of how vigorously these molecules are moving and colliding. A higher temperature signifies greater molecular motion.
It's crucial to remember that temperature doesn't tell us about the total energy content of a substance. A vast iceberg, despite its frigid temperature, possesses a tremendous amount of thermal energy due to its sheer size.
Heat: Energy in Transit
In contrast to temperature's state-based measurement, heat describes the transfer of thermal energy. It exists solely when energy is moving from one object or system to another because of a temperature difference.
This transfer always occurs from a region of higher temperature to a region of lower temperature. Once equilibrium is reached, and the temperature difference vanishes, the transfer of energy as heat ceases.
State Variable vs. Process Variable: A Critical Difference
Perhaps the most significant distinction lies in their classification as thermodynamic variables. Temperature is a state variable. This means its value depends only on the current state of the system, irrespective of how that state was achieved.
Heat, however, is a process variable. It describes the path by which energy is transferred. The amount of heat involved depends entirely on the process, not simply the initial and final states.
Everyday Examples: Illustrating the Divide
To solidify this understanding, let's examine a few real-world examples:
-
Heating Water: When you heat water on a stove, you're adding energy in the form of heat. As the water absorbs this energy, its molecules move faster, and the temperature rises. However, the amount of heat required to raise the water's temperature depends on factors like the volume of water and the efficiency of the heat source.
-
Ice Melting: At 0°C, ice absorbs heat to transition into water, without a change in temperature. The added heat isn't increasing kinetic energy (temperature). Instead, it's breaking the bonds holding the ice molecules in a solid structure. Once all ice is melted, adding more heat will increase the water's temperature.
-
Touching Metal and Wood: On a cold day, metal feels colder than wood, even if both are at the same temperature. This is because metal is a much better conductor of heat. It quickly draws heat away from your hand, making it feel colder. Wood, being a poor conductor, doesn't draw heat away as rapidly. The temperature is the same, but the rate of heat transfer differs.
These examples highlight that while temperature provides a snapshot of molecular activity, heat describes the dynamic process of energy exchange. Grasping this distinction unlocks a deeper comprehension of the thermal world around us.
Video: Heat and Temperature: The Shocking Difference You Didn't Know
FAQs: Heat and Temperature Demystified
Hopefully, the article shed light on the distinction between heat and temperature. Here are some common questions that often arise when exploring this topic.
How can something have a high temperature but low heat?
Temperature measures the average kinetic energy of molecules. A small spark from a lighter can have a very high temperature because its molecules are moving incredibly fast, but because there are so few molecules involved, the total amount of heat (total kinetic energy) is quite low.
So, heat is just the total energy of everything moving?
Essentially, yes. Heat is the total kinetic energy of all the molecules within a substance. The more they jiggle and bounce around, the greater the amount of heat contained within that substance. This contrasts with temperature, which, again, is just the average.
If I add heat to something, will its temperature always increase?
Not necessarily. If the substance is undergoing a phase change (like melting ice or boiling water), the added heat will be used to break the intermolecular bonds, changing its state. During this process, the temperature will remain constant until the phase change is complete.
Why is understanding the difference between heat and temperature important?
Understanding the difference is crucial for various applications, from designing efficient engines to accurately predicting weather patterns. It prevents misconceptions that can lead to errors in scientific reasoning and practical applications involving energy transfer and thermodynamic processes. Appreciating the subtle yet crucial difference between heat and temperature enhances our ability to engage with and comprehend the physical world.